Embryology PDF - Medical Embryology
Document Details
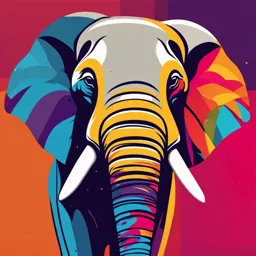
Uploaded by CheaperHummingbird
2022
Enrico Pierantozzi
Tags
Summary
This document presents an overview of embryology, focusing on the microenvironment of the reproductive system and the growth of the zygote. It discusses ethical considerations, the impact of birth defects, and the historical development of the field. The importance of embryonic stem cells and animal models in understanding human development are also highlighted.
Full Transcript
MICROENVIRONMENT OF REPRODUCTIVE SYSTEM - PIERANTOZZI ENRICO HISTOLOGY AND STEM CELL IN REPRODUCTION Textbook: Medical Embryology, T.W. Sadler...
MICROENVIRONMENT OF REPRODUCTIVE SYSTEM - PIERANTOZZI ENRICO HISTOLOGY AND STEM CELL IN REPRODUCTION Textbook: Medical Embryology, T.W. Sadler Exam: true or false; histological images to recognise 1/12/2022 GROWTH OF ZYGOTE Initially we focus on the first two weeks of development which represent a particular type of morphogenesis growth and differentiation. The first two weeks also have a lot of implication for what concerned the ethical aspect of the study of embryology. Why ethical aspect? Because what we actually know about embryology comes from studies that began during the period of the ancient Greeks, so the ancient theory about embryo development it was postulated also by Aristotle. The very moment in which embryology starts to be a real biological discipline began in the moment in which some kind of microscope could be available (middle of 17th century). Starting from here, there were a lot of theories and some of these are also quite funny, but they are quite funny now for us: without the possibility to see what happens into the embryo, it was impossible to postulate what really happens during embryo development. WHY EMBRYOLOGY? It's an important development. – birth defects are the leading cause of infant mortality – birth defects are a major contributor to morbidity, including physical and mental handicaps – all women of childbearing age (= età fertile) are at risk of having an infant with a birth defect. The incidence rate is 6/100 (six out of hundred) births. – each of you will have contact with women of childbearing age; either as a friend as a companion or as a patient. There are different aspects concerning the knowledge about the events that characterize embryo development that have a lot of application for our social life, in particular the main aspect is the incoming of birth defects. If also a little thing goes wrong, the final effect could lead to a birth defect. Some kinds of birth defects are malformation and deformation: Malformation -> the results of a process that goes wrong when a specific cell is not properly specified during its differentiation. Baby born without an entire hand. Deformation -> the results of a process in which a differentiated cell or a differentiated group of cells that constitute a tissue/organ encounter some kind of problem in the organogenesis, in the final morphogenesis. Baby born with only four fingers. Birth defects have a big incidence rate: 6 out of 100 births. It is not so low; there is a part of these that have birth defects that don’t create so many problems, but there are some kind of birth defects that could severely affect the quality of the life of the individual. Knowing which are the mechanisms that altered this aspect is obviously really important. Over the years we have acquired information that enbase the entire period of embryo development within well focused molecular and morphogenic aspects and now we know a lot of stresses which can outbreak these types of progressive development, and we know these kinds of stresses altered these mechanisms because over the years we have been able to understand which where those work. In fact, the problems of those individuals with birth defects have a great incidence also in many other aspects that are not directly limited to embryology and one of those is the costs to support the life of individual born with birth defects. We have to keep in mind that the embryo knows very well when it can proceed with its proper development and when it’s the case to stop it (that results in abortion): if the kind of birth defect has a great impact on the embryo development the further development stops and obviously the embryo, and subsequentially fetus, dies. But there are some kinds of birth defects that don’t affect the ability of the embryo to complete his development, and it results in birth defects that can affect the quality of life of the individuals which carry them, but also of his family. With the knowledges about embryology, we can prevent many of those birth defects because they are cause to specific chemicals, or alcohol, or a particular environment, … Obviously when birth defects depend on a genetical alteration we cannot fight against that. But if we know which are those kinds of genes that if mutated results in birth defects, we can predict what could happens to that embryo during its development. Divorce rate is higher among those couples that have children with birth defects than couples that have “normal” children. The social impact that birth defects may have is often underestimated. Most women do not know they are pregnant until the second month of pregnancy (before the 8 week of embryo development → that is the most sensitive period for an embryo). The embryonic period start from week 3. In this period the embryo is more sensitive to any kind of external signals. It's also the period where the embryo starts the differentiation. The first two weeks of embryo development are not so sensitive to external factors because this is the period during which the embryo increases in number of cells without any clear differentiation between the cells that compose it. This is not completely true: also, during the first two week of development some kinds of inner differentiation occurs but during the first two weeks the group of cells that will form specific structures are induced but those cells, although they’re induced to work as specific differentiation fate, do not acquired a mature differentiation stage. This period of development is not so dangerous in terms of the production of birth defects. MAIN STEP OF EMBRYOLOGY HISTORY – Middle of 17th century → Microscopes allowed to individuate some “elements” (spermatozoon) inside seminal fluid. In that period, it was impossible for women to see and in facts initially they thought that the merge between this seminal fluid containing these elements with menstrual blood could be the reason why the babies born. Subsequently with the improvement of microscopes, it was also possible to explore analysis on fetuses aborted or exploring animals like cows and rabbits. Due to this, it was quite clear that elements similar to those that have been observed in animals’ fluids were also in a particular structure within female that originally were called “female testis” (just because they were quite rounded similar to testis that could be obviously invisible). – Mid of 19th century → cell's theory: scientists start thinking the merge between a male specific cell with a female specific cell was the very first moment that characterize the formation of new life. – All the information we now know from a molecular point of view have been acquired during the lasts 15 years, essentially with the description of DNA, of the functions of the gene and the merge between molecular biology and histological observation represents the moment in which the study of the mechanisms of embryo development rises up. – During the 1850/1960 many genes have been characterized for the functioning; so mechanisms that drive the embryo development start to be clear. – 1981 represents a sort of spike for embryo research → the embryonic stem cells (= undifferentiated cell of a multicellular organism which is capable of giving rise to indefinitely more cells of the same type and from which certain other kinds of cell originate by differentiation) have been identified by Evans. Initially the identification was make in mouse and only about 20 years later they were also isolated from human embryo. These kinds of cells can be obtained from a very early stage of embryo development. They possess specific characteristics: if a single embryonic stem cell is transplanted in another developing embryo, this single cell coming from another individual is included within the developing embryo and it takes part to the formation of specific region of the embryo that develops properly without any difficulties. This implies that if I modify a single embryonic stem cell in term of gene expression (for example knocking-out a specific gene) I can follow which part of the embryo development is affected by the absence of that specific gene. This gives the possibility to associate the role of gene in specific