Encapsulation Overview PDF
Document Details
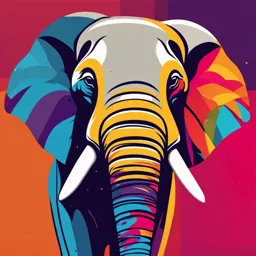
Uploaded by NoteworthyOpal3387
University of Home Economics
Munmaya Mishra
Tags
Summary
This document is a handbook on encapsulation and controlled release, focusing on various techniques for microencapsulation. It details definitions, justifications, classifications, specific technologies, and criteria for selecting appropriate techniques, including processes like annular jet, centrifugal extrusion, and spray drying. The handbook is targeted at students and researchers in fields such as chemistry, food science, and pharmaceutics.
Full Transcript
# Handbook of Encapsulation and Controlled Release ## Edited by Munmaya Mishra # 1 Overview of Encapsulation and Controlled Release ### Munmaya K. Mishra ### CONTENTS - 1.1 Definitions. - 1.2 Justification for Encapsulation - 1.3 Classification. - 1.4 Techniques of Microencapsulation - 1.5 C...
# Handbook of Encapsulation and Controlled Release ## Edited by Munmaya Mishra # 1 Overview of Encapsulation and Controlled Release ### Munmaya K. Mishra ### CONTENTS - 1.1 Definitions. - 1.2 Justification for Encapsulation - 1.3 Classification. - 1.4 Techniques of Microencapsulation - 1.5 Criteria for Selecting Encapsulation Technology - 1.6 Annular Jet (Vibrational Nozzle). - 1.7 Centrifugal Extrusion - 1.8 Centrifugal Suspension Separation. - 1.9 Cocrystallization... - 1.10 Coacervation.. - 1.11 Emulsification/Emulsion Polymerization. - 1.12 Fluid-Bed Coating - 1.13 Interfacial Polymerization - 1.14 Inclusion Complexation - 1.15 Ionic Gelation (Hydrogel Microspheres) - 1.16 Liposomes. - 1.17 Lyophilization. - 1.18 Melt Extrusion (Melt Injection) - 1.19 Pan Coating. - 1.20 Phase Separation. - 1.21 Spray Drying. - 1.22 Spray Congealing. - 1.23 Spinning Disk (Centrifugal Suspension Separation) - 1.24 Solvent Evaporation - 1.25 Supercritical Fluid Assisted Encapsulation - 1.26 Controlled Release. - 1.27 Release Mechanisms. - 1.28 Release Rates - 1.29 Conclusions.. - References. Encapsulation or commonly referred to as microencapsulation involves the incorporation of actives such as: flavors, drugs, enzymes, cells, or other materials) in small capsules. Capsules offer a means to protect sensitive components, transform liquids into easily handled solid ingredients, and incorporate controlled release attributes such as time-release, targeted-release, or trigger-release mechanisms) into the product formulations. Various techniques are available to design capsules depending on the end use of the final product. A timely and targeted release improves the effective- ness of actives, broadens the application range of ingredients, and ensures optimal dosage, thereby improving the cost effectiveness for the manufacturer. Encapsulation techniques can facilitate prod- uct development through the use of carefully tuned controlled release attributes. ### 1.1 DEFINITIONS Encapsulation is defined as a technology of casing solids, liquids, or gaseous materials in minia- turesealed capsules which are nanometer to micrometer to millimeter range) that can release their contents at controlled rates under specific conditions. This technique depends on the physical and chemical properties of the material to be encapsulated. The microencapsulation technology has been employed in a diverse range of industry such as chemicals, cosmetics, food, pharmaceuticals, printing, etc. The development of early encapsulation technology and preparation of microcapsules dates back to 1950s when Green and coworkers produced microencapsulated dyes by complex coacervation of gelatin and gum Arabic, for the manufacture of carbonless copying paper. The technologies developed for carbonless copy paper have led to the development of various microcapsule products in later years. ### 1.2 JUSTIFICATION FOR ENCAPSULATION The capsule has the ability to preserve a substance in the finely divided state and to release it as needed. The size of the capsules may range from submicrometer to several millimeters in size and have a multitude of different shapes, depending on the materials and methods used to prepare them. The encapsulations/entrapment of active ingredients are done for a variety of reasons: - Protecting the core material from degradation by reducing its reactivity to the outside envi- ronment such as UV light, heat, moisture, air oxidation, chemical attack, acids, bases, etc.). - Reducing/retarding the evaporation or transfer rate of a volatile active ingredient the core material) to the outside environment. - Enhancing the visual aspect and marketing concept of the final encapsulated product. - Modifying the physical characteristics of a material, making it easier to handle