Enzyme Action Lecture 4 PDF
Document Details
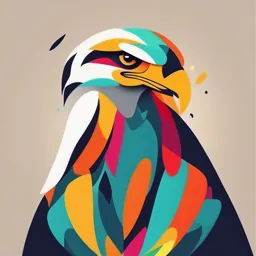
Uploaded by BetterRational
Cairo University Medicine
Tags
Summary
This lecture provides an overview of enzyme action, covering the lock-and-key model, induced fit model, transition state theory, and factors affecting the rate of enzymatic reactions. It also touches upon different inhibition types and relevant applications.
Full Transcript
Mechanism of Enzyme Action Substrate in the enzyme active site Enzymes are usually proteins – each has a very specific shape or conformation. Within this large molecule is a region called an active site, which has pr...
Mechanism of Enzyme Action Substrate in the enzyme active site Enzymes are usually proteins – each has a very specific shape or conformation. Within this large molecule is a region called an active site, which has properties allowing it to bind tightly to the substrate molecule(s). The enzyme - substrate interactions can be explained by the following theories: 1) Lock and key model 2) Induced fit model 3) Transition state theory The lock and key model proposed by Fischer in 1894, In this model the active site of the enzyme is complementary in shape to that of the substrate. The substrate is held in such a way that its conversion to the reaction products is more favorable. It was thought that the substrate exactly fitted into the active site of the enzyme molecule like a key fitting into a lock. "lock" refers to enzyme and "key" refers to its complementary substrate. 1- Lock and Catalysis therefore only takes when a substrate with the key model right shape complements the active site shape. Lock and key model do not explain the stability of the transition state for it would require more energy to reach the transition state complex. To explain this concept Koshland in 1958, first proposed the induced-fit model, this suggests that the enzyme active site is conformationally fluid. Enzyme itself usually undergoes a change in conformation when the substrate binds, induced by multiple weak interactions and hydrophobic characteristics on the enzyme surface mold into a precise formation. i.e. This model proposes that the initial interaction between enzyme and 2- Induced substrate is relatively weak, but that these weak interactions rapidly induce conformational changes in the enzyme that fit model strengthen binding. According to this theory when an enzyme catalysis, the enzyme binds more strongly to its "transition state complex rather than its ground state reactants." This indicates, the transition state is more stable. A simple enzymatic reaction can be written as, E +S ES EP E+ P Where E, S, and P represent the enzyme, substrate, and product respectively; ES and EP are transient complexes of the enzyme with the substrate and with the product respectively, i.e. enzyme-catalyzed reactions have three basic steps: 1. binding of substrate: E + S ES complex 3- Transition 2. conversion of bound substrate to bound product: ES 3. release of product : EP E+P EP state theory The transition state is not a chemical species with any significant stability and should not be confused with a reaction intermediate (such as ES or EP). It is simply a fleeting molecular moment in which events such as bond breakage, bond formation, and charge development have proceeded to the precise point at which decay to either substrate or product is equally likely. The difference between the energy levels of the ground state and the transition state is the activation energy; the rate of a reaction reflects this activation energy: higher activation energy corresponds to slower reaction. Reaction rates can be increased by raising the temperature, thereby increasing the number of molecules with sufficient energy to overcome the energy barrier. Alternatively, the activation energy can be lowered by adding a catalyst. 1.Temperature. Factors affecting the 2.pH. rate of 3.Enzyme concentration. enzymatic 4.Substrate concentration. reaction 5.Concentration of end-products. 6.Inhibition. 1- Temperature If temperature is reduced to near or below freezing point, enzymes are inactivated only, not denatured. They will regain their catalytic influence when higher temperature is restored. As the temperature increase, the kinetic energy of the substrate and enzyme molecules increases and so they move faster. The faster these molecules move, the more often they collide with one another and the greater the rate of reaction. As the temperature increases further, the more the atoms which make up the enzyme molecules vibrate. This breaks the hydrogen bonds and other forces which hold the molecules on their precise shape. So, the shape of the active site altered and no longer fit the substrate. The enzyme is said to be denatured and loses its catalytic activity forever. 