moment of development. At the beginning of this millennium, the entire human genome and the entire mouse genome were sequenced → possibility to authored the expression of whatever gene we want. Exploring these characteristics of the embryonic stem cells, the role of thousands genes was described. Most of molecular aspects have been identify thanks to this discovery (in vitro stem cells → that allowed to discovery the function of all the genes, essentially during the embryo development which is the moment of any kind of development that in mammal is really hard to follow). The use of embryonic stem cells has been explored in animal models, like mouse. This because many morphogenetical and molecular events, that occur during mouse embryo development, are really similar to those events that occur during human embryo development. In facts we can’t distinguish the human embryo from animal ones because they are very similar. This is just because the very first moments of any metazoan embryo development and differentiation are really similar, because of an evolutionary point of view → this is the better way to achieve the production of a new life. On those pictures we can see the embryo of 6 different animals th that correspond to the 28 day of human development. The very first event from the zygote to the blastocyst are really similar between mouse and human, indicated that this moment of development is fundamental for the development of every kind of animal. In facts, if we go further in differentiation, we can now exclude some embryo for being human: A and B probably are not a human embryo, but C D E F (in a period of development that correspond nd to the end 2 month of human embryo development) are quite similar between these 4 animals. Finally, we can recognize at the end which is the human embryo (F). Animal models have represented actually one of the best ways to study embryo development. For what concerned human development, many notions comes from mouse and essentially the th notions we have concerning human embryo development starting from the 15 day of development. HOW TO STUDY EMBRYOLOGY? – morphological examination (optical microscope) → from aborted fetuses (in vitro), but also in vivo (from mouse) – improved imaging techniques Now we have a lot of tools to follow better morphogenesis of the embryo and in the case of the human we can study it only starting from aborted fetuses, but if we study the embryo development in a mouse line, we are allowed to perform also in vivo treatment that can alter this kind of development to allow us to understand how this development takes place. Many morphogenetic and molecular aspects are similar between mice and humans → a lot of information that now we have come from study on mouse. Embryonic stem cells have improved our knowledge in the study of embryology, they have been / are so important: genetical modifying these cells and transplant them into an healthy embryo, make them included and forming the normal differentiation of the embryo; but the progeny of that specific cells will unlock a specific gene so we can understand which is the role of that specific gene. No more than 10 years ago, with also the improvement of culture techniques, some scientists have been able to induced the differentiation of a single healthy embryonic stem cell isolated from mouse. When they found a proper culture environment, they observed that in some cases the embryonic stem cells start to spontaneously differentiate and the progenitor, this single cell, forms a structure that mirrors quite exactly the physiological early steps of development of normal mouse embryo. In the picture, on the left, we can see the morphology acquired by a 4-day old mouse embryo derived from embryonic stem cells compared to a healthy mouse embryo that develops normally from the zygote. The reciprocal rearrangement of the progeny of the embryonic stem cells parallels the development of the normal embryo and this has been a tool that allow the embryologist to define many other additional molecular and morphogenetical events that characterize the embryo development. This can be translated to the human embryo development. For what concerned human embryo development, we have to keep in mind 2 main things: the typical structure of every metazoan differentiation is the BLASTOCYST th that in human forms around the 6 day of development. In the mouse the rd blastocyst forms around the 3 day of development, so these structure, that are practically the same in terms of morphology, in human forms after six days and we have a gestational period of 38 weeks while in mouse forms following three days with a gestational period of 20 days. the GASTRULATION occurs at the end of the 2nd week of embryo development in humans th and the 14 day is really important in term of ethics and human embryo study. In facts the th law prohibits to culture in vitro human embryo behind the 14 day of development because at the beginning of the third week of development, gastrulation starts occurring. The gastrulation is the morphogenetical event that transforms the bi-laminar embryo into trilaminar embryo, giving rise to the three germ layers that will form any parts of the embryo. Actually we are able to follow in vitro morphogenesis events that occur in human embryo but only th until the 14 day and also is prohibited by the law to modify the embryo: the only thing that you can do is just picking a cell if you want to perform a genetical analysis of that embryo, just to be sure that it doesn’t carry any kind of mutation and it is an healthy embryo. When the blastocyst is formed, in the practice of assisted reproductive techniques, the human blastocyst fertilized in vitro is left to develops till the stage of blastocyst and then it is re-implanted in the mouse. In some cases, not in Italy, you can culture a human embryo for scientific purposes and so study what happens but the fourteenth day represents worldwide the moment in which the embryo must be killed and cannot do further culture in vitro. The structure of the embryo that is going to gastrulate is quite different between human and mouse, so from a morphogenetic point of view there is not a grade overlapping between mouse and human for what concerned the morphogenesis of the moments after blastocyst formation. But the molecular aspects are really similar: all the mechanisms involved to drive gastrulation are really similar between mouse and human, in spite of the differences in morphology. The very first steps of development till the formation of the blastocyst are really similar in human and mouse. So what we are going to see about the development of zygote to go at the stage of blastocyst, all these information are taken either studying mice development and either studying human development during last fifteen years. In contrast, following the implantation you can see how the structures are different although the general mechanism is similar. TIME ASPECTS OF HUMAN EMBRYO DEVELOPMENT: Pregnancy: 38 weeks (266 days) from fertilization When a pregnant woman goes to the gynaecologist, he says that she’s pregnant from 2/3/4/… weeks considering her Last Normal Menstrual Period (LNMP). But now we know that the ovulation in female occurs in average 14 days after the end of menstrual flow. The 1 day of fertilization is the moment in which sperm enters the oocyte → formation of zygote morula → 2 - 3 days the formation of blastocyst → 4 - 5 days implantation → 6 - 8 days gastrulation → 14 - 28 days (when the embryo is fully implanted) – First and second month (1 to 8 weeks) = EMBRYONIC PERIOD At the end of 2 months, the heart is formed in every single part / completed. – Third to 9th month = FETAL PERIOD: 3rd - 6th month = non-living fetal period 6th - 9th month = living fetal period How calculate embryonic age? Most scientists calculate the age as gestational or menstrual age and use the LNMP (last normal menstrual period) as the starting point, supposing that fertilization occurs at the midpoint of the cycle. Therefore, converting fertilization age to LNMP age requires adding 2 weeks. The day 1 of a new embryo is the formation of the zygote, that is the moment of the fertilization. The most important step after the fertilization is the formation of blastocyst that occurs around the th 5 day of embryo development. The blastocyst is the morphological form of the embryo that will implants → it means that blastocyst docks to the endometrium and starts growing to enter the mother tissue (endometrium). At the end of implantation, the entire blastocyst will be enveloped within the endometrium. Another fundamental moment for embryo development is gastrulation, and it occurs in every single embryo starting from the beginning of the 3rd week of development: when the embryo is fully th th implanted, the gastrulation starts occurring and it goes from 14 