e.g., converting liquid into solid form, improving the handling properties of a sticky mate- rial, etc.). - Achieving controlled and/or targeted release of active ingredients. The product can be tailored to either release slowly over time or at a certain point. Improving shelf life by preventing degradative reactions dehydration, oxidation, etc.). - Masking of taste or odors of active ingredient(s). - Handling highly valuable active ingredient the core material can be diluted when only very small amounts are required, yet still achieve a uniform dispersion in the host material). - Mixing incompatible compounds by separating components within a mixture that would otherwise react with one another. - Improved processing of materials texture and less wastage of ingredients, control of hygroscopic attributes, enhance other attributes such as flowability, solubility, and dispers- ibility; dust-free powder). - For safe handling of the toxic materials. ### 1.3 CLASSIFICATION Microcapsules can be classified on the basis of their size or morphology, and they range in size from 1 µm to few millimeters. Some microcapsules whose diameter is in the nanometer range are referred to as nanocapsules to highlight their small size. Particles having diameter between 3 and 8 µm are called microparticles or microspheres or microcapsules. Particles larger than 1000 µm are called macroparticles. The microscopic size of microcapsules provides a huge surface area e.g., the total surface area of 1 µm of hollow microcapsules having a diameter of 0.1 mm has been reported to be about 60 m²) is available for sites of adsorption and desorption, chemical reactions, etc. The morphology of microcapsules depends mainly on the core material, how it is distributed within the system, and the deposition process of the shell. Similarly, the morphology of the internal structure of a microparticle depends largely on the selected shell materials and the microencapsula- tion methods that are employed. The microcapsules may be categorized into several arbitrary and overlapping classifications such as - Mononuclear also known as core-shell) microcapsules contain the shell around the core. This is also called a single-core or monocore capsule. - Polynuclear capsules have many cores enclosed within the shell. This is also called polycore- or multicore-type capsule. - Matrix encapsulation in which the core material is distributed homogeneously within the shell material. In addition to these three basic morphologies, microcapsules can also be mononuclear with multiple shells such as layering of shells), or they may form clusters of microcapsules as noted in Figure 1.1). Matrix encapsulation is the simplest structure, in which the active ingredient core) is much more dispersed within the carrier/shell material either in the form of relatively small droplets or more homogenously distributed/embedded in a continuous matrix of wall material. The active ingredients in the matrix type morphology are also present at the surface unless there is additional coating applied. The composition, mechanism of release, particle size, and final physical form of microcapsules can be changed to suit specific applications. The properties of the shell materials are extremely impor- tant for the stabilization of the core material. Importantly, it must be inert toward active ingredients. - **Figure 1.1** Schematics of types of microcapsules (spherical shaped shown; however, other forms possible). It could be film-forming, pliable, tasteless, stable, nonhygroscopic, no high viscosity, economical, soluble in an aqueous media or solvent, or melting. In addition, the shell material can be flexible, brittle, hard, thin, etc. Microcapsule-based systems increase the life span of actives and control the release of said actives. ### 1.4 TECHNIQUES OF MICROENCAPSULATION In a sense, the process of microencapsulation actually covers three separate processes on a time scale: 1. The first process consists of forming a shell wall around the core material. 2. The second process involves keeping the core materials intact inside the wall material so that it does not release. 