2- pH Changes in pH alter the ionic charge of the acidic and basic groups that help to maintain the specific shape of the enzyme i.e. break the hydrogen bonds. The pH change leads to an alternation in enzyme shape, particularly at its active site. i.e. enzyme denatured. Under constant temperature and pressure, every enzyme has a narrow pH range within which it will function effectively. As the pH of reacting medium increases above or falls below the optimum pH, enzyme activity decreases. At either extreme of pH, the enzyme is denatured. Different enzymes may have different optimum pH. 3- Enzyme concentration The active site of an enzyme may be used again and again, thus enzymes work efficiently at very low concentrations. One enzyme molecule can deal with only one substrate molecule at instant of time. Thus, the more the enzyme molecules present at the same time, the larger the number of substrate molecules can be converted to products if there is an unlimited supply of substrate. 4- Substrate concentration At low substrate concentrations, the active sites of the enzyme molecules are not all used because there are not enough substrate molecules to occupy them all. As substrate concentration is increased, more and more sites come into use. When over the saturation point, a point where all active sites are being occupied by substrates, increasing the substrate concentration cannot increase the rate of reaction. This is because any extra substrate must wait for the E-S complex dissociated into products and free enzyme. Therefore, at high substrate levels, both enzyme concentration and the dissociation time are the limiting factors. 5- Concentration of end-products As the end-products accumulate, pH of the medium may change so that the rate of reaction may also be changed. In some enzymatic reactions, the enzyme itself combines with one of the end products i.e., those end - products will inhibit the activity of the enzymes - negative feedback. this may be due to the shape of the product like that of the substrate and therefore can fit into the active sites of enzymes. So, rate of enzymatic reaction decreases with the accumulation of the end-products. 6- Inhibition A variety of small molecules exist which can reduce the rate of an enzyme-controlled reaction. They are called enzyme inhibitors. Inhibition may be reversible or irreversible. I- Reversible inhibition The inhibitor can be easily removed from the enzyme and cause no permanent damage. It can be competitive, non-competitive or uncompetitive inhibitor. First, we should know the assumptions are made in deriving the Michaelis- Menten rate equation and their relations to the inhibition types. The following assumptions are made in deriving the Michaelis- Menten rate equation 1. Relative concentrations of E and S The concentration of substrate [S] is much greater than the concentration of enzyme [E], so that the percentage of total substrate bound by the enzyme at any one time is small. 2. Steady-state assumption [ES] does not change with time (the steady-state assumption), that is, the rate of formation of ES is equal to that of the breakdown of ES (to E + S and to E + P). 3. Initial velocity Initial reaction velocities (Vo) are used in the analysis of enzyme reactions. This means that the rate of the reaction is measured as soon as enzyme and substrate are mixed. At that time, the concentration of product is very small and, therefore, the rate of the back reaction from P to S can be ignored Michaelis–Menten Kinetic Theory of Enzyme Action Michaelis–Menten model accounts for the kinetic properties of some enzymes. It helps to describe many enzymatic reactions under the following assumptions: ►The reaction has only one substrate. ►The substrate concentration is much higher than that of the enzyme. ►Only the initial reaction velocity (Vo) is measured.. Why is it necessary to consider only the initial reaction rate? At the beginning of the reaction the substrate alone, is present and the unoccupied active- sites of the enzyme molecule are free to bind them. Therefore, the substrate is rapidly converted to the product. As the product concentration builds up and the substrate concentration falls, the reaction keeps getting slower and eventually stops at equilibrium. Since it is impossible to consider this “changing” reaction rate, only the initial reaction-rate (Vo) is taken into account. The relation between Vo and the substrate concentration [S] is given by Michaelis–Menten equation: The equation predicates a hyperbolic curve of Vo against [S]. Vo is measured at different substrate concentrations by incubating different amounts of the substrate with enzyme. The rate of disappearance of the substrate or the rate of appearance of the product during the first few minutes of the reaction only is taken into account. Vmax is the maximum velocity that can be reached by increasing the substrate concentration. For any set of conditions, it is a constant. It indicates a saturation state