EMI Unit 1 PDF
Document Details
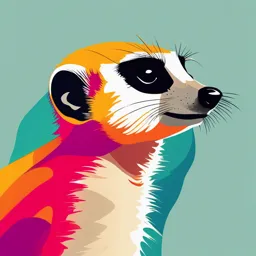
Uploaded by DesirousPrehistoricArt
2024
OCR
Tags
Summary
This document provides an introduction to EMI Unit 1, covering topics like instrumentation, measurement systems, and electronic instruments. It includes various definitions, classifications, block diagrams, and examples.
Full Transcript
EMI UNIT 1 UNIT I: BLOCK SCHEMATIC OF MEASURING SYSTEM INTRODUCTION ✓What is Instrumentation? Instrumentation is a branch of engineering, related to study of various instruments and their control. ✓ An instrument is a device that measures a physical or e...
EMI UNIT 1 UNIT I: BLOCK SCHEMATIC OF MEASURING SYSTEM INTRODUCTION ✓What is Instrumentation? Instrumentation is a branch of engineering, related to study of various instruments and their control. ✓ An instrument is a device that measures a physical or electrical quantity such as flow, temperature, current, voltage, level, distance, angle, or pressure. ✓What is measurement? The measurement of a given parameter or quantity is the act of a quantitative comparison between a predefined standard and an unknown quantity to be measured. PURPOSE OF MEASUREMENT SYSTEM ✓ For each and every physical quantity, the output should be in numerical. So as to make anyone to understand. e.g., 5kg, 7mts ✓ The purpose of measurement system is to present measured value to numerical value. ✓ Measured Value vs True Value: Measured Value: Any value calculated from measurement system or measuring instrument. True Value: Any value calculated from rated value. ✓ ERROR: The deviation of measured value from true value. 3 ADVANTAGES OF INSTRUMENTATION SYSTEM 1. Remote measurement 2. Accurate measurement 3. Measurement in adverse conditions e.g., Nuclear rector, Space applications etc. 4. Convenience in : recording of data, printout etc. 5. Reduction in size ADVANTAGES OF ELECTRONIC INSTRUMENTS 1. Different physical quantities can be converted into electrical signal by transducers. 2. Electrical signal can amplified, multiplexed, filtered and measured easily. 3. Electrical signals can be transmitted over long distances by wire or radio link etc. 4. Electrical signal can be converted from A/D or D/A signal. 5. Digital signal are compatible with computers. 6. Many measurements can be carried simultaneously. 20-Aug-24 5 CLASSIFICATION OF INSTRUMENTS Instruments can be classified into many categories, one classification is given as under. 1. Monitoring of processes and operations 2. Control of processes and operations 3. Experimental engineering and analysis 20-Aug-24 6 CLASSIFICATION OF INSTRUMENTS contd.. 1. Monitoring of processes and operations ❑ Monitoring function ❑ Simply indicate the condition ❑ Cannot control any function ❑ e.g., Thermometers 20-Aug-24 7 CLASSIFICATION OF INSTRUMENTS contd.. 2. Control of processes and operations ❑ Involves controlling of function ❑ e.g., home-heating system using a thermostat function ❑ A temperature-measuring instrument senses the room temperature, thus providing information necessary for proper functioning of control system. 20-Aug-24 8 CLASSIFICATION OF INSTRUMENTS contd.. 3. Experimental engineering and analysis ❑ In R&D work, measuring instruments are used in carrying out experiments ❑ e.g., Measurement of current, voltage etc., ❑ The application of instruments is specifically intended for experimental purpose only. 20-Aug-24 9 Block Schematics of Measuring Systems 20-Aug-24 10 Measurand: The quantity to be measured is known as measurand. Transducer: A device that converts a physical quantity into electrical quantity or vice versa. Signal Conditioning: Amplification, Multiplexing, Modulation, Demodulation, Sampling, Filtering, ADC etc. Display / Record: The Quantity is recorded using X-Y or strip chart recorders or displayed on monitors. Any general measuring system will have a transducer that converts the physical quantity into an electrical form so that the signal may be modified according to the requirements. Signal conditioning devices will carryout this task, and the parameter to be measured is displayed or recorded. 20-Aug-24 11 Block Schematic of a Telemetry System 20-Aug-24 12 Telemetry stands for measurement of a parameter from a distance The parameter is measured at one place and recorded at a remote point ( distinct) 20-Aug-24 13 BLOCK SCHEMATIC OF CONTROL INSTRUMENTATION Here a feedback loop exists between the input and the output to control the parameter to be measured. 20-Aug-24 14 Data Process System 20-Aug-24 15 In this, digital control techniques are employed using microprocessor or microcontrollers or personal computers as shown in block diagram. The analogue signal is converted to a digital signal by using ADC and is processed by a MP or MC or PC 20-Aug-24 16 Objectives of Measurement 1. To establish the validity of design. 2. To predict the limit of capacity. 3. To provide information needed to supplement further analytical work. 20-Aug-24 17 PERFORMANCE CHARACTERISTICS A knowledge of the performance characteristics of an instrument is essential for selecting the most suitable instrument for specific measuring jobs The performance characteristics may be broadly divided into two groups, namely Static characteristics Dynamic characteristics 20-Aug-24 18 STATIC AND DYNAMIC CHARACTERISTICS Static :It Describes the performance of measuring instruments when subjected to low freq inputs. Dynamic: Transient behaviour between the time a measured quantity changes value and the time when the instrument output attains a steady value in response. 