3. The third process involves releasing the core material at the right time and at the right rate. ### 1.5 CRITERIA FOR SELECTING ENCAPSULATION TECHNOLOGY The selection of the microencapsulation process is determined by the physical and chemical prop- erties of core and shell/coating materials and the intended application. Various technologies and shell materials have been developed to design microcapsules with wide variety of functionalities. By using selective encapsulation techniques and shell materials, designed microcapsules with con- trolled and/or targeted release of the active encapsulated ingredients by using triggers, such as pH change, mechanical stress, temperature, enzymatic activity, time, osmotic force, etc.) can be obtained. It is not a matter of trial and error for selecting the proper encapsulation technology rather a well- thought process, which includes fulfilling certain requirements. The purpose of encapsulation must be clearly defined before considering the properties desired in encapsulated products. One of the first things to access is the type of benefits such as desired properties, improving product/process, storage stability, etc.) one would like to accomplish through encapsulation technology. Various con- siderations come into play in designing the encapsulation process. The following items need to be considered for designing the encapsulation and selecting the proper encapsulation process: - The physicochemical characteristics of the active and the functionality of the encapsulated ingredients in the final product. - The appropriate processing conditions during the final production of products for the sur- vival of the encapsulated ingredient. - The storage conditions of encapsulatedencapsulated ingredients prior to use. - The storage conditions of the final product containing the ingredients. - The physical properties the particle size, density, and stability requirements) of the encap- sulated ingredient. - The trigger(s) and mechanism(s) for releasing the active ingredient from microcapsules. - The cost constraints if any. Based on the outcome of the evaluation process, the following items need to be selected for the preferred encapsulation process. - The type of coating/shell-wall material(s). The selection of coating material decides the physical and chemical properties of microcapsules/microspheres. The polymer should be capable of forming a film that is cohesive with the core material. - The amount/percentage of core/loading for the microcapsule. The concentration of the active ingredient in the microcapsule. - The type of encapsulation technology/process. - The legal issues, if any, to be considered concerning the technology. - The intellectual property status and freedom to practice/use. - The scale-up possibilities with no quality concerns. ### TABLE 1.1 ### Various Encapsulation Processes - Annular Jet Process - Centrifugal suspension separation - Coacervation and phase separation - Cocrystallization - Controlled precipitation - Dispersion/suspension polymerization - Dripping and jet break up - Electrospraying - Emulsification/emulsion polymerization - Emulsion-solvent removal - Fluidized bed coating technology - Freeze-drying - Garnulation - Hot-melt extrusion process - In situ polymerization - Interfacial cross-linking/reaction - Interfacial polymerization - Ionotropic gelation - Layer-by-Layer Deposition - Matrix encapsulation - Melt-dispersion - Microfluidic encapsulation - Molecular encapsulation - Organogels - Pan coating technology - Phase inversion/precipitation - Polyelectrolyte complexation - Self-assembly - Sol-gel process - Solvent evaporation - Spinning disk technology - Spray chilling/cooling/congealing - Spray drying - Supercritical fluid technology - Templating - Vapor phase deposition ### TABLE 1.2 ### Microencapsulation Processes with Their Relative Particle Size Ranges | Approximate Particle/Capsule Size (µm) | Approximate Payload% | |---|---| | 150-5000 | 30-90 | | 2-1200 | 40-90 | | 2-100 | 20-60 | | 0.2-5000 | 1-100 | | 5-2000 | 5-50 | | 20-5000 | Varies | | Varies | Varies | | | 1-1000 | Varies | | 0.001-0.01 | 5-20 | | 1-1000 | Varies | | 200-5000 | 20-50 | | 100-3000 | 20-60 | | 1-10 | 5-50 | | 300-5000 | 5-40 | | 200-2000 | 5-20 | | 2-1000 | 30-60 | | 250-5000 | Varies | | 0.5-5 | Varies | | 0.2-5000 | 1-90 | | 0.5-1000 | Varies | | 0.5-1000 | Varies | | 5-1500 | Varies | | 20-1000 | 10-20 | | 10-400 | 5-50 | | 10-400 | 20-50 | Microencapsulation processes are usually categorized into two main groupings: chemical processes and mechanical or physical processes. These distinctions can be somewhat misleading as some processes classified as mechanical might involve or even rely upon a chemical reaction, and some chemical techniques rely solely on physical events. Some of the encapsulation processes are listed in Tables 1.1 and 1.2. The subsequent sections will briefly describe the most widely used encapsulation processes how- ever; the other chapters in the book will describe some of the techniques in detail. ### 1.6 ANNULAR JET (VIBRATIONAL NOZZLE) The Annular Jet technology was developed by the Southwest Research Institute, USA. The tech- nique involves two concentric jets, the inner containing the active ingredient (liquid core material) and