day to the 28 day of embryo development. At the end of the first month of development the gastrulation is over and the gastrulation stops when the neural tube is completely closed, it means caudal part and cranial part. Embryonic period and fetal period: Why there is a differentiation between embryonic period and fetal period? Why the first two months are defined as the embryonic period of the development? This runs in parallel with heart development: at the end of the second month the heart is formed in every single part (it has only to grow starting from the end of the second month), from an anatomical point of view. THE FIRST WEEK of development The fertilization occurs in the upper section of the tube, in the region called ampulla. Soon th after fertilization the embryo starts travelling towards the uterus where at the 6 day of development it will implants. The implantation will occur also thanks to specific histological differentiation of the endometrium where there is a higher progesterone and estrogens stimulation in order to make a series of modification that prepares the endometrium to receive the implanting embryo. While travelling along the tube to go to the uterus, we can see that the embryo divides and it’s important to know how the very first division starts occurring usually 24 hours later the fertilization (point 4). In facts during the very first day the zygote is composed by 2 pronuclei that are still not fused → once they fused than the embryo starts his first mitotic division. During his travel along the tube, we can see that 2 cells are produced, then 4 cells, 8, 16 and so on. When the embryo arrives in the uterus, it's in a blastocyst stage and then implants. If something goes wrong during these mitotic divisions or during blastocyst’s formation usually the embryo dies: if the embryo fails the fully implants, he dies. nd Once implanted within the endometrium at the end 2 week of development, the embryo starts to gastrulate. Histological aspects: th when the ovulation occurs, in average at 14 day of the menstrual cycle, the formation of corpus luteum determines the production of progesterone which is the hormone that drives the thickness (spessore) of endometrium. At the end of the menstrual phase, it’s called basal layer, indicated on the images like SB (stratum basale). The basal layer of endometrium is formed by an epidermal layer and underneath connective tissue. Following the ovulation and the increased levels of progesterone, basal layer starts to support the formation of the functional layer, called SF (stratum functionalis) that increases in thickness and starts to be full of glands that produce mucus, this is an important aspect for the endometrium to prepare itself to receive the embryo. th Around the 25 day of the menstrual cycle, the functional layer is full of glands and lacunaes filled by mucus. This is the moment in which, if the embryo is present, he is able to dock the functional layer and starts to digest its mother tissue to be received within the endometrium. If the fertilization doesn't occur, the lifespan (durata della vita) of corpus luteum is about 2 weeks, so at the end he starts to degenerate and no longer produces progesterone. Without th progesterone the functional layer starts degenerating and it is displaced at the end of the 28 day of menstrual cycle. This is the reason why the menstrual phase produces blood and other material that correspond to displaced functional layer. If fertilization occurs, the implanting embryo once implanted, starts itself to produce progesterone to support the maintenance of the functional layer. The presence of HCG (Human Chorionic Gonadotropin), produced both by the embryo and by the endometrium, supports the life of the corpus luteum that continues to produce progesterone, to support the blood irroration and the maintenance of the endometrium. This is the main hormone regulation in the case of fertilization. THE STAGE OF EMBRYO DIVISION (from a morphogenetic point of view): During the 1 week of development (= from fertilization to implantation): 1. fertilized egg 2. 4-cell embryo 3. 8-cell embryo and 8-cell embryo compacted/morula 4. blastocyst 5. hatching blastocyst → ready to implant In the first photo there is the zygote; when the pronuclei are fused, the zygote is formed. The embryo starts to divide forming 4-cell embryo, then 8-cell embryo and so on till the stage of blastocyst (more than 100 cells, around 137 cells). The dimension / volume is basically the same between 4-cell embryo, 8-cell embryo and blastocyst. The mitotic divisions, that leads to the formation of the blastocyst, are called SEGMENTATION just because the cells produced by a single mitotic event are in general smaller than the cell from which they arrived. There is a sort of membrane that incapsulates the embryo, called zona pellucida → during the th 5 day of development is maintained around the embryo to compress and impede it to increase in dimension. In the photo number 5, we can see that the external cells of the blastocyst digest zona pellucida and the blastocyst finally hatches and freely flows within the uterus. The blastocyst is now already to implants. BLASTOMERES: each of the cells that result from the cleavage of the fertilized egg. The image on the left shows an 8-cells stage embryo and it is easy to identify the single 8 cells. Once the embryo reaches the 8-cells stage, the embryo compacts. The event of compaction is considered the very first moment of embryo development in which a kind of differentiation between the cells composed the embryo occurs. In facts at the 8-cells stage before compaction, every single cell of the embryo has the same potency (each of these cell is TOTIPOTENT): it means that if I pick a single cell from a non-compacted embryo at the 8-cells stage (or at previous stages), that single cell is able to produce a “complete embryo” (= all the parts that compose the embryo properly and the embryonical annexes, which are chorion, yolk sac and amniotic cavity. These 3, together with the maternal part of the tissue, will form the placenta). (When we approach to embryology, we have to keep in mind that embryo development is probably the most dynamic environment that we ever had studied. In a specific time window, all the things we will going to talk about occur concurrently and that thing that normally occurs at the second day, if it occurs in the very same manner at the fifth day, it will have a completely different effect.) While compaction occurs, the embryo is still proliferating and this means that the space between the cells, that allow us to identify singularly those cells, disappeared when embryo compacts. But as the embryo is proliferating, it means that some cells will be enclosed within the embryo, they will not have any further contact with the external environment. This means that these cells are exposed to the tube environment and then to the uterus environment, and it’s a different environment from the environment in which are exposed the cells enclosed within the developing embryo. This is really important because the external cells sense some chemical aspects or for example the pH that there are in the uterus environment and which is different from chemical environment or the pH that are experienced by cells that are not in direct contact with the uterus environment. Compaction’s events depend on the modulation of those kind of cellular adhesion structures that characterize every single cells. In the image on the right there is a summary of the cellular adhesion structures. KINDS OF ADHESION AND INTERCELLULAR CONTACTS: ZONULA OCCLUDENS (TIGHT JUNCTION) Tight junctions are structures that strictly make adhere two joining cells. They are a kind of cell junction that impede the paracellular flow. The chemical environment is different between two sides of the cells layer and these cells select through their plasma membrane which kind of molecules can enter the cells and can be exposed to the inner part of the cells. In other words, when the blastocyst forms, we can delineate 2 kinds of cells: the external cells (a kind of layer that determines the border of the embryo) → they are full of tight junctions! They select which kind of molecules can be transported from the uterus to the inner part of the embryo and what kind of molecules does interact with inner cells of the embryo (that are not in direct contact with the uterus environment). the group of inner cells (that are separated from the external environment). The very first moments of compaction are determined by the production of tight junctions between all the cells that composed the embryo in that moment; so the embryo lost the visible form of every single cell that composed it. While compaction occurs, also proliferation of the embryo occurs, so inner cells will be segregated from the external environment. The