20-Aug-24 19 STATIC CHARACTERISTICS Accuracy Precision Resolution Sensitivity 20-Aug-24 20 ACCURACY The accuracy of an instrument is a measure of how close the output reading of the instrument is to the true value. If the accuracy of a 100V voltmeter is + or -1%, the maximum error for any reading will not exceed + or -1V. % of True value= Measured value- True value * 100 True value 20-Aug-24 21 PRECISION Precision is a term that describes the ability of measuring system to reproduce the same output among several independent measurements under specified conditions or with in a given accuracy Ex: I/P is applied to transducer with the known i/p ,if the measuring instrument produces its o/p which lies between +/- 1%, then precision of measuring instrument can be +/-1%. There fore it is said to be highly precise if it produces same o/p for an applied i/p through the measurent is repeated no.of times. 20-Aug-24 22 ACCURACY and PRECISION 20-Aug-24 23 ACCURACY vs. PRECISION 20-Aug-24 24 RESOLUTION Resolution is the smallest change in the measured value to which the instrument can respond. For example, a 100V voltmeter may not be able to measure 100mV. Only when minimum input is 0.5V, the needle may deflect or the reading changes from 0. Any input or change in input less than 0.5V may have no effect on the instrument. Therefore, the resolution for that particular instrument is 0.5V. 20-Aug-24 25 SENSITIVITY The ratio of change in the output signal to the change in the input is called sensitivity. For a voltmeter, it is the ratio ∆V0 / ∆Vi. If Vi changes by 0.1V, the output reading should also change by 0.1V. This change in V0 for a change in Vi is expressed as sensitivity. 20-Aug-24 26 DYNAMIC CHARACTERISTICS Repeatability Reproducibility Fidelity Speed of Response Lag 20-Aug-24 27 REPEATABILITY Repeatability describes the closeness of output readings when the same input is applied repetitively over a short period of time, with the same measurement conditions, same instrument and observer, same location, and same conditions of use maintained throughout 20-Aug-24 28 REPRODUCIBILITY Reproducibility describes the closeness of output readings for the same input when there are changes in the method of measurement, observer, measuring instrument, location, conditions of use, and time of measurement. 20-Aug-24 29 FIDELITY Fidelity of a system is defined as the ability of the system to reproduce the output in the same form as the input. It is the degree to which a measurement system indicates changes in the measured quantity without any dynamic error. Supposing if a linearly varying quantity is applied to a system and if the output is also a linearly varying quantity the system is said to have 100 percent fidelity. Note: dynamic error is the difference between the true value of the quantity changing with time and the value indicated by the instrument. 20-Aug-24 30 SPEED OF RESPONSE It is defined as the rapidity with which an instrument or measurement system responds to changes in measured quantity. This shows how active and fast the system is. Speed measuring instrument example: wind speed meter 20-Aug-24 31 LAG Speed of response of the instrument is referred in terms of lag. If the input is changing rapidly, output must also change exactly at the same rate, in an ideal case. The time delay in the output to change with the input is termed as lag. 20-Aug-24 32 The dynamic characteristics of the instruments are represented by differential equations. The order of the equation represents the order of the instrument. But, in general, the instruments are classified as 1. Zero order 2. First order 3. Second order Zero-order instrument: E.g. Linear potentiometer. It is represented by the equation ------ a0 y = bx First-order instrument: E.g. Mercury thermometer. It is represented by the differential equation ------ a1 (dy/dt) – a0 y = bx Second-order instrument: E.g. Spring balance. These are represented by the equation ------ a2 d2y/dx2 + a1 dy/dx + a0 y + bx = 0 TYPES OF ERRORS 20-Aug-24 34 GROSS ERRORS These are basically human errors caused by the operator or person using the instrument. The instrument may be good and may not give any error but still the measurement may go wrong due to the operator. The different types of gross errors are: 1. Taking wrong readings. 2. Reading with parallax error. 3. Incorrect adjustments of zero and full-scale adjustments. 4. Improper applications of instruments: Using a 0-100V voltmeter to measure 0.1V, etc. 5. Wrong computation 20-Aug-24 35 SYSTEMATIC ERRORS 20-Aug-24 36 OBSERVATIONAL ERRORS Observational errors are errors introduced by the observer. The most common error is the parallax error introduced in reading a meter scale, and the error of estimation when obtaining a reading from a meter scale. These errors are caused by the habits of individual observers. For example, an observer may always introduce an error by consistently holding his head too far to the left while reading a needle and scale reading. 20-Aug-24 37 ENVIRONMENTAL ERRORS Ambient parameters such as temperature, pressure, humidity, magnetic and electrostatic fields, dust, and other such external parameters can effect the performance of the instrument. Such errors can be avoided by air-conditioning, magnetic shielding, cleaning the instruments and housing the instruments properly depending on the application and type of the instrument. 20-Aug-24 38 INSTRUMENTAL ERRORS Even if human errors are avoided or proper care is taken to see that such errors do not occur, error can still occur in measurements due to the instrument. The possible reasons can be as follows: 1. Friction in bearings of various moving components can cause incorrect readings. 2. Irregular spring tension in analog meters. 3. Calibration errors due to aging. 4. Zero setting not adjusted properly. 5. Full-scale setting not adjusted properly. 6. Faulty display circuit in digital instruments. These errors can be avoided by: 1. Applying correction factors. 2. Selecting suitable instruments. 3. Calibrating the instruments. 20-Aug-24 39 RANDOM ERRORS These are errors that remain after gross and systematic errors have been substantially reduced or at least accounted for. Random errors are generally an accumulation of a large number of small effects and may be of real concern only in measurements requiring a high degree of accuracy. Such errors can be analyzed statistically. These errors are due to unknown causes, not determinable in the ordinary process of making measurements. Such errors are normally small and follow the laws of probability. Random errors can thus be treated mathematically. 