the outer jet contains the liquid wall material, generally molten that solidifies when exiting the jet. Core-shell encapsulation can be done by using a laminar flow through a nozzle and an additional vibration of the nozzle or liquid. The vibrational nozzle also helps to control the droplet size giving a more uniform product with lower microcapsule sizes down to submicron diameters. The process involves the pumping of a dual fluid stream of liquid core and shell materials through concentric tubes/nozzles and forms droplets under the influence of vibration. The shell is then hardened by cooling (thermal gelation), chemical cross-linking, or solvent evaporation. Different types of extru- sion nozzles have been developed in order to optimize the process. The vibration has to be done in resonance with Rayleigh instability and leads to the formation of very uniform droplets as the dual fluid stream naturally breaks. ### 1.7 CENTRIFUGAL EXTRUSION This process is mostly used to encapsulate flavor oils. The liquid coextrusion process employs nozzles consisting of concentric orifices located on the outer circumference of a rotating cylinder. Simultaneously the liquid core material and the liquid wall material are fed through inner and outer orifice, respectively. The extruded rope of core material surrounded by wall material splits into round droplets directly after clearing the nozzle as the device rotates. By the action of surface ten- sion, the coating material envelops the core material, thus accomplishing encapsulation. The shell wall of the droplets is solidified by cooling or gelling bath to produce capsules. The microcapsules thus formed can be collected on a moving bed of fine-grained starch, which cushions their impact and absorbs unwanted coating moisture. The capsule size depends on the rotational speed. Typical wall materials include starch, maltodextrins, gelatin, polyethylene glycol (PEG). Particles produced by this method have diameter ranging from 150 to 2000 mm. ### 1.8 CENTRIFUGAL SUSPENSION SEPARATION This is a continuous high-speed process that involves mixing the core and wall materials and then adding to a rotating disk. The core materials with a coating of residual liquid ejects out of the disk. The microcapsules are then dried or chilled after removal from the disk. Solids, liquids, or suspen- sions of 30 µm to 2 mm can be encapsulated in this manner. ### 1.9 COCRYSTALLIZATION Cocrystallization encapsulation process utilizes sucrose as a matrix for the incorporation of core mate- rials. The cocrystallization of sucrose/core ingredient is induced by adding a core material to super- saturated sucrose syrup maintained at a high temperature to prevent crystallization) under vigorous mechanical agitation. The addition of core ingredient provides nucleation for the sucrose/core ingredi- ent mixture to crystallize. A substantial heat is released as the syrup reaches the temperature at which transformation and crystallization begin. It is very important to properly control the rates of nucleation and crystallization as well as the thermal balance during the various phases. By this process, core materials in a liquid form can be converted to a dry powdered form without additional drying. ### 1.10 COACERVATION A coacervate is a tiny spherical droplet of assorted organic molecules, which is held together by hydrophobic forces and the sizes ranging from 1 to 100 µm across. The name derives from the Latin coacervare, meaning “to assemble together or cluster." There are two types of coacervation pro- cesses available, namely, simple and complex coacervation. In simple coacervation, a desolvation agent is added for phase separation. Whereas in complex coacervation, a complexation is involved between two oppositely charged polymers. Complex coacervation refers to the phase separation of a liquid precipitate, or phase, when solutions of two hydrophilic colloids are mixed under suitable conditions. As an example in a complex coacervation process, the core material usually oil) is dispersed into a polymer solution e.g., a cationic aqueous polymer, and gelatin) to which a second polymer anionic, water soluble, and gum Arabic) solution is added. Deposition of the shell material onto the core particles occurs when the two polymers form a complex, which is generally triggered by the addition of salt or by changing the pH, temperature, or dilution of the medium. The micro- capsules can be stabilized by cross linking, desolvation, or thermal treatment. The microcapsules are usually collected by filtration or centrifugation, washed with an appropriate solvent, and subse- quently dried by standard techniques such as spray- or fluidized-bed drying to yield