exposition to different environments determines, while the embryo develops, that the inner cells lose the ability to form tight junction and the external cells maintain the ability to form tight junction. At the end of this process, we have a blastocyst in which the external layer is tight adhere, while the inner cells are not tight adhere; it’s just because these two groups of cells experience two different environments that modulate, due to some molecules present in uterus environment, the ability of the cells to maintaining this kind of structure or losing this kind of structure. Obviously the maintenance or the loss of this structure depends on the ability of the cells, through specific signals, to activate the transcription of those genes that are required to form these structures; for example, the main proteins that form tight junctions are occludin and claudine, so those cells that actively transcribes occludin and claudine are cells able to generate tight junction. ZONULA ADHERENS AND DESMOSOMES Depending on the type of junctions, the inner part of every single cell that participate in the formation of junctions (tight junction or zonula adherens or desmosome) is connected with different parts of the cytoskeleton of the cell. For example, tight junction and zonula adherens are connected with actin filaments, which usually reside underneath the plasma membrane; in contrast desmosomes are in contact with keratin, so the intermedial filaments that compose cytoskeleton. The tight junctions that connect two joining cells are important to discriminate between environments to which cells are exposed. Is also important to drive a collective movement of some cells that are connecting through desmosomes or zonula adherens. HEMIDSMOSOMES and FOCAL ADHESIONS There are additional types of junctions, here represented by focal adhesions (that are in connection with the actin cell’s cytoskeleton) and the hemidesmosomes (that are very similar to desmosomes and are connected with keratin filaments). The peculiarity of focal adhesions and hemidesmosomes is that usually are located in the basal pole of the cells, in direct contact with the extracellular matrix (ECM) and the extracellular matrix during the embryo development has a great important because it represents the roots on which the cell can move. In facts the embryo development relies on continuous cell movement, because every cell of the embryo needs reach its proper position where it must differentiate to work to the specific structure that it is supposed to form. This is true during very early stage of development and it is true and more comprehensible starting from gastrulation which is a moment during which all the cells of the embryo start to move one respect to the others, in order to reach their proper position and they move exploring extracellular matrix environment; they can move if the contact with the extracellular molecules allow the cells to move to determine those actine rearrangements that are required for the migration of the cells (= one of the movements of the cells) → one of the main aspects of first week of embryo development, just because all the cell must reach their proper and correct position in which they have to differentiate into the structure they are supposed to form. GAP JUNCTIONS Gap junctions put in connection the cytoplasm of 2 joining cells. The pore for gap junctions can be travelled only by ions (suggest calcium that is one of the main second messenger in cell signaling) or by very small peptides. Essentially gap junctions allow a series of cells to experience the very same cytoplasmic environment. The ability to generate or not gap junctions account for a determined group of cells to respond all together to those signaling. BLASTOCYST FORMATION Right after compaction, the embryo is called MORULA → a sort of a ball full of cells (some are in the external layer of the embryo, while some others are included within the embryo). Once the embryo has compacted, all the compacted cells start to product a fluid (essentially composed by water and some inorganic ions) that starts to accumulates between the space of those inner cells that have lost the ability to create tight junctions. Fluid is pumped toward the inside and begins to displace the inner cells; so the fluid remains within the embryo and it starts accumulate it between inner cells' spaces. In fact, intracellular spaces are rapidly filled by fluid produced by trophoblast; when the inner cellular spaces merged together and are filled with this fluid, a sort of cavity is formed (= blastocele). At this stage, outer cells begin to be committed to form trophoblast (placental) cells, while cells on the inside will form cells for the embryo. The embryo is now called BLASTOCISTI, with 2 distinct cell populations: the inner cells are moved to the upper part / to one pole (= inner cell mass / embryoblast) the external cells form an external layer / the “wall” of blastocisti (= trophoblast / outer cell mass), in direct contact with the uterus. This was the second clear differentiation → the separation of the outer cells from the inner cells is the very consequence of the compaction of the embryo. So trophoblast cells, starting from the formation of blastocyst, will be completely different from the inner cell mass in term of differentiation potential: the structure of the embryo, the amniotic cavity and the yolk sac will be formed starting from the inner cell mass; trophoblast will be responsible for the mechanical implantation of the embryo and it will differentiate toward that part of the embryo, chorion, that will form, together with maternal tissue, the placenta. The cells from an embryo at 8-cells stage is able to differentiate in every kind of cell following the formation of the blastocyst and can give rise to either the cells included within the inner cell mass or included within the trophoblast, but the cells that form the trophoblast display a completely different differentiation potential respect to the cells that compose the inner cell mass. Embryonic stem cells, in vitro, are cells derived from cells of the inner cell mass; this means that embryonic stem cells are cells able to differentiate into every kind of cells that compose our body but embryonic stem cells, that are derived from these blue cells (in the image) so from the inner cell mass, are not able to differentiate toward trophoblast derived cells (such as the cytotrophoblast or the syncitiotrophoblast, cells that will form the chorion). FULL SWITCH TO EMBRYONAL GENETIC CONTROL All the cells of the 8-cell stage are considered totipotent, they are able to differentiate into all the parts of the embryo (embryo proper and all the embryonical annexes). The morula (8-cell stage) formation is before the formation of blastocyst, then all the cells produce the fluid that accumulates in here to determine the formation of the blastocyst and the definitive differentiation between the external layer, the trophoblast, and the inner layer, the inner cell mass. In spite of the presence of the zona pellucida which is essentially composed of fibrous proteins, the trophoblast is in direct contact with the uterus environment, and after the implantation, with the endometrium (maternal cells). In facts tissues derived from the trophoblast will be the tissues that start producing HCG and progesterone together with the endometrium. In contrast, the inner cell mass are not in direct contact with the uterus cells but some of those cells represent the roof of the blastocele and they are in contact with the fluid produced by the cells while some others are not in direct contact with the blastocele; even within the inner cell mass there are some regions that experience some different external environments and this will be really important for further differentiation of cells within the inner cell mass. Molecular mechanisms: In addition to morphogenetic events that determine the initial differentiation, we will see also the main molecular mechanisms that drive the proper embryo differentiation. The 8 cells-stage compaction is the very first morphogenic event that will determine the initial differentiation between embryo's cells → at this stage the embryo began the real controller of its proper development / his proper transcriptional activity. Until this stage the transcriptional activity of the embryo is limited: the cells that compose the embryo and all the proteins that are necessary to the embryo to form mitotic fuse and all the cytoplasmic requirement for segmentation of the embryo are provided by mRNA that are stored in the cytoplasm of the maternal oocyte. It means that the embryo relies on the presence of these kinds of mRNA that, once translated, they produce the protein apparatus that is required for the embryo to segmentate. Starting from the 8-cells stage, every single cell of the embryo starts to control its proper transcriptional activity and one of the main genes that is transcribed following this sort of activation is OCT4 (= a transcriptional factor which is the master-gene of pluripotency in the embryo). In the ovulated oocyte (before fertilization) are stored a lot of mRNAs; when the fertilization occurs the first step of embryo development depends on the presence of these maternal mRNA that are transcribed. In the slide these mRNAs are represented as ESSP1 (= Embryonic Stage Specific Pattern 1) which comprises all the mRNAs that are stored in the maternal cytoplasm → it is calculated that about 1800 genes are transcribed and produce these different transcripts that are used by the embryo during his first step of development. The mRNAs transcript, produced by the embryo, are limited until the 8-cell stage, that occurs around the rd beginning of the 3 day of development. If we implant an embryo in a mother we have to be sure that this embryo is healthy. Several studies recognized three major parameters that collectively predict if the blastocyst, followed in vitro, is healthy, and it is possible based only on the observation of embryo morphology in the stage of blastocyst. 