40 STATISTICAL ANALYSIS This method is employed to estimate the value or error when unpredictable errors or random errors are dominant. When the reason for specific error cannot be identified and the deviation from the true value is to be estimated, the statistical analysis method is to be employed. This will give the deviation from the true value, and the correctness of the readings taken. The statistical analysis is employed by taking a large number of readings of a particular parameter, and calculations are made in the following ways: 20-Aug-24 41 ARITHMETIC MEAN The most probable value of a measured variable is the arithmetic mean of the number of readings taken. The best approximation is possible when the number of readings of the same quantity is very large. The arithmetic mean of n measurements at a specific count of the variable x is given by the expression 20-Aug-24 42 DEVIATION FROM THE MEAN This is the departure of a given reading from the arithmetic mean of the group of readings. If the deviation of the first reading, x1, is called d1 and that of the second reading x2 is called d2, and so on, The deviations from the mean can be expressed as d1 = x1 – x¯, d2 = x2 – x¯..., similarly dn = xn – x¯ The deviation may be positive or negative. The algebraic sum of all the deviations must be zero. 20-Aug-24 43 AVERAGE DEVIATIONS The average deviation is an indication of the precision of the instrument used in measurement. Average deviation is defined as the sum of the absolute values of the deviation divided by the number of readings. Average deviation may be expressed as Dav = |d1|+ |d2|+ |d3|+……. |dn| = ∑ |d| _______________________ ____ n n where Dav = average deviation |d1|, |d2|, …, |dn| = Absolute value of deviations and n = total number of readings Highly precise instruments yield a low average deviation between readings. 20-Aug-24 44 STANDARD DEVIATION 20-Aug-24 45 20-Aug-24 46 ROOT SUM SQUARES (RSS) FORMULA In the statistical analysis method to associate the probable error, the number of readings of the same parameters is taken, and graphs are plotted. A simple mathematical formula to estimate the probable error, by taking only one set of readings, is the Root Sum Squares (RSS) formula. If a parameter N is a function of other parameters u1, u2, …… un, N is computed by measuring u1, u2, …… un, and each measurement of u1, u2, …… un has a certain degree of error or uncertainty; the overall error, in the computed value of N, ERSS is given by 20-Aug-24 47 PROBLEM 20-Aug-24 48 TYPES OF INSTRUMENTS Analogue ammeters and voltmeters are classified together, since there is no basic difference in their operating principles. The essential requirements of a measuring instrument are: (a) that its introduction into the circuit where measurements are to be made, should not alter the circuit conditions, and (b) the power consumed by it be small. The following types of instrument are mainly used as ammeters and voltmeters. 1. PMMC 2. Moving Iron 3. Electrodynamometer 4. Hot wire 5. Thermocouple 6. Induction type 7. Electrostatic 8. Rectifier 20-Aug-24 49 BASIC METER MOVEMENT The action of the most commonly dc meter is based on the fundamental principle of the motor. The motor action is produced by the flow of a small current through a moving coil, which is positioned in the field of a permanent magnet. This basic moving coil system is often called the D’Arsonval galvanometer. 20-Aug-24 50 The D’Arsonval movement shown in Fig. 2.1 employs a spring-loaded coil through which the measured current flows. The coil (rotor) is in a nearly homogeneous field of a permanent magnet and moves in a rotary fashion. The amount of rotation is proportional to the amount of current flowing through the coil. A pointer attached to the coil indicates the position of the coil on a scale calibrated in terms of current or voltage. It responds to dc current only, and has an almost linear calibration. The magnetic shunt that varies the field strength is used for calibration. 20-Aug-24 51 PERMANENT MAGNETIC MOVING COIL MOVEMENT A coil suspended in the magnetic field of a permanent magnet in the shape of a horse-shoe. The coil is suspended so that it can rotate freely in the magnetic field. When current flows in the coil, the developed (electromagnetic) torque causes the coil to rotate. The electromagnetic (EM) torque is counter balanced by a mechanical torque of control springs attached to the movable coil. The equation for the developed torque, derived from the basic law for electromagnetic torque is τ = B x A x I x N 20-Aug-24 52 where τ = torque, Newton-meter B = flux density in the air gap ,Wb/m2 A = effective coil area (m2) N = number of turns of wire of the coil I = current in the movable coil (amperes) The equation shows that the developed torque is proportional to the flux density of the field in which the coil rotates, the current coil constants (area and number of turns). Since both flux density and coil constants are fixed for a given instrument, the developed torque is a direct indication of the current in the coil. The pointer deflection can therefore be used to measure current. 20-Aug-24 53 20-Aug-24 54 20-Aug-24 55 ELECTRODYNAMOMETER The D’Arsonval movement responds to the average or dc value of the current flowing through the coil. If ac current is sought to be measured, the current would flow through the coil with positive and negative half cycles, and hence the driving torque would be positive in one direction and negative in the other. Therefore, to measure ac on a D’Arsonval movement, a rectifier has to be used to produce a unidirectional torque. This rectifier converts ac into dc and the rectified current deflects the coil. Another method is to use the heating effect of ac current to produce an indication of its magnitude. This is done using an electrodynamometer (EDM). 