free-flowing, discrete particles. In recent years, modified coacervation processes have also been developed to overcome some of the problems encountered during a typical gelatin/gum acacia complex coacer- vation process. For example, a room-temperature process for the encapsulation of heat-sensitive ingredients such as volatile flavor oils by mixing the coating materials and inducing the phase separation coacervation) by adjusting the pH. The formation of a multilayered coacervated micro- capsule can be achieved by repeating the coacervation stages in which an additional layer of wall material is applied to the microcapsule at each passage. Complex coacervation can be used to produce microcapsules containing flavor, fragrant oils, liquid crystals, dyes, or inks as the core material. Capsules for carbonless paper applications were produced by complex coacervation process. ### 1.11 EMULSIFICATION/EMULSION POLYMERIZATION Emulsions can be used as a delivery vehicle for either water soluble and/or lipophilic active ingre- dient. In an emulsion polymerization, surfactant is dissolved in water until the critical micelle concentration is reached. The interior of the micelle provides the site necessary for polymeriza- tion. As the polymerization proceeds, these nuclei grow gradually and simultaneously entrap the core material to form the final microcapsules. The most common type of emulsion polymeriza- tion is an oil-in-water emulsion, in which droplets of monomer the oil) are emulsified (with sur- factants) in a continuous phase of water. Water-soluble active ingredients might be encapsulated in water-in-oil w/o) emulsions or double emulsions of the type water-in-oil-in-water w/o/w). Similarly lipophilic active ingredients might be encapsulated via o/w emulsions. Several technologies have been developed to produce highly uniform emulsion droplets, including single-drop technologies like microfluidics. The release profile of entrapped active ingredients could be more defined and predictable with uniform/narrow dispersed emulsions than the poly- dispersed ones. This can be very important in pharmaceutical applications. The emulsions can be dried by spray drying or freeze drying to provide a dry powder version of the encapsulated product. ### 1.12 FLUID-BED COATING Fluid-bed coating is a technique in which a coating is applied onto powder particles in a batch process or a continuous setup. Different types of fluid-bed coaters include top spray, bottom spray, and tangential spray and used for encapsulating solid or liquids absorbed into porous particles. The bottom spray version of the coating process is commonly known as Wurster coating and was developed in the 1950s and 1960s. Wurster coater relies upon a bottom positioned nozzle spray- ing the wall material up into a fluidized bed of core particles. Particles of the active ingredient, spheres or granules, are suspended in an upward-moving stream of air and then covered with a spray of liquid coating material in a temperature and humidity-controlled chamber of high veloc- ity air where the coating material is atomized. The coating material must have an acceptable viscosity to enable pumping and atomizing and must be thermally stable and should be able to form a film over a particle surface. The rapid evaporation of the solvent helps in the formation of an outer layer on the particles. The particles to be coated by fluid bed should ideally be spherical and dense and should have a narrow particle size distribution and good flowability. The whole cycle of suspending, spraying, and cooling is continued and repeated as needed until the desired coating thickness and weight is obtained. The size of the core particle for this technique is usu- ally large (~100 µm). This technique gives improved flexibility and control as compared to pan coating. ### 1.13 INTERFACIAL POLYMERIZATION Most commercial processes use this type of polymerization to produce small uniform capsules in the range of 20–30 micron diameter; however, the process can be tuned to produce large microcap- sules. The size of these microcapsules and the properties of the wall material/polymer matrix can be altered by using different monomers, utilizing additives, and adjusting reaction conditions. The encapsulation occurs by wall formation around the dispersed core material via the rapid polymer- ization of monomers at the surface of the droplets or particles. The solution of a multifunctional monomer in the core material is dispersed in an aqueous phase. The polymerization is commenced at the surfaces of the core droplets forming the capsule walls, by adding a reactant to the monomer dispersed in the aqueous phase. ### 1.14 INCLUSION COMPLEXATION