1. In vitro and in vivo, for a normal developing embryo, the duration of the first cytokinesis (= the division of the cytoplasm cell in 2 daughters cells) must be no longer than 15 minutes. 2. The time interval between the end of the first cleavage (= mitotic divisions / segmentations between the zygote stage and the blastocyst stage) and the initiation of the second one must be no longer than 11 hours. Once the first 2 cells are formed, if they don't start cleaving following the subsequent 11 hours, it means that this in vitro-embryo is not healthy and it may have some problems in terms of percentage success of implantation. 3. The beginning of the second cleavage may occurs even in only one of the two cells, so there is not synchronicity: is not required synchronicity respect of the beginning of the second cleavage of the embryo at the 2-cells stage. It could be possible to see a developing embryo composed by three cells just because one of first two has begun the cleavage and the other still not. However, the 4-cell stage must be reached after the complete cleavage of both the cells that form the 2-cells embryo. What does it mean? Looking at the image, it means that if at the stage of 2-cells embryo the cell on the right (called R in the image) is quiescent, the cells on the left (called L in the image) starts to do his first cleavage and produce this 3-cells embryo. If one of the two daughter cells (L1, L2) begins the third cleavage before the quiescent cell R completes its second cleavage, it means the embryo is not considered healthy. The 4-cells stage must be reached following the complete cleavage of the two cells that composed the 2-cells stage embryo. Starting from this point, then the cleavage will not be synchronized but this is not a problem. 2/12/2022 EGA = embryonic genome activation. It means that, at the stage of 8-cell, the embryo starts to produce its own factors to drive his proper development. The embryo development is sustain by maternal transcript during very early stage of development, then from the stage of 8-cell the embryo acquires its own potential and starts transcribing those factors that will drive the initial specific differentiation. The first differentiation starts at the 8-cell stage, in fact the event of compaction distribute, in different relatives position, the cells that in this specific moment compose the embryo. Focusing on the “LATE BLASTOCYST”: we can see 3 type of cells: Trophoblast cells (blue cells) → they will not participate in differentiation / constitution of any part of the embryo; the entire embryo comes from the inner part. Hypoblast (= red cells in direct contact with the fluid / blastocyst cavity) Epiblast (green inner cells) The parts of the embryo that will compose the embryo proper will develop only from the epiblast; in contrast the hypoblast will have a fundamental role in regulating the early phase of gastrulation and will concur to the formation of additional and necessary parts of the entire embryo which are essentially yolk sac and the chorionic plate. When the embryo acquire its proper control of its transcription, we can see a series of genes starts to do the transcribe: specific genes allow the 3 type of cells to acquire the proper characteristics, that will, subsequently, drive the formation of those structures that depends on those cells. Before the embryonic genome activation there is a minor gene activation. This means that, during the stage of 2-cell and 4-cell, the embryo activates some genes but they didn't participate essentially in those mechanism that allow the embryo to reach the 8-cell stage. All the requirements to reach the 8-cell stage are provided by the maternal transcripts that are stolen from the cytoplasm of the oocyte. MATERNAL OCT4 is one of the main transcription factor and it has been found within more than 1 thousand maternal transcripts that have been identified in cytoplasm of the oocyte; so it is transcribed by the oocyte even before the fertilization. Oct4 is one of the 27 proven maternal-effect genes and is regarded to be functionally important for embryonic/zygote genome activation. It is also actively transcribed, starting from the moment in which the embryo acquired its own control of the transcription. Oct4 is the master gene of the early stage of the embryo development! Oct4, a master gene pluripotent, is a transcriptional factor that is actively transcribed by the embryo, from the stage of zygote to the stage of blastocyst (in mice and humans oocytes). When the blastocyst starts implanting and then gastrulate, the transcriptional OCT4 falls down. When the cells of the embryo start to take their way of differentiation, this transcriptional factor is inhibited (= is no longer transcribed), except for what concern the primordial germ cells (those cells, depending on the sex of the embryo, that will differentiate to spermatozoa or oocyte). In fact, in the adult ovary, where oocyte reside, there is an intense transcriptional activity of this transcription factor; so the oocyte, also during his maturation, keeps maintain the ability to transcribe Oct4. For what concern the time of development of a mouse embryo, “ZGA” is referred to the fact that the activation of the transcription in the embryo in mice occurs soon after the fertilization; this is different from what occurs in the development of human embryo. The embryonic genome activation in mice occurs earlier, but the effect of this activation are quite the same between mouse and human embryo. The transcription factor Oct4 regulates the expression of additional transcription factors that are required to maintain the undifferentiated state of those cells that compose the blastocyst. Those cells only need to proliferate without reaching a mature state (at this stage of development is not required to the embryo to produce for example fibroblast). Oct4 modulates its own expression and transcription factor's one. The core components of the pluripotency circuitry are formed by Oct4, Nanog and Sox2. The regulation of Oct4 usually goes together with the presence of Sox2; while Nanog expression is directly regulated by Oct4 and Sox2. Nanog has an additional role in discriminating those cells that will become epiblast and those who will become hypoblast. OCT4 is a homeodomain transcription factor of the POU family. The motor activity of Oct4 is the one who recruit additional transcription factor that could be 10 / 12 / 20 / 25. These factors, all together, regulate the activation or inhibition of specific promoters. Oct4 is the master transcription factor that coordinate transcription factors that can bind or not a specific promoter. Inhibiting those kind of genes, Oct4 maintain the undifferentiated state of cells; in contrast, by recruiting transcription factor (Sox2 or Nanog), Oct4 determine the transcription of those additional genes that are required to maintain the undifferentiated state. There are 2 isoforms of Oct4 (A and B). Oct4 is also able to regulate its own transcription (= positive feedback). 