Environmental and Societal Considerations PDF
Document Details
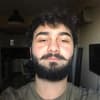
Uploaded by LovableSonnet
Dokuz Eylül University
Tags
Summary
This document discusses the environmental and societal considerations related to the materials cycle. It details the various stages involved in the use and disposal of materials from extraction to manufacturing to application. The document emphasizes the importance of considering environmental impacts at each stage and the benefits of material recycling. It also highlights the connection between environmental impact and economic factors.
Full Transcript
**MME1107 TECHNICAL ENGLISH I** **ENVIRONMENTAL AND SOCIETAL CONSIDERATIONS** Materials play a crucial role in the technology-economy-environment scheme. A material that is utilized in some end product and then discarded passes through several stages or phases; these stages are represented in Figu...
**MME1107 TECHNICAL ENGLISH I** **ENVIRONMENTAL AND SOCIETAL CONSIDERATIONS** Materials play a crucial role in the technology-economy-environment scheme. A material that is utilized in some end product and then discarded passes through several stages or phases; these stages are represented in Figure 23.1, which is sometimes termed the "total materials cycle" or just "materials cycle," and represents the "cradle-to-grave" life circuit of a material. Beginning on the far left side of Figure 23.1, raw materials are extracted from their natural earthly habitats by mining, drilling, harvesting, and so on. These raw materials are then purified, refined, and converted into bulk forms such as metals, cements, petroleum, rubber, and fibers. Further synthesis and processing results in products that are what may be termed "engineered materials"; examples include metal alloys, ceramic powders, glass, plastics, composites, semiconductors, and elastomers. Next, these engineered materials are further shaped, treated, and assembled into products, devices, and appliances that are ready for the consumer---this constitutes the "product design, manufacture, assembly" stage of Figure 23.1. The consumer purchases these products and uses them (the "applications" stage) until they wear out or become obsolete, and are discarded. At this time the product constituents may either be recycled/reused (whereby they reenter the materials cycle) or disposed of as waste, normally being either incinerated or dumped as solid waste in municipal land-fills---as such, they return to the earth and complete the materials cycle. It has been estimated that worldwide, on the order of 15 billion tons of raw materials are extracted from the earth every year; some of these are renewable and some are not. Over time, it is becoming more apparent that the earth is virtually a closed system relative to its constituent materials and that its resources are finite. In addition, as our societies mature and populations increase, the available resources become scarcer, and greater attention must be paid to more effective utilization of these resources relative to this materials cycle. Furthermore, energy must be supplied at each cycle stage; in the United States it has been estimated that approximately one-half of the energy consumed by manufacturing industries goes to produce and fabricate materials. Energy is a resource that, to some degree, is limited in supply, and measures must be taken to conserve and more effectively utilize it in the production, application, and disposal of materials. Finally, there are interactions with and impacts on the natural environment at all stages of the materials cycle. The condition of the earth's atmosphere, water, and land depends to a large extent on how carefully we traverse this materials cycle. Some ecological damage and landscape spoilage undoubtedly result during the extraction of raw materials phase. Pollutants may be generated that are expelled into the air and water during the synthesis and processing stage; in addition, any toxic chemicals that are produced need to be disposed of or discarded. The final product, device, or appliance should be designed so that during its lifetime, any impact on the environment is minimal; furthermore, that at the end of its life, provision is made for recycling of its component materials, or at least for their disposal with little ecological degradation (i.e., it should be biodegradable). Recycling of used products rather than disposing of them as waste is a desirable approach for several reasons. First of all, using recycled material obviates the need to extract raw materials from the earth, and thus conserves natural resources and eliminates any associated ecological impact from the extraction phase. Second, energy requirements for the refinement and processing of recycled materials are normally less than for their natural counterparts; for example, approximately 28 times as much energy is required to refine natural aluminum ores as to recycle aluminum beverage can scrap. Finally, there is no need to dispose of recycled materials. Thus, this materials cycle (Figure 23.1) is really a system that involves interactions and exchanges among materials, energy, and the environment. In many countries, environmental problems and issues are being addressed by the establishment