Encapsulation via inclusion complexation host-guest complexation) occurs at a molecu- lar level. This is also known as molecular encapsulation process or host-guest complexation. Cyclodextrin is one of the most useful host molecules for a variety of encapsulation applica- tions from food to pharma industries. There are generally three types of cyclodextrins such as a-cyclodextrin made up of six glucopyranose units), ß-cyclodextrin made up of seven gluco- pyranose units), and y-cyclodextrin made up of eight glucopyranose units). They are prepared from partially hydrolyzed starch maltodextrin) by an enzymatic process. The external part of the cyclodextrin molecule is hydrophilic, whereas the internal part is hydrophobic. The guest molecules, which are apolar, can be entrapped into the apolar internal cavity through a hydro- phobic interaction. The size of the cavity varies depending on the cyclodextrin. Depending on the type of cyclodextrin one or more molecules can be entrapped in the interior of the mol- ecule. Cyclodextrin molecules form inclusion complexes with compounds that can fit dimen- sionally into their central cavity. ### 1.15 IONIC GELATION (HYDROGEL MICROSPHERES) This is one of the simplest methods for making encapsulated material. Microspheres are microbeads composed of a biopolymer gel network entrapping an active. Calcium alginate gel is the best known gelling system used for the preparation of gel beads to encapsulate a wide variety of active agent, such as oil droplets containing aroma, cells, probiotics, yeast, enzymes, etc. Gelation of alginate in the presence of divalent or trivalent cations can be easily controlled. Microspheres made of gel-type polymers, such as alginate or pectinate are produced by dissolving the polymer in an aqueous solu- tion then, suspending the active ingredient in the mixture followed by extruding through a precision device the dripping tool can be simply a pipette, syringe, vibrating nozzle, spraying nozzle, jet cut- ter, atomizing disk, coaxial air-flow, or electric field) producing microdroplets. These microdroplets are hardened by cross-linking the wall material or polymer chain by using di- or multivalent metal ion such as calcium chloride) aqueous solutions. There are several advantages of these methods that involve an all-aqueous system; the particle size of microspheres can be controlled by using various size extruders or by varying the polymer solution flow rates. Alternatively, the extrusion or dropping method can be used with a concentric nozzle coextrusion), to prepare core-shell type of encapsu- lates with a lipophilic core and a shell of a gel network. Similarly, specially formulated emulsions can be used to make microspheres. Calcium chloride can be added to an emulsion of water droplets of an alginate solution and active ingredient in vegetable oil. This results in the "break-up" of the emulsion and microbeads are formed by the gelation of the alginate droplets. Alternatively, both alginate and calcium in an insoluble form such as calcium carbonate) can already be present in the water phase of the emulsion. Upon addition of an oil-soluble acid such as acetic acid) the pH decreases, liberating free calcium ions in the system and initiating the gel formation of alginate droplet with calcium. ### 1.16 LIPOSOMES A liposome is a tiny vesicle generally made from phospholipids with diameter ranges from 25 nm to 10 µm capable of entrapping both hydrophobic and hydrophilic active ingredients. The lipo- somes consist of one or more layers of lipids and have been used for delivery of vaccines, hor- mones, enzymes, and vitamins. The properties such as permeability, stability, surface activity, and affinity can be varied through size and lipid composition variations. The capsules can range from 25 nm to several microns in diameter, are easy to make, and can be stored by freeze-drying. Kirby and Gregoriadis have devised a method to encapsulate at high efficiency, which is easy to scale-up and uses mild conditions appropriate for enzymes. The liposomes impart stability to water-soluble material in high water activity application. Another unique property of liposomes is the targeted delivery of the encapsulated ingredient their content at a specific and well-defined temperature in a specific location. The liposome bilayer is instantly broken down at the transi- tion temperature of the phospholipids, typically around 50°C, at which temperature the content is immediately released. ### 1.17 LYOPHILIZATION Lyophilization or freeze-drying is a simple technique that is suitable for the encapsulation of aro- mas, water-soluble essences, drugs, and importantly used for the dehydration of almost all heat- sensitive