20-Aug-24 56 ELECTRODYNAMOMETER AS AN AMMETER The electrodynamometer can be used by slightly modifying the PMMC movement. A movable coil is used to provide the magnetic field in an electrodynamometer, instead of a permanent magnet, as in the D’Arsonval movement. This movable coil rotates within the magnetic field. The EDM uses the current under measurement to produce the required field flux. A fixed coil, split into two equal halves provides the magnetic field in which the movable coil rotates, as shown in Fig. 2.6 (a). The coil halves are connected in series with the moving coil and are fed by the current being measured. 57 20-Aug-24 58 The fixed coils are spaced far apart to allow passage for the shaft of the movable coil. The movable coil carries a pointer, which is balanced by counter weights. Its rotation is controlled by springs, similar to those in a D’Arsonval movement. The complete assembly is surrounded by a laminated shield to protect the instrument from stray magnetic field which may affect its operation. The torque developed by a coil suspended in a magnetic field is given by τ = B x A x I x N The torque which deflects the movable coil is directly proportional to the coil constants (A and N), the strength of the magnetic field in which the coil moves (B), and the current (I) flowing through the coil. 20-Aug-24 59 ELECTRODYNAMOMETER AS A VOLTMETER The addition of a series multiplier converts the basic EDM into a voltmeter Fig. 2.6 (b) which can be used for ac and dc measurements. 20-Aug-24 60 ELECTRODYNAMOMETER AS WATTMETER When it is used as a wattmeter, the fixed coils, are connected in series and carries the total line current. The movable coil is connected in series with a current-limiting resistor across the power line, and carries a small current. The deflection of the movable coil is proportional to the product of the instantaneous value of current in the movable coil and the total line current. 20-Aug-24 61 DC VOLTMETER To use the basic meter as a dc voltmeter, it is necessary to know the amount of current required to deflect the basic meter to full scale. This current is known as full scale deflection current (Ifsd). Sensitivity = 1/Ifsd = 1/50 μ A = 20 k Ω /V. Hence, a 0 – 1 m A would have a sensitivity of 1 V/1 mA = 1 kΩ/V or 1000 Ω. The sensitivity is based on the fact that the full scale current of 50 μ A results whenever 20,000 Ω of resistance is present in the meter circuit for each voltage applied. 20-Aug-24 62 A basic D’Arsonval movement can be converted into a dc voltmeter by adding a series resistor known as multiplier, as shown in Fig. 4.1. The function of the multiplier is to limit the current through the movement so that the current does not exceed the full scale deflection value. The multiplier limits the current through the movement, so as to not exceed the value of the full scale deflection Ifsd. 20-Aug-24 63 20-Aug-24 64 MULTIRANGE VOLTMETER As in the case of an ammeter, to obtain a multi range ammeter, a number of shunts are connected across the movement with a multi- position switch. Similarly, a dc voltmeter can be converted into a multi range voltmeter by connecting a number of resistors (multipliers) along with a range switch to provide a greater number of workable ranges. Figure 4.2 shows a multi range voltmeter using a three position switch and three multipliers R1, R2, and R3 for voltage values V1, V2, and V3. 20-Aug-24 65 MODIFIED MULTIRANGE VOLTMETER Here the multiplier resistances are connected in a series string, and the range selector selects the appropriate amount of resistance required in series with the movement. This arrangement is advantageous compared to the previous circuit, because all multiplier resistances except the resistor R4 have standard values. R4 has to be specially manufactured. 20-Aug-24 66 20-Aug-24 67 EXTENDING VOLTMETER RANGES The range of a voltmeter can be extended to measure high voltages, by using a high voltage probe or by using an external multiplier resistor, as shown in Fig. 4.4. In most meters the basic movement is used on the lowest current range. Values for multipliers can be determined using the expression 20-Aug-24 68 SENSITIVITY It is the ratio of total circuit resistance (RT) to the voltage range (V). It is essentially the reciprocal of the full scale deflection current of the basic movement. S = RT / V = 1 / IFSD ohm/v Sensitivity ‘S’ can be used to calculate the value of multiplier resistance in a DC voltmeter as RT = RM + RS S x V = RM + RS, RS = (S x V ) – RM Where, RM is internal resistance, RS is multiplier resistance, RT is total circuit resistance, V is the voltage and S is sensitivity 20-Aug-24 69 LOADING EFFECT OF A DC VOLTMETER When selecting a meter for a certain voltage measurement, it is important to consider the sensitivity of a DC voltmeter. A low sensitivity meter may give a correct reading when measuring voltages in a low resistance circuits, but it is certain to produce, unreliable readings in a high resistance circuit. A voltmeter when connected across shunt for that portion of the circuit, reducing the total equivalent resistance of that portion. The meter then indicates a lower reading than what excited before the meter was connected. This is called the loading effect of an instrument and is caused by low sensitivity instruments. 20-Aug-24 70 DC AMMETER The PMMC galvanometer constitutes the basic movement of a dc ammeter. Since the coil winding of a basic movement is small and light, it can carry only very small currents. When large currents are to be measured, it is necessary to bypass a major part of the current through a resistance called a shunt. The resistance of shunt can be calculated using conventional circuit analysis. 20-Aug-24 71 Rm = internal resistance of the movement. Ish = shunt current Im = full scale defl ection current of the movement I = full scale current of the ammeter + shunt (i.e. total current) Since the shunt resistance is in parallel with the meter movement, the voltage drop across the shunt and movement must be the same. The total current I to be ‘n’ times larger than Im. This is called a multiplying factor and relates the total current and meter current. 