at which most enzyme active sites are occupied by the substrate molecules, and the reaction rate is no longer limited by substrate availability. [S] is the substrate concentration at the initial phase of the reaction. This changes as the reaction proceeds, so the experimental data are taken, in the first few minutes of the reaction. Km is a special rate constant called the Michaelis constant. It is defined as the substrate concentration at which the reaction rate is half-maximal. At this instance, the enzyme is half-saturated with its substrate. Km has a characteristic value for a given enzyme substrate pair. In case of the enzyme which acts on more than one substrate, the value of Km is different for each of them. e.g. Km of the enzyme hexokinase for fructose is 1.5 mM, whereas for glucose it is 0.05 mM. Km reflects binding affinity The Michaelis–Menten constant reflects the binding affinity of the enzyme for its substrate. If affinity is more, less substrate is required to saturate the enzyme, so that Vmax is reached at a relatively low substrate concentration. Consequently, the substrate concentration corresponding to the half Vmax (i.e. Km) is relatively low. For this reason, high enzyme-substrate affinity implies a low Km value, and conversely, low-affinity implies high Km. e.g. Higher Km of glucose for glucokinase (10 mM) than that for hexokinase (0.05 mM) indicates that hexokinase binds glucose with higher affinity than glucokinase. Lineweaver–Burk plot The slope of the Michaelis–Menten curve is gradual and upwards giving it a hyperbolic shape. From this shape, it is not possible to estimate the exact value of Vmax. However, if reciprocal of these parameters: 1/ Vo and 1/[S] are plotted against each other, a straight line is obtained. From this line it is possible to determine the exact value Vmax. This plot known as double reciprocal plot or the Lineweaver–Burk plot, can be used to determine the mechanism of action of enzyme inhibitors, discussed latter. The equation describing the double reciprocal plot is: a) Competitive inhibitors They compete with the substrates for the active sites of enzyme molecules as they are structurally like the substrate. while remain in the active sites, they prevent access of the true substrate. if the substrate concentration is increased, the rate of reaction will be increased. For example: i) Malonic acid --- It compete with succinate for the active sites of succinic dehydrogenase, an important enzyme in the Krebs Cycle. ii) Sulphonamides --- during the Second World War, sulphonamides were used extensively to prevent the spread of microbial infection. Sulphonamides are similar in structure to paraaminobenzoate (PAB), a substance essential to the growth of many pathogenic bacteria. The bacteria require PAB to produce folic acid, an important enzyme cofactor. Sulphonamides acts by interfering with the synthesis of folic acid from PAB. E + S ES E + PIn competitive inhibition, + the inhibitor binds only I to the free enzyme, not to the ES complex. EI Competitive Inhibition Mechanism The inhibitor exhibits is a close structural resemblance with the actual substrate. It impairs substrate-binding to the enzyme, because it occupies the active site of the enzyme making it unavailable for the substrate. Occupancy of the enzyme active site by the inhibitor yields EI complex. Since the formation of (EI) depends upon the concentration of the inhibitor (just as the formation of ES is dependent on the substrate concentration), The inhibition is strictly dependent upon the relative concentrations of the inhibitor and the substrate at fixed concentration of the enzyme. Effect on enzyme kinetics: The competitive inhibitor The Km shows an apparent increase in the presence of the inhibitor. This reflects decreased affinity of the substrate with the enzyme active site. The decreased affinity is because the substrate must now compete with the inhibitor for the active site. This is depicted in a double reciprocal plot as a shift in the x- intercept (–1/Km) and in the slope of the line (Km/Vmax). Vmax (the measure of fastest possible rate) remains unchanged because the binding of inhibitor is reversible; and at infinite concentration of the substrate (obtained by extrapolation) the inhibitor can be completely displaced from the enzyme active site. b) Non-competitive inhibitors This type of inhibitor has no real structural similarity to the substrate and forms an enzyme-inhibitor complex at a point on the enzyme other than its active site. They alter the shape of the enzyme in such a way that the active site can no longer properly accommodate the substrate. increase the substrate concentration will not therefore reduce the effect of the inhibitor as they are not competing the same site, i.e. increasing the substrate concentration does not reverse the inhibition. but increase the inhibitor concentration will decrease