materials. It is a process that requires a long dehydration period. The retention of volatile compounds during lyophilization is dependent upon the chemical nature of the system. ### 1.18 MELT EXTRUSION (MELT INJECTION) Extrusion as applied for encapsulating flavor ingredient(s) is a relatively low-temperature entrap- ping method, which involves forcing a core material in a molten carbohydrate mass composed of more than one ingredient, such as sucrose, maltodextrin, glucose syrup, glycerine, and glucose) through a series of dies into a bath of dehydrating liquid. The process temperature and the pressure are around ~115°C or below and typically <100 psi, respectively. The coating material hardens on contacting the liquids, forming an encapsulating matrix to entrap the core material, which can then be separated from the liquid bath, dried, and sized. The polymer matrices and the plasticizers used can be modified to produce the capsules for controlled release application. ### 1.19 PAN COATING The pan coating process is amongst the oldest industrial techniques used particularly in the pharma- ceutical industry for coating tablets or forming coated particles. The concept of the technology was initially patented by William E. Upjohn in the nineteenth century. It generally required large core particles and produces the coated tablets that we are familiar with. The tablets/particles are tumbled in a pan or other device while the coating material is sprayed/applied slowly. In another aspect solid particles are mixed with a dry coating material and the temperature is raised so that the coating material melts and encloses the core particles, and then is solidified by cooling. On the other hand, the coating material can be gradually applied to core particles tumbling in a vessel rather than being wholly mixed with the core particles from the start of encapsulation. ### 1.20 PHASE SEPARATION Phase separation process takes advantage of the phenomenon called polymer-polymer incompat- ibility. The process utilizes two polymers that are soluble in a common solvent; yet do not mix with one another in the solution. The polymers form two separate phases; one polymer intended to form the capsule walls, the other incompatible polymer meant to induce the separation of the two phases, but not meant to be part of the capsule wall material. This process is somewhat related to the com- plex coacervation process. The phase separation process is considered as the oldest true encapsula- tion technology first developed by the National Cash Register Company for carbonless copy-paper. Microencapsulation by coacervation involves the phase separation of one or more hydrocolloids from the initial solution, and the subsequent deposition of the newly formed coacervate phase around the active ingredient suspended or emulsified in the same reaction media. The size of the microcapsules formed may be in the range of 10-250 µm. ### 1.21 SPRAY DRYING Microencapsulation by spray-drying is a low-cost commercial process, which is mostly used for the encapsulation of fragrances, oils, and flavors. Spray-drying encapsulation has been used in the food industry since the late 1950s to convert liquids to powders. The process is economical and flexible, in that it offers substantial variation in microencapsulation matrix, and produces particles of good qual- ity. The flavor or ingredient to be encapsulated is added to the carrier and homogenized to create small droplets. The resultant emulsion is fed into the hot chamber of the spray dryer where it is atomized through a nozzle or spinning wheel. The size of the atomizing droplets depends on the surface tension and viscosity of the liquid, pressure drop across the nozzle, and the velocity of the spray. The size of the atomizing droplets also determines the drying time and particle size. Hot air contacts the atomized droplets; the shell material solidifies onto the core particles as the solvent evaporates, and leaving dried particles. It is immensely suitable for the continuous manufacture of dry solids as either powder, granu- lates, or agglomerates from liquid feeds. The microcapsules obtained are of polynuclear or matrix type. ### 1.22 SPRAY CONGEALING The spray congealing is also known as spray chilling or spray cooling. In spray chilling and spray cooling, the core and wall mixtures are atomized into the cooled or chilled air, which causes the wall to solidify around the core. This technique can be accomplished with spray-drying equipment when the protective coating is applied as a melt. The process involves the dispersion of the core material in a coating material melt. Upon spraying, the hot mixture into a cool air stream the coat- ing