20-Aug-24 72 The general requirements of a shunt are as follows: 1. The temperature coefficients of the shunt and instrument should be low and nearly identical. 2. The resistance of the shunt should not vary with time. 3. It should carry the current without excessive temperature rise. 4. It should have a low thermal emf. 20-Aug-24 73 MULTIRANGE AMMETERS The current range of the dc ammeter may be further extended by a number of shunts, selected by a range switch. Such a meter is called a multi range ammeter, shown in Fig. 3.2. The circuit has four shunts R1, R2, R3 and R4, which can be placed in parallel with the movement to give four different current ranges. Switch S is a multi position switch. 20-Aug-24 74 This switch is so designed that when the switch position is changed, it makes contact with the next terminal (range) before breaking contact with the previous terminal. Multi range ammeters are used for ranges up to 50A. The resistance used for the various ranges are of very high precision values, hence the cost of the meter increases. 20-Aug-24 75 THE ARYTON SHUNT OR UNIVERSAL SHUNT The Aryton shunt eliminates the possibility of having the meter in the circuit without a shunt. This advantage is gained at the price of slightly higher overall resistance. Figure 3.3 shows a circuit of an Aryton shunt ammeter. 20-Aug-24 76 When the switch is in position “1”, resistance Ra is in parallel with the series combination of Rb, Rc and the meter movement. Hence the current through the shunt is more than the current through the meter movement, thereby protecting the meter movement and reducing its sensitivity. If the switch is connected to position “2”, resistance Ra and Rb are together in parallel with the series combination of Rc and the meter movement. Now the current through the meter is more than the current through the shunt resistance. If the switch is connected to position “3” Ra, Rb and Rc are together in parallel with the meter. Hence maximum current flows through the meter movement and very little through the shunt. 20-Aug-24 77 EXTENDING OF AMMETER RANGES The range of an ammeter can be extended to measure high current values by using external shunts connected to the basic meter movement (usually the lowest current range), as given in Fig. 3.5. Note that the range of the basic meter movement cannot be lowered. For example, if a 100 mA movement with 100 scale division is used to measure 1 μA, the meter will deflect by only one division. Hence ranges lower than the basic range are not practically possible. 20-Aug-24 78 AC VOLTMETER AC voltmeters are designed in a manner so that they can measure the AC voltage under measurement. The main difference between AC voltmeter circuit and DC voltmeter circuit is the usage of a rectifier. The rectifier is used in order to transform the AC voltage into DC voltage. 20-Aug-24 79 BLOCK DIAGRAM OF AC VOLTMETER 20-Aug-24 80 To develop a basic understanding of AC voltmeter, it is crucial to have a brief idea of the block diagram of AC voltmeter circuit. The block diagram of AC voltmeter resembles the block diagram of DC voltmeter except the fact that rectifier is used in case of AC voltmeter. The input to be measured is given to the attenuator circuit which performs the operation of selection of a particular range of voltage. The output of attenuator is given to rectifier which converts the AC voltage into pulsating DC voltage. Then the final output of DC amplifier is given to the PMMC meter. 20-Aug-24 81 The rectifier can be used before the multistage amplifier or after the amplifier. This depends on the type of amplifier used in AC voltmeter. If we are using multistage AC amplifier, then the rectifier circuit will be used after the amplifier. On the contrary, if the multistage amplifier used is DC, then the rectifier should be used before it. The amplifier is a vital component because it amplifies the signal which got attenuated during the measurement procedure. The usage of the amplifier increases the cost of the circuit. If the designing needs to be economical, then we should use multistage DC amplifier. 20-Aug-24 82 CLASSIFICATION OF AC VOLTMETERS There can be three types of AC voltmeters based on the type of measurement it provides. These are average reading, peak reading and true RMS reading AC voltmeter. 20-Aug-24 83 AVERAGE READING AC VOLTMETER The average reading voltmeter measures the average value of the AC voltage. The scale calibrated in average reading voltmeter is in terms of RMS value. This is because most of the AC voltages to be measured are in sinusoidal form. Besides, it is economical to measure the average value of AC voltage rather than its true RMS value. Average reading voltmeters can be designed in various manners such as by using vacuum diode, semiconductor diode or a full wave rectifier. In this, the voltage-current characteristics must be linear, because the change in voltage is measured by the change in current. Let’s understand first the basic construction of AC voltmeters using vacuum diode. 20-Aug-24 84 The requisite factor in such voltmeters is that the resistance of plates of vacuum tube should be low so that it does not create variation in linear characteristics of plate voltage and current. For this, the resistance used in series with vacuum tube should possess sufficiently high value so that the linear characteristics can be maintained. The current across the resistor is fed to an amplifier which is connected with a PMMC meter used for measurement. 20-Aug-24 85 20-Aug-24 86 The above graph shows the variation of plate voltage and plate current. Now, let’s consider an average reading AC voltmeter using semiconductor diode. In this, the semiconductor diode operated for an alternate half cycle. This is because it operated on the principle of half wave rectifier. 