50 years ago the locus gene of Oct4 has been identified/sequenced. There are 2 type of promoters who regulate the transcription of Oct4: the DISTAL ENHANCER and the PROSSIMAL ENHANCER. The distal enhancer is used to drive the transcription of both form; when the embryo reaches the stage of the blastocyst, the distal enhancer works in the inner cell mass. Similarly, as the embryonic stem cells can be isolated from the inner cell mass, the same enhancer is also active in ES; this distal enhancer is active also in PGCs (primordial germ cells). This positive regulation of this enhancer determine the transcription of Oct4. In contrast, the proximal enhancer is activated only in the epiblast, so the inner cell mass are not in direct contact with the blastocyst. The cells that will form trophoblast don't express Oct4; the cells that will form inner cell mass do express Oct4. Transcription of either Oct4 and Cdx2 is the main molecular event that allow the discrimination between those cells that will begin trophoblast and those who will begin inner cell mass. Cdx2 is the typical marker of trophoblast; while Oct4 is the typical marker of the inner cell mass. At the stage of morula, when compaction occurs, the entire embryo starts transcribing Oct4, but concurrently the entire embryo starts also transcribing Cdx2. Depending on the reciprocal position that the cells acquired in the morula state, additional signals will determine which cell is able to maintain activation and which is not. Those cells that are in the external part of the embryo start maintaining transcription of Cdx2 (which is an inhibitor of Oct4); so in the external cells Oct4 transcription starts to slow down. In contrast, those cells who are incapsulated in the embryo stop transcribing Cdx2, so Oct4 can maintain its proper transcription. The Immunofluorescence on the right represents a mouse embryo development in vitro: at the stage of early morula, the cells that express Cdx2 are nearly the same of the cells that express Oct4, but there are some cells in the external part and others in the inner part. Those inner cells, at “late morula” state, stop transcribing Cdx2 (scientists didn't identify which molecular determine that), so Oct4 start to be confined in the inner part, although the external cells still express Oct4; but the maintenance of Cdx2 expression determine subsequently the stop of the transcription of Oct4 in the trophoblast cells. Once the blastocyst has formed only inner cells mass keep transcribing Oct4. Many studies try to identify which are the factors that determine the specific activation of Oct4 in the embryo. Initially it was thought that was the maternal Oct4 the responsible for his activation. Experiment 1: Oocyte that has been knock out for Oct4 (= the Oct4 gene doesn't work). When this kind of oct4-null oocyte is fertilized by a normal sperm (his genome contain the functionary sequence of Oct4, but the spermatozoa doesn't express Oct4, but is able to do it, if properly activated), the embryo goes on the development without problems. When the embryo activates its own proper transcription of Oct4, the embryo continues the development with any kind of problems. When this blastocyst is implanted in a receiving mother, an healthy mouse borns. Oct4 in the maternal transcript is not required for the proper activation of Oct4. Maternal Oct4 expression is not critical for developmental competence of the oocyte. Experiment 2: Scientists generate an embryo in which all the oocytes and sperms are knock out for Oct4. The embryo regularly develop in the stage of morula and early blastocyst, but it's no longer able to go on its development without the ability to activate Oct4. Beyond that point the embryo dies. Oct4 is not critical for the early stage of development, otherwise embryonic Oct4 is fundamental for the overall development. The activation of Oct4 by the cells of the embryo is critical for its proper development. Similarly, in the human embryo, at the stage of 8-cell, Cdx2 and Oct4 are both express by all cells, but going through the stages, the cells that transcribe Cdx2 or Oct4 redistribute within the embryo, and at the end, when the blastocyst is fully formed, only the trophoblast cells express Cdx2 and only the inner cells mass express Oct4 (and obviously not Cdx2 → they are mutually exclusive). The inner cells mass, once the blastocyst is formed, differentiate in 2 different lineages: – epiblast – hypoblast This kind of differentiation is driven by Nanog, which is under control of Oct4. The 2 transcription factors are Gata6 (expressed only in hypoblast) and Nanog (in epiblast). Nanog and Gata6 are mutually exclusive (nanog is an inhibitor for gata6 and vice-versa). The reciprocal disposition of the inner cells mass determine in which cells the expression of Nanog prevails and in which other cells the expression of Gata6 prevails. This molecular mechanisms is not fully identified. Some experiments in the mouse embryo identify different expression of FGF4 and FGF2, as 2 additional factors, that help the inner cells mass to discriminate which cells will express Nanog and which Gata6. When the blastocyst is fully form, it is composed by different kind of cells: – trophoblast (expressing Cdx2) – epiblast (expressing Nanog) – hypoblast (expressing Gata6) While all this events occur, the embryo is travelling along the tube to reach the uterus. The human blastocyst is fully formed around the beginning of day 6 of development and the embryo has reached the uterus and now need to implant. The event that allowed the embryo to implant is: hatching outside of the zona pellucida (envelop the oocyte / zygote). The cells of the trophoblast start digesting cells of zona pellucida. Once it is hatched, it is free to implant. The initial mechanism of implantation is essentially driven by 2 kinds of cells adhesion molecules: L-selectin (express by the cells of the trophoblast) find connection with oligosaccharides (exposed by the endometrium epithelium). Once this kind of connection has been made, the blastocyst starts rolling along the epithelium until a stronger interaction is given by integrins (expressed by the the cells of the trophoblast) and corresponding receptor of endometrium epithelium. At this point, the embryo starts digesting the maternal tissue; this digestion is driven by the cells of the trophoblast. The cells of the trophoblast, once the embryo starts implanting, differentiate into 2 lineages: Cytotrophoblast (is an active proliferating kind of cells; when they proliferate, some cells remain cytotrophoblast, so they delimit the border of the embryo, while some others differentiate into syncytiotrophoblast) Syncytiotrophoblast (is a tissue that actively digest the maternal endometrium; it's produced by proliferating cytotrophoblast cells that at the end don't experience cytokinesis. This cells deeply enter the maternal endometrium). At the end of the 1 week of the development the embryo is implanted. The embryo usually takes one week to be completely incapsulated by maternal endometrium. SITES FOR ECTOPIC PREGNANCIES: Sometimes it can occur that the embryo doesn't implant in the correct tissue → ectopic pregnancies. It depends on mutation in self cells adhesion molecules (in the embryo) or it depends on mutation in cells adhesion molecules on the maternal tissue. Usually the fertilization occurs in the Ampulla and the zona pellucida has also the role to block the implantation of the embryo while he is traveling along the tube. But if there are problems (example: mutation of proteins) the cells adhesion molecules, that are present on the external part of the embryo, determine the implantation in the tube tissue, that obviously are not responsive to the progesterone stimulation (role of progesterone is to allow the uterus to be ready to receive the embryo). When tubal pregnancy occurs, the embryo continue to develop correctly until the moment in which the tubal tissue is no longer able to sustain its growing and generate bleeding and haemorrhagic events (really dangerous for the mother); obviously the embryo dies. The problem concern the mother essentially. The vast majority of ectopic pregnancies occur in the tube, but sometimes ectopic pregnancies can also occur in the ovary (this determine the disruption of one of the 2 ovaries); the embryo keeps growing and digest the ovary. In addition, from an anatomical point of view, the ovary from which the oocyte is released, when the oocyte is ovulated the tube is not in complete contact with the ovary: the fimbriae (distal part of the tube) are open into the peritoneum. The embryo, in this wrong case, escapes from the tube and enters the peritoneum and tries to find a gut tissue / gut mucosa to implant. It's really dangerous (the most dangerous type of ectopic pregnancy) for the mother because the haemorrhagic events are huge. Another part in which the embryo could implant in a wrong way is the lower part of the uterus: it's a type of ectopic pregnancy that may not affect the mother's or fetus's health, but it has to be controlled. At the moment of delivery there could be some kind of dangers for both the baby and the mother. The correct position in which the embryo implant is the anterior or the posterior portion of the uterus. On the right there is an example of a tubal pregnancy in which the embryo properly develop but the tissue is not able to support for long time this kind of role in a right way; at a certain moment the tissue will be broken by the