solidification (and microencapsulation) is accomplished. In spray cooling, the coating material can be some form of vegetable oil or its derivatives, fat (melting points of 45°C–122°C), as well as hard mono- and diacylglycerols (melting points of 45°C-65°C). In spray chilling, the coating material is typically a fractionated or hydrogenated vegetable oil with a melting point in the range of 32°C-42°C. Microcapsules prepared by spray chilling and spray cooling are insoluble in water due to the hydrophobic coating. Consequently, these techniques can be useful for encapsulating water-soluble core materials. ### 1.23 SPINNING DISK (CENTRIFUGAL SUSPENSION SEPARATION) This technology is rapid, cost-effective, and relatively simple and has high production efficiencies. The technology was developed by Robert E. Sparks in 1980s. The suspensions of core particles in liquid shell material are poured into a rotating disk. Due to the spinning action of the disk, the core particles become coated with the shell material and the coated particles are then cast from the edge of the disc by centrifugal force. The shell material of the coated particles is solidified by external means (usually cooling). The microcapsules are then dried or chilled after removal from the disk, and the whole process can take between a few seconds to minutes. Solids, liquids, or suspensions of 30 µm to 2 mm can be encapsulated in this manner. Coatings can be 1-200 µm in thickness and include fats, PEG, diglycerides, and other meltable substances. ### 1.24 SOLVENT EVAPORATION It is one of the most extensively used methods of microencapsulation. For example, the aqueous solution of the drug (may contain a viscosity building or stabilizing agent) is added to a water immiscible volatile organic phase consisting of the polymer solution in solvents like dichlorometh- ane or chloroform with vigorous stirring to form the primary water in oil emulsion. The resulting emulsion is then added drop wise to a stirring aqueous solution containing an emulsifier/stabilizer like poly (vinyl alcohol) or poly (vinyl pyrrolidone) to form the multiple emulsion w/o/w) forming small polymer droplets containing encapsulated material. Droplets hardened to produce the cor- responding polymer microcapsules. This hardening process is accomplished by the removal of the solvent from the polymer droplets either by solvent evaporation by heat or reduced pressure), or by solvent extraction. The microspheres can then be washed and dried. ### 1.25 SUPERCRITICAL FLUID ASSISTED ENCAPSULATION Supercritical fluids are highly compressed gasses that possess several properties of both liquids and gases. The supercritical CO₂ or N₂O are normally used for this type of encapsulation process. The microcapsules are formed when the supercritical fluid under high pressure containing the active ingredient and the shell material are released through a small nozzle at atmospheric pressure. The sudden drop in pressure causes desolvation of the shell material, which is then deposited around the active ingredient (core) and forms a coating layer. Different core materials such as pesticides, pigments, vitamins, flavors, and dyes are encapsulated using this method. Although there are some advantages of this process, one of the requirements is that both the active ingredient and the shell material should be soluble in supercritical fluids. ### 1.26 CONTROLLED RELEASE Controlled release is a term referring to the presentation or delivery of compounds in response to stimuli (such as pH, enzymes, light, magnetic fields, temperature, ultrasonic, osmosis, and more recently electronic control) or time. It may be defined as a method by which one or more active ingredients are made available at a desired site and time at a specific rate. Various applications include in areas such as pharmaceuticals, agriculture, cosmetics, food sciences, and personal care. This terminology commonly refers to time dependant release, sustained release, pulse release, delayed release in oral dose formulations. There are many advantages as well as challenges such as: biocompatibility, the fate of controlled release system if not biodegradable, cost of formulation, etc.) associated with designing a controlled release system/formulation. The science of controlled release originates from the development of oral sustained-release products in the late 1940s and early 1950s, the development of controlled release of marine anti-foulants in the 1950s and con- trolled release fertilizer in the 1970s. Delivery of active ingredient is usually effected by dissolu- tion, degradation, or disintegration of the shell material. Other encapsulation technologies including enteric coatings can