20-Aug-24 87 The average current through the diode can be given by the below equation. The value of 1.11 represents the form factor. The multiplying factor of 2 represents the half wave rectification phenomenon. The average voltmeter can also be designed with the help of full wave rectifier. The average current in case of full wave rectifier will be double to that of half wave rectifier. 20-Aug-24 88 20-Aug-24 89 ADVANTAGES OF AVERAGE READING AC VOLTMETER Low Power Consumption High input impedance Simple Construction Uniform scaling 20-Aug-24 90 DISADVANTAGES OF AVERAGE READING AC VOLTMETER The circuit under measurement should possess low resistance otherwise it will load the circuit. The linear characteristics should be strictly maintained because non- linear characteristics will create issue during measurement of low voltages. It is appropriate only in audio-frequency range because, in radio frequency range, errors occur due to distributed capacitance. 20-Aug-24 91 PEAK READING AC VOLTMETER The peak reading voltmeter can also be designed in two ways. One is by using vacuum tube and another with the help of semiconductor diode. The difference between the peak reading and average reading circuit arrangement is only that the capacitor is not used in case of average reading while it is used in case of peak reading voltmeter. 20-Aug-24 92 The above circuit shows the AC voltmeter using vacuum tube. The capacitor is connected in parallel to the resistance of high magnitude. The resistance is connected in parallel to the amplifier and the PMMC meter. In the same way, it can be designed with the help of semiconductor diode. In place of the vacuum tube, we need to connect semiconductor diode in this case. 20-Aug-24 93 The working of the peak reading voltmeter can be easily understood with the help of above two circuits. When the input voltage is applied the capacitor starts charging until it attains its peak value. When the capacitor attains peak voltage, it starts getting discharged through the resistor. 20-Aug-24 94 The main disadvantage of using peak reading AC voltmeter is that it is not suitable for the measurement of a low voltage signal. This is because, in case of the low input voltage, the electrons at high velocity still contribute to current flow in the circuit which produces errors in the measurement. 20-Aug-24 95 TRUE RMS READING AC VOLTMETER The average reading voltmeter is economical and provides RMS value of sinusoidal waveforms then what is the need for true RMS reading AC voltmeters? And that too when the cost of true RMS reading voltmeter is higher than that of average reading AC voltmeters. This is because in case of non-sinusoidal waveforms we need to calculate true RMS voltage which is not possible with the help of average reading AC voltmeter. In such case, we need true RMS reading voltmeter. 20-Aug-24 96 20-Aug-24 97 PRINCIPLE OF TRUE RMS READING AC VOLTMETER The heating power associated with the waveform varies directly with the square of the RMS value of the voltage. Thus, if by some means we succeed in measuring the heating power of the waveform we can easily measure the RMS value associated with the input waveforms. For this purpose, a thermocouple is utilized. The thermocouple is an instrument which is used for measuring heating power of waveforms. 20-Aug-24 98 WORKING The thermocouple used here will fetch input voltage from AC amplifier. This is because the magnitude of the signal should be sufficiently high in order to provide correct measurements. The thermocouple will receive the input waveforms through its input terminal. This thermocouple is called main thermocouple. The non-linearity associated with the main thermocouple needs to cancel out. Otherwise, it will create errors in the final measurements. For this purpose, the balancing thermocouple is used. The non- linearity produced by balancing thermocouple and the non-linearity produced by the main thermocouple cancel out each other. 20-Aug-24 99 In this way, it acts as a balancing bridge circuit. The feedback current supplied by the AC amplifier is used for balancing in case of any error signal produced. When the input is not applied to the main thermocouple, then the output of both thermocouples will be equal and will produce zero voltage signal. Thus, true RMS reading AC voltmeter provides an aid to measure the RMS value of the non-sinusoidal waveform. It must be kept in mind that dynamic range of AC amplifier should be sufficiently high so that peak excursion created by input waveform does not exceed the range of AC amplifier. 20-Aug-24 100 AC CURRENT METER / RF METER / THERMOCOUPLE Pair of dissimilar metals and at junction we give heat effect, then it generates DC voltages and is connected to ammeter to measure high currents. A thermocouple consists of a junction of two dissimilar wires, so chosen in such a way that a voltage is generated by heating the junction. The output of the thermocouple is delivered to a sensitive DC micro ammeter. Thermocouple are the standard means for measuring current at radio frequencies. Hence named as RF ammeter. The generation of DC voltage by heating the junction is called thermos electric action and the device is called thermocouple. 20-Aug-24 101 TYPES OF THERMOCOUPLE In a thermocouple instrument, the current to be measured is used to heat the junction of two metals. These two metals form a thermocouple and they have the property that when the junction is heated it produces a voltage proportional to the heating effect. This output voltage drives a sensitive DC meter i.e, DC ammeter giving a reading proportional to the magnitude of AC input. Various types of thermocouples are as follows: 1. Mutual type thermocouple 2. Contact type thermocouple 3. Separate heater type thermocouple 4. Bridge type thermocouple 20-Aug-24 102 MUTUAL TYPE THERMOCOUPLE In this type, the AC current passes through the thermocouple itself and not through a heating wire. The meter shunts the thermocouple , these are the disadvantages in it. 20-Aug-24 103 CONTACT TYPE THERMOCOUPLE This is less sensitive than mutual type. In this type there are separate thermocouple leads which conduct away the heat from the heater wires. 