the reaction rate. For example: Cyanide --- attaches itself to copper prosthetic group of cytochrome oxidase, thereby inhibiting respiration. E + S ES E + P The non-competitive inhibitor may + + bind the free enzymes as well as the I I enzyme substrate complex, forming binary (EI) and ternary (ESI) complexes, respectively. Both are EI+S ESI catalytically inactive and are therefore dead-end complexes. The inhibitor binds equally well to free enzyme and the ES complex, so it doesn’t alter apparent affinity of the enzyme for the substrate. Effect on enzyme kinetics: The non-competitive inhibitor Non-competitive inhibitor does not block the active site of the enzyme. Therefore, the Km (indicating enzyme-substrate affinity) remains unaffected. Increasing the concentration of the substrate does not affect binding of the inhibitor to the enzyme. This is because the shape of the enzyme is different when the inhibitor is bound to it, and hence it does not matter how much substrate is present; the enzyme will always be less effective due to this shape change. This is reflected in decreased Vmax, and is seen graphically on the double reciprocal plot where both the slope and the y-intercept are changed. c) Uncompetitive Inhibitors The inhibitor binds with only the ES form of the enzyme to form the enzyme-substrate-inhibitor (ESI) complex. E + S ES E + P Binding may occur at a site distant from the + active site, or at a site overlapping with the active I site. In either case, the result is lowering the catalytic efficiency of the enzyme and increasing its ESI apparent binding affinity for the substrate. These result in changes in Km and Vmax (both decreases). uncompetitive inhibitors don’t bind to the free enzyme, so there is no EI complex in the reaction mechanism As a result, in the double reciprocal plot a line parallel to that of the uninhibited enzyme (slope remains unchanged), but with altered intercepts on both, the x and the y axis. The binding of an inhibitor to a site other than the active site may not only change the shape of the enzyme, but also affect the binding of the substrate at the active site, so that Km as also Vmax is altered. Finally we conclude that II- Irreversible inhibition The inhibitor combines with the enzyme permanently and so the enzyme unable to carry out its catalytic function forever. Heavy metal ions such as mercury and silver cause disulphide bonds to break. These bonds E + S ES E + P help to maintain the shape of the enzyme molecule. Once broken the + enzyme molecule’s structure becomes irreversibly altered with the I permanent loss of its catalytic properties. EI The inhibitor has no structural resemblance with the substrate and may bind to a site other than the substrate-binding site. This type of inhibition resembles the non-competitive inhibition, except that covalent bonds are formed in this case. i.e. the inhibitor reacts near the active site of the enzyme with covalent modification of the active site.. The Michaelis-Menten plot for an irreversible Reaction Rate inhibitor looks like noncompetitive inhibition Vmax - Inhibitor Irreversible inhibitors decrease Vmax,app , but Vmax,app leave the apparent Km unchanged. Irreversible 1V + Inhibitor 2 max inhibitors differ from 1V other types of inhibitors 2 max,app because they covalently modify the enzyme. This results in the permanent Km [Substrate] inhibition of the enzyme Km,app activity. Examples of the irreversible inhibition i) Diisopropyl fluorophosphate (DFP) (the nerve gas used in Second World War) forms enzyme - inhibitor complex with the amino acid serine at the active site of enzyme acetylcholinesterase. This enzyme deactivates the chemical transmitter substance acetylcholine so that a normal transmission of nerve impulse can be propagated. If this enzyme is inhibited, paralysis or death will be the end result. ii) Some insecticides (such as parathion) --- have similar effects as the nerve gas (DFP). Therapeutic Applications of Enzyme Inhibitors Several synthetic drugs (and natural compounds) exert pharmacological effects by acting as enzyme inhibitors. This application is most easily appreciated with antiviral, antibacterial, and antitumor drugs that are administered under the condition of limited toxicity. Those drugs that resemble the substrate of an enzyme-catalyzed reaction are competitive inhibitors with respect to that substrate and non-competitive with respect to other substrates. Example for therapeutic Inhibitors Captopril, a commonly used drug in the treatment of hypertension acts by competitively inhibiting the angiotensin converting enzyme (ACE). This enzyme catalyzes the proteolytic cleavage of angiotensin I to angiotensin II; the latter elevates the arterial blood pressure. Captopril and other ACE inhibitors decrease formation of angiotensin II, thereby lowering blood pressure. More examples of clinically useful enzyme inhibitors