growth of the embryo, generating haemorrhagic events. HYDATIDIFORM MOLES (not properly an ectopic pregnancy) is a sort of tumour that can occur when an embryo, with a particular problem, starts to implant; usually the hydatidiform is a sort of tumour that is generating by the trophoblast (because it's the tissue responsible for implantation of the embryo). It happen when in an embryo is present only the paternal genome (= an embryo that cannot survive, because the maternal genome is absent). When the nucleus of the sperm enter an oocyte that doesn't own nucleus, the sperm produces a mitotic division that reconstitute the 2n stage of the DNA and, based on that, the oocyte and the pseudo-zygote start to develop 2-4-8-16-cells, as structures resembling the blastocyst, and when this structure reaches the uterus, it start implanting. This kind of structure, producing only by paternal genome, has a functioning trophoblast, which starts implanting but, without the maternal genome (that has been proven, it controls the rate of digesting), this digestion keep going and going, generating this kind of tumour (= hydatidiform moles). It can be removed with some problems for the mother. In some cases, it could continue to digest the endometrium, generating haemorrhagic events. What prove the existence of this kind of structure? The regulation of the growth of moles is due to the presence of the paternal genome. Thus, during the earliest stages of development, paternal genes regulate differentiation of the trophoblast (placenta), while maternal genes regulate growth of the embryoblast. This implies, also, that the presence of the paternal genome doesn't allow the differentiation of the inner cells mass, of the epiblast or hypoblast. On the right is possible to see an implanted embryo. While the embryo is implanted, in concurrence with that there are some additional events and also the embryoblast (composed by the epiblast and hypoblast) keeps growing and starts producing new structures that are required for the proper growth of the embryo. The embryo is composed by 2 layers of cells: – epiblast (blue) – hypoblast (yellow) Some flat cells start to be produced from the mitotic activation of the epiblast; these cells delimitate a space filled by liquid produced by the epiblast cell. This is the initial amniotic cavity. 2-3 days after the implantation, the epiblast acquire this cylindrical shape and represent the floor of the amniotic cavity. Concurrently, the roof of the amniotic cavity is formed by epiblast cells that acquire a flat shape and are in direct contact with cytotrophoblast (that it is sustaining the formation of syncytiotrophoblast). The flat epiblast cells exit the route of embryo development; so only cylindrical epiblast cells will participate to the further development of the structure of the embryo. Concurrency, the hypoblast start proliferate and generate Heuser's membrane, that is in direct contact with the cytotrophoblast. This membrane delimitate a space corresponding to a primitive yolk sac. This is the point in which, initially, the embryo has enter the maternal endometrium. While the implantation keeps going, 2 cavities form: the amniotic cavity (formed by the epiblast cells) the primitive yolk sac (formed by the Heuser's membrane, produced by hypoblast) Once the 2 cavities have been formed, from the point of connection (on both sides of the embryo) when hypoblast and epiblast merged, a new type of cells is produced. Today it's not clear if those dark green cells are produced starting from proliferation of hypoblast cells or epiblast cells. Another layer, the extra-embryonic mesoderm, forms around the embryo and yolk sac and inside the cytotrophoblast. Initially, this layer is solid, but soon spaces appear (continued proliferation) causing a separation into 2 layers: extra-embryonic splanchnopleuric mesoderm (splanchnic or visceral layer → that means it is internal) around the yolk sac and embryo. It is in contact with the Heuser's membrane. extra-embryonic somatopleuric mesoderm (somatic or parietal layer → that means it is external) lining the inside of the cytotrophoblast. This 2 type of mesoderm, although they don't participate, they will have a fundamental importance for the early phase of embryo development: the somatic / external mesoderm, together with cytotrophoblast and syncytiotrophoblast, will form the Chorionic plate (the chorion = that part of the placenta that cover the embryo); the visceral mesoderm, under the regulation of the Heuser's membrane, will be the very first place in which initial blood cells will be produced. Those cells initially supply oxygens to the developing structures of the embryo; quite soon they will be lost and the blood cells produced by the embryo protein start to function. Concurrently finger-shaped structures, of the cytotrophoblast, are formed, within the syncytiotrophoblast. Lacunae are spaces generating by the syncytiotrophoblast that will be invaded by maternal sinusoid / blood. In correspondence with lacunae, filled by maternal blood, is possible to see some finger-shaped projection of the cytotrophoblast. – Primary villus → are initially composed by a core of cytotrophoblast, enveloped by syncytiotrophoblast. – Secondary villus → when the somatic extra-embryonic mesoderm starts invading cytotrophoblast core, it generates a structure formed by 3 type of cells: a core of somatic extra-embryonic mesoderm, enveloped by cytotrophoblast, which is enveloped by syncytiotrophoblast. – Tertiary villus → when blood vessels of the embryo invade the mesoderm core, this tertiary villus is formed. This is the final organization. Around the 12 weeks of development it will be ride to a fully formed placenta. Primary and secondary villus are formed all over the surface of the embryo, but while the embryo is growing and it occupies the entire space of uterus, the tertiary villus will be formed, only on the side where the embryo is. Focusing on the external mesoderm, is important to note that in every part of the embryo this 2 layers are separated. But, in correspondence of a region, the visceral and the somatic layers merged, forming, around 12 days of differentiation, a structure called connecting stalk. It will allow the embryo to hang on within the cavity and it will be the region where the umbilical cord will be formed to connect the placenta to the embryo. During implantation, the Heuser's membrane delimits the entire blastocyst. When visceral and somatic extra-embryonic mesoderm are fully formed, on the equatorial plane of the blastocyst, a proliferation of the visceral extra-embryonic mesoderm determine a narrowing of the original primitive yolk sac. While this narrowing keeps going on, the entire primitive yolk sac is divided in 2 additional spaces: Secondary / definitive yolk sac → a cavity, always delimited by Heuser's membrane, that is delimited by visceral extra embryonic mesoderm; the roof is represented by the hypoblast; the remaining membrane coming from the division of the original blastocyst, will be digested. Chorionic cavity At the end of the second week of development, the embryo appears like a bi-laminar structure, composed by 2 layers of tissue (epiblast and hypoblast). The embryo is divided into 2 cavities: Amniotic cavity (delimited by the epiblast) is above; Yolk sac is below. The embryo is now hanging in the extra-embryonic cavity, that has been generating following the formation of the definitive yolk sac, by the connecting stalk. Secondary and then tertiary villi are only on the embryonic pole of the cavity. At the end of the 2nd week (14 days) of development the implantation is concluded. The image, on the left, is an human embryo obtained following scraping of the uterus, due to some kind of abortion. The nuclei of the cells that composed the embryo have been marked. Gastrulation starts exactly at the beginning of third week of human embryo development. Some women don't realize they are pregnant until the second month of pregnancy, as already mentioned. In some case it is not their fault; it is due to a physiologic event. The pink circle represent the exact point in which the embryo has entered the endometrium. When the embryo is fully implanted, at the end, the endometrium join the 2 parts of the epithelium / merged / envelop complete the embryo within the endometrium. In some cases, when this 2 parts merged together, a sort of more or less conspicuous bleeding can occur. Counting the days during which all this events occur: – in average, ovulation occurs at the 14 days of the menstrual cycle – fertilization can occur within the subsequence 24-36 hours (at the 15 days of the menstrual cycle, fertilization occurs) – full implantation occurs following 14 days from the fertilization 14 + 14 = 28 days – at 28 days can occur this kind of bleeding that can be mistaken for menstrual cycle. A woman has to wait another month to know that she is in amenorrhoea due to pregnancy. – At 14 days of development gastrulation occurs and last for additional 2 weeks. So one other month. At the end of the first month gastrulation is concluded; so a woman cannot be conscious she is pregnant, until the moment gastrulation has been completed. She could have some kind of behaviour (for example drinking ethanol) that can put at risk the pregnancy or induce birth defects. 