20-Aug-24 104 SEPARATE HEATER TYPE THERMOCOUPLE In this type the thermocouple is hold near the heater but insulated from it by the glass bead as shown in figure. This makes the instrument sluggish and also less sensitive because of the temperature drop in the glass bead. To avoid loss of heat by radiation, the thermocouple arrangement is placed in vacuum to increase its sensitivity. 20-Aug-24 105 BRIDGE TYPE THERMOCOUPLE This type has high sensitivity compared to mutual type and avoids the shunting effect on the micro ammeter as shown in figure below. The sensitivity is increased by placing it in vacuum since loss of heat by conduction is avoided and absence of oxygen permits operation at much higher temperatures. 20-Aug-24 106 LIMITATIONS OF THERMOCOUPLE Heaters can stand only for small overload. A rise in temperature causes a change in the resistance of heater. Presence of harmonics change meter readings, because the heating effect is proportional to the square of the current. 20-Aug-24 107 OHMMETER (SERIES TYPE OHMMETER) A D’Arsonval movement is connected in series with a resistance R1 and a battery which is connected to a pair of terminals A and B. The unknown resistance is connected across A and B. This forms the basic type of series ohmmeter. The current flowing through the movement then depends on the magnitude of the unknown resistance. Therefore, the meter deflection is directly proportional to the value of the unknown resistance. R1 = current limiting resistance R2 = zero adjust resistance V = battery Rm = meter resistance Rx = unknown resistance 20-Aug-24 108 CALIBRATION OF THE SERIES TYPE OHMMETER To mark the “0” reading on the scale, the terminals A and B are shorted, i.e. the unknown resistance Rx = 0, maximum current flows in the circuit and the shunt resistance R2 is adjusted until the movement indicates full scale current (Ifsd). The position of the pointer on the scale is then marked “0” ohms. Similarly, to mark the “∞” reading on the scale, terminals A and B are open, i.e. the unknown resistance Rx = ∞, no current flow in the circuit and there is no deflection of the pointer. The position of the pointer on the scale, is then marked as “∞” ohms. By connecting different known values of the unknown resistance to terminals A and B, intermediate markings can be done on the scale. 109 The accuracy of the instrument can be checked by measuring different values of standard resistance, i.e. the tolerance of the calibrated resistance, and noting the readings. Therefore, in a series ohmmeter the scale marking on the dial, has “0” on the right side, corresponding to full scale deflection current, and “∞” on the left side. 20-Aug-24 110 CALIBRATION OF R1 AND R2 The values of R1 and R2 can be determined from the value of Rx which gives half the full scale deflection. where Rh = half of full scale deflection resistance. The total resistance presented to the battery then equals 2Rh and the battery current needed to supply half scale deflection is Ih = V/2 Rh. To produce full scale current, the battery current must be doubled. Therefore, the total current of the circuit, It = V/Rh The shunt current through R2 is given by I2 = It– Ifsd The voltage across shunt, Vsh, is equal to the voltage across the meter. 20-Aug-24 111 20-Aug-24 112 20-Aug-24 113 20-Aug-24 114 20-Aug-24 115 20-Aug-24 116 MULTIRANGE OHMMETER For wide range of resistance measurement, the range is to be extended called a multi range ohmmeter. 20-Aug-24 117 SHUNT TYPE OHMMETER Consists of a battery in series with an adjustable resistor R1, and a D’Arsonval movement. The unknown resistance is connected in parallel with the meter, across the terminals A and B, hence the name shunt type ohmmeter. In this circuit it is necessary to have an ON/OFF switch to disconnect the battery from the circuit when the instrument is not used. 20-Aug-24 118 CALIBRATION OF THE SHUNT TYPE OHMMETER To mark the “0” ohms reading on the scale, terminals A and B are shorted, i.e. the unknown resistance Rx = 0, and the current through the meter movement is zero, since it is bypassed by the short-circuit. This pointer position is marked as “0” ohms. Similarly, to mark “∞” on the scale, the terminals A and B are opened, i.e. Rx = ∞, and full current flows through the meter movement; by appropriate selection of the value of R1, the pointer can be made to read full scale deflection current. This position of the pointer is marked “∞” ohms. Intermediate marking can be done by connecting known values of standard resistors to the terminals A and B. 20-Aug-24 119 This ohmmeter therefore has a zero mark at the left side of the scale and an ∞ mark at the right side of the scale, corresponding to full scale deflection current. 20-Aug-24 120 MULTIMETER What is a Multimeter? A Device which consists of an ammeter, voltmeter and ohmmeter combined, with a function switch to connect the appropriate circuit to the D’Arsonval movement. 20-Aug-24 121 DC VOLTAGE MEASUREMENT The part of the circuit diagram of Multimeter, which can be used to measure DC voltage is shown in below figure. 20-Aug-24 122 The above circuit looks like a multi range DC voltmeter. The combination of a resistor in series with PMMC galvanometer is a DC voltmeter. So, it can be used to measure DC voltages up to certain value. We can increase the range of DC voltages that can be measured with the same DC voltmeter by increasing the resistance value. The equivalent resistance value increases, when we connect the resistors are in series. In above circuit, we can measure the DC voltages up to 2.5V by using the combination of resistor, R5 in series with PMMC galvanometer. By connecting a resistor, R4 in series with the previous circuit, we can measure the DC voltages up to 10V. In this way, we can increase the range of DC voltages, simply by connecting a resistor in series with the previous (earlier) circuit. We can measure the DC voltage across any two points of an electric circuit, by connecting the switch, S to the desired voltage range. 