of standards that are mandated by governmental regulatory agencies. Furthermore, from an industrial perspective, it becomes incumbent for engineers to propose viable solutions to existing and potential environmental concerns. Correcting any environmental problems associated with manufacturing will influence product price. That is, manufacturing cost is normally greater for a "green" (or "environmentally friendly") product than for its equivalent that is produced under conditions wherein environmental issues are minimized. Thus, a company must confront the dilemma of this potential economic-environmental trade-off and then decide the relative importance of economics and of environmental impact. One approach that is being implemented by industry to improve the environmental performance of products is termed life cycle analysis/assessment. With this approach to product design, consideration is given to the cradle-to-grave environmental assessment of the product, from material extraction to product manufacture to product use, and, finally, to recycling and disposal; sometimes this approach is also labeled "green design." One important phase of this approach is to quantify the various inputs (i.e., materials and energy) and outputs (i.e., wastes) for each phase of the life cycle; this is represented schematically in Figure 23.2. In addition, an assessment is conducted relative to the impact on both global and local environments in terms of the effects on the ecology, human health, and resource reserves. **RECYCLING ISSUES IN MATERIALS SCIENCE AND ENGINEERING** Important stages in the materials cycle where materials science and engineering plays a significant role are recycling and disposal. The issues of recyclability and disposability are important when new materials are being designed and synthesized. Furthermore, during the materials selection process, the ultimate disposition of the materials employed should be an important criterion. Let us conclude this section by briefly discussing several of these recyclability/disposability issues. From an environmental perspective, the ideal material should be either totally recyclable or completely biodegradable. Recyclable means that a material, after having completed its life cycle in one component, could be reprocessed, could reenter the materials cycle, and could be reused in another component---a process that could be repeated an indefinite number of times. By completely biodegradable, we mean that, by interactions with the environment (natural chemicals, microorganisms, oxygen, heat, sunlight, etc.), the material deteriorates and returns to virtually the same state in which it existed prior to the initial processing. Engineering materials exhibit varying degrees of recyclability and biodegradability. **Metals** Most metal alloys (i.e., Fe, Cu), to one degree or another experience corrosion and are also biodegradable. However, some metals (i.e., Hg, Pb) are toxic and, when land-filled, may present health hazards. Furthermore, alloys of most metals are recyclable; on the other hand it is not feasible to recycle all alloys of every metal. In addition, the quality of alloys that are recycled tends to diminish with each cycle. Product designs should allow for the dismantling of components composed of different alloys. Another of the problems of recycling involves separation of various alloys types (i.e., aluminum from ferrous alloys) after dismantling and shredding; in this regard, some rather ingenious separation techniques have been devised (i.e., magnetic and gravity). Joining of dissimilar alloys presents contamination problems; for example, if two similar alloys are to be joined, welding is preferred over bolting or riveting. Coatings (paints, anodized layers, claddings, etc.) may also act as contaminants, and render the material nonrecyclable. Aluminum alloys are very corrosion resistant and, therefore, nonbiodegradable. Fortunately, however, they may be recycled; in fact, aluminum is the most important recyclable nonferrous metal. Since aluminum is not easily corroded, it may be totally reclaimed. A low ratio of energy is required to refine recycled aluminum relative to its primary production. In addition, there are a large number of commercially available alloys that have been designed to accommodate impurity contamination. The primary sources of recycled aluminum are used beverage cans and scrapped automobiles. **Glass** The one ceramic material that is consumed by the general public in the greatest quantities is glass, in the form of containers. Glass is a relatively inert material, and, as such, it does not decompose; thus, it is not biodegradable. A significant proportion of municipal land-fills consists of waste glass; so also does incinerator residue. In addition, there is not a significant economic driving force for recycling glass. Its basic raw materials (sand, soda ash, and limestone) are inexpensive and readily available. Furthermore, salvaged glass (also called "cullet") must be sorted by color (clear, amber, and green), by type (plate versus container), and by composition (lime, lead, and borosilicate \[or Pyrex\]); these sorting procedures are time-consuming and expensive. Therefore, scrap glass has a low market value, which diminishes its