6/12/2022 RECAP: At the end of the 2nd week of development the embryo is formed by 2 layers of cells: the epiblast and the hypoblast. They have acquired a defined morphology which is cylindrical/columnar in the case of the epiblast and is almost ovoidal for what concern the hypoblast. The embryo at this stage is attached to the cytotrophoblast, so the syncytiotrophoblast and the forming future placenta, through the region where umbilical cord will form, which is the region where the two layers of extraembryonic mesoderm (splanchnic and visceral) join together. The zygote segments, through consecutive cleavages, reaching the condition of blastocyst where the first differentiation in inner cell mass and trophoblast occurs, and where the hypoblast and epiblast, derived from the inner cell mass, differentiate one respect to the other. The cells of the epiblast will form the amniotic ectoderm, while cells of the hypoblast will form the Heuser’s membrane. Both these two groups of cells participate in forming the extraembryonic mesoderm. From the hypoblast, the cytotrophoblast will differentiate and proliferation of the cytotrophoblast will produce the syncytiotrophoblast which is the embryonic tissue that erodes maternal endometrium. At the end of the 2nd week of development gastrulation starts occurring. The initial signal that identifies the beginning of the gastrulation is the formation of the primitive streak, which is a sort of organizing structure of the embryo during gastrulation. The cells of the epiblast will start to differentiate in the 3 embryonic germ layers, from which every single kind of tissue will develop. From the primitive streak there is also the formation of additional extraembryonic mesoderm. Extraembryonic mesoderm comes from the hypoblast which will not participates in the formation of any part of the developing embryo except for the very first blood cells that initially will circulate in the embryo. The 3 germ layers that are fully formed at the end of gastrulation will produce any kind of tissue of the embryo: Ectoderm: from this layer the entire nervous system will form Mesoderm: from this layer the cardiovascular system, bones, connective tissue, urogenital system, skeletal muscle tissue Endoderm: from this layer originate gut, liver and also lungs This image delineates all the different lineages that come from every single layer. Following the initial differentiation of the neural system, which comes from the ectoderm, the green box identifies a kind of cells, called neural crest cells, that some embryologists define as the fourth germ layer, but in reality, these are not. These cells have an ectodermal origin and differentiate into a plethora of tissue and particular cells within the embryo. Neural crest cells also differentiate in fully differentiated cells that usually comes from mesoderm, endoderm or ectoderm, in fact neural crest cells are capable of differentiating into adipocytes, that usually differentiate starting from the mesoderm layer, in some part of the heart, into bone cells present in the face, so the differentiation potential of these cells appear to be even greater than the differentiation potential of the single cells that are initially present in the three germ layers. The entire part of the proper embryo (excluding yolk sak and amniotic cavity) derives from cells of the epiblast, in fact, from the embryonic epiblast (in blue) the 3 germ layers differentiate. Which kind of germ layer generates primordial germ cells -yellow- ? None of them, in fact the differentiation of primordial germ cells (PGCs) occurs very early during gastrulation (in the first and second day of gastrulation), even at the moment of blastocyst formation, there are some molecular signals that specific cells are able to respond, which tell them to start their journey to become gametes. This kind of specification, of which we don’t actually know the molecular mechanisms, occurs at the moment of blastocyst formation, and this kind of specification can determine the behavior of this group of cells during the gastrulation. So, if any kind of tissue has a specific derivation from a well-defined germ layer, usually embryologists say that primordial germ cells derive from the epiblast. During embryo development, embryo grows in volume and the entire space of exocoelomic cavity will be occupied by the embryo, so around the end of gastrulation is completely occupied by the embryo. The embryo at the beginning of gastrulation is bilaminar (epiblast and hypoblast) and it has a 2- dimensional morphology, at the end of gastrulation the embryo acquires the so-called fetal morphology, which consists in cylindrical morphology. So, during gastrulation the 3 germ layers form and the embryo, at the end of gastrulation, acquires its fetal tridimensional morphology. At the end of gastrulation several structures can be recognized, essentially the structures that form the nervous system are formed, the mesodermal structure that will form the entire skeletal muscles system, the presence of otic placode that represents the region where eyes and ears will form, and the paraxial mesoderm starts to acquire its segmented morphology. All these events occur during the 3rd and the 4th week of development. A quite famous sentence of Lewis Wolpert is: 'It is not birth, marriage or death, but gastrulation which is truly the most important time in your life'. While gastrulation occurs the 3 germ layers will start to form, and initially they will form a trilaminar embryo, but this trilaminar embryo will be bidimensional as well during the first week of gastrulation (3rd week of development). Subsequently, during the 4th week of gastrulation, embryo starts to fold in a particular way in order to generate the tridimensional and fetal morphology. (During the first phases of gastrulation the three germ layers are formed but being bidimensional. These three germ layers are in continuity with external tissues of the embryo, in particular the new formed endoderm will be in continuity with extraembryonic endoderm which is represented by yolk sak. In the early stages of gastrulation, the new formed endoderm is in continuity with the Heuser’s membrane that delimits the yolk sak. Similarly, the ectoderm is in continuity with the amnioblasts, those cells that delimits the amniotic cavity. The mesoderm is in continuity with the extraembryonic mesoderm, more precisely the part of the extraembryonic mesoderm (visceral mesoderm) which is contact with the Heuser’s membrane.) Once the embryo is full implanted, it starts gastrulating and the very first event that identify the beginning of gastrulation is the appearance of the PRIMITIVE STREAK, which is a structure that originates from proliferation of longitudinal cells of the epiblast that give rise to columnar cells that arrange themselves to form a depression between the two rows of columnar cells. In the middle of the embryo, more precisely at the end of the primitive streak, there is a depressed enlargement called primitive node. We don’t know why the primitive streak starts forming in a specific site of the embryo, but this site in which primitive streak starts forming will represent the caudal portion of the embryo. The primitive streak begins at the very caudal portion of the embryo and proceeds lengthwise to the cranial part of the embryo, without reaching the cranial part, but stopping, more or less, in the middle of the embryo. There are some structures that start to form while the primitive streak is forming, including the oropharyngeal membrane and simultaneously, at the starting point of the primitive streak, the cloacal membrane (starting point of the primitive streak). What are these two membranes? These are 2 regions of the bilaminar embryo, in which the epiblast and the hypoblast are strongly attached due to the formation of tight junctions between a selected part of the cranial portion and a selected part of the caudal region, these regions essentially will represent the region where the mouth and the anus will form. The direction that the primitive streak acquires, determines the formation of the first axis (caudal cranial axis) of the embryo. The axis are: cranial-caudal axis right-left axis dorsal-ventral axis For the complete specification of the cranial-caudal axis of the embryo a great importance is claimed by a region called AVE (anterior visceral endoderm), which is a region that