20-Aug-24 123 DC CURRENT MEASUREMENT The part of the circuit diagram of Multimeter, which can be used to measure DC current is shown in below figure. 20-Aug-24 124 The above circuit looks like a multi range DC ammeter. the combination of a resistor in parallel with PMMC galvanometer is a DC ammeter. So, it can be used to measure DC currents up to certain value. We can get different ranges of DC currents measured with the same DC ammeter by placing the resistors in parallel with previous resistor. In above circuit, the resistor, R1is connected in series with the PMMC galvanometer in order to prevent the meter gets damaged due to large current. We can measure the DC current that is flowing through any two points of an electric circuit, by connecting the switch, S to the desired current range. 20-Aug-24 125 AC VOLTAGE MEASUREMENT The part of the circuit diagram of Multimeter, which can be used to measure AC voltage is shown in below figure. 20-Aug-24 126 The above circuit looks like a multi range AC voltmeter. We know that, we will get AC voltmeter just by placing rectifier in series (cascade) with DC voltmeter. The above circuit was created just by placing the diodes combination and resistor, R6 in between resistor, R5 and PMMC galvanometer. We can measure the AC voltage across any two points of an electric circuit, by connecting the switch, S to the desired voltage range. 20-Aug-24 127 RESISTANCE MEASUREMENT The part of the circuit diagram of Multimeter, which can be used to measure resistance is shown in below figure. 20-Aug-24 128 We have to do the following two tasks before taking any measurement. Short circuit the instrument. Vary the zero adjust control until the meter shows full scale current. That means, meter indicates zero resistance value. Now, the above circuit behaves as shunt ohmmeter and has the scale multiplication of 1, i.e. 100. We can also consider higher order powers of 10 as the scale multiplications for measuring high resistances. 20-Aug-24 129 MULTIMETER OPERATING INSTRUCTIONS ❑ To prevent meter overloading and possible damage when checking voltage or current, start with the highest range of the instrument and move down the range successively. ❑ For higher accuracy, the range selected should be such that deflection falls in the upper half on the meter scale. ❑ For maximum accuracy and minimum loading, choose a voltmeter range such that the total voltmeter resistance is at least 100 times the resistance of the circuit under test. ❑ Make all resistance readings in the uncrowded portion on the meter scale, whenever possible 20-Aug-24 130 MULTIMETER OPERATING INSTRUCTIONS ❑ Take extra precautions when checking high voltages and checking current in high voltage circuits. ❑ Verify the circuit polarity before making a test, particularly when measuring dc current or voltages ❑ When checking resistance in circuits, be sure power to the circuit is switched off, otherwise the voltage across the resistance may damage the meter. ❑ Renew ohmmeter batteries frequently to insure accuracy of the resistance scale. ❑ Recalibrate the instrument at frequent intervals. ❑ Protect the instrument from dust, moisture, fumes and heat. 20-Aug-24 131 METER PROTECTION When we measure an electrical signal there very often may be a chance of over current through the meter. This may be due to following reasons. The meter may be connected wrongly to the circuit. The rating of the meter is selected wrongly for the measurement. Occurrences of over current in the circuit itself during measurement. Over current causes overheating in the meter which may ultimately lead to a permanent damage to the meter. The reasons of the over current cannot be avoided 100% although it is convenient to protect the meter from the effect of over current. This is done by using semiconductor diode of suitable rating. Whenever a meter is connected in the circuit to measure an electrical signal, there must be a voltage drop across it. If the current through the meter is increased beyond safety limit, the voltage drop also crosses the rated limit. 20-Aug-24 132 Suppose the rated voltage drop limit of the meter is 0.6 volt. Now, let us connect a diode across the meter, whose forward barrier voltage is 0.6 volt. Now, if due to excess current through the meter, if the voltage drop across the meter becomes more than 0.6 volt, the diode becomes short- circuited, since this excess voltage also appears across the diode. As soon as the diode is short-circuited, the meter current is diverted through the diode. As result, the meter is being protected from excess heating. If only one diode is used it is called Single Diode Protection. 20-Aug-24 133 If two diodes are connected across the meter in opposite direction, it is called double diode protection. This arrangement protects the meter for both directions of current. 20-Aug-24 134 EXTENSION OF RANGE 20-Aug-24 135 EXTENSION OF THE RANGE OF AMMETER The range of ammeter can be extended using shunt resistance parallel with coil. 20-Aug-24 136 The ratio of total Current to be measured to The full scale Deflection current is called as the multiplying power of the shunt. it may be denoted by N. 20-Aug-24 137 EXTENSION OF THE RANGE OF VOLTMETER The range of Voltmeter can be extended using Multiplier (a high resistance ) Series with coil. 20-Aug-24 138 The ratio of total Voltage to be measured to the voltage across the voltmeter for which it is actually designed is known as multiplying factor. it may be denoted by m. 20-Aug-24 139 Hence for the measurement of voltage m times the voltage range of instrument ,the series multiplying resistance R should be (m – 1 ) times the meter resistance Rm. 20-Aug-24 140 SPECIFICATIONS OF INSTRUMENTS 20-Aug-24 141 20-Aug-24 142 20-Aug-24 143 20-Aug-24 144