recyclability. Advantages of utilizing recycled glass include more rapid and increased production rates and a reduction in pollutant emissions. **Plastics and Rubber** One of the reasons that synthetic polymers (including rubber) are so popular as engineering materials lies with their chemical and biological inertness. On the down side, this characteristic is really a liability when it comes to waste disposal. Polymers are not biodegradable, and, as such, they constitute a significant land-fill component; major sources of waste are from packaging, junk automobiles, automobile tires, and domestic durables. Biodegradable polymers have been synthesized, but they are relatively expensive to produce. On the other hand, since some polymers are combustible and do not yield appreciable toxic or polluting emissions, they may be disposed of by incineration. Thermoplastic polymers, specifically poly(ethylene terephthalate), polyethylene, and polypropylene, are those most amenable to reclamation and recycling, since they may be reformed upon heating. Sorting by type and color is necessary. In some countries, type sorting of packaging materials is facilitated using a number identification code; for example, a "1" denotes high-density polyethylene (HDPE). Table 23.1 presents these recycling code numbers and their associated materials. Also included in the table are uses of virgin and recycled materials. Plastics recycling is complicated by the presence of fillers that were added to modify the original properties. The recycled plastic is less costly than the original material, and quality and appearance are generally degraded with each recycle. Typical applications for recycled plastics include shoe soles, tool handles, and industrial products such as pallets. The recycling of thermoset resins is much more difficult since these materials are not easily remolded or reshaped due to their crosslinked or network structures. Some thermosets are ground up and added to the virgin molding material prior to processing; as such, they are recycled as filler materials. Rubber materials present some disposal and recycling challenges. When vulcanized, they are thermoset materials, which makes chemical recycling difficult. In addition, they may also contain a variety of fillers. The major source of rubber scrap in the United States is discarded automobile tires, which are highly nonbiodegradable. Scrap tires have been utilized as a fuel for some industrial applications (i.e., cement plants), but yield dirty emissions. Recycled rubber tires that have been split and reshaped are used in a variety of applications such as automotive bumper guards, mud flaps, door mats, and conveyor rollers; and, of course, used tires may also be recapped. In addition, rubber tires may be ground into small chunks that are then recombined into the desired shape using some type of adhesive; the resulting material may be used in a number of nondemanding applications such as place mats and rubber toys. **Composite Materials** Composites are inherently difficult to recycle because they are multiphase in nature. The two or more phases/materials that constitute the composite are normally intermixed on a very fine scale and trying to separate them complicates the recycling process. However, some techniques have been developed, with modest success, for recycling polymer--matrix composites. Recycling technologies will differ only slightly for thermoset--matrix and thermoplastic--matrix composite materials. The first step in recycling both thermoset-- and thermoplastic--matrix composites is shredding/grinding, wherein the components are reduced in size to relatively small particles. In some instances, these ground particles are used as filler materials that are blended with a polymer (and perhaps other fillers) before fabrication (usually using some type of molding technique) into postconsumer products. Other recycling processes allow for separating of the fibers and/or matrix materials. With some techniques the matrix is volatilized; with others it is recovered as a monomer. Of course, the recovered fibers have short lengths, as a result of the shredding/grinding process. In addition, fibers will experience a reduction of mechanical strength, the degree of which will depend on the specific recovery process as well as fiber type. **[After reading this text, make sure that you can answer the following questions by yourself:]** **Questions** 1. What are the stages of materials cycle? 2. What are the two major inputs of this cycle? 3. What is the problem in terms of raw materials? 4. What is the problem in terms of energy? 5. What are the negative impacts of this cycle on the environment? 6. What are the two approaches to reduce the environmental impact? 7. Why is it better to recycle used products than disposing them as waste? 8. What is "life cycle analysis/assessment" of a product? 9. What do you understand from "green design"? 10. What is an "ideal material" from an environmental perspective? 11. What is "recyclable material"? 12. What is "biodegradable material"? 13. What is the most important recyclable nonferrous metal, why? 14. Why recycling glass is not preferred? 15. What can be done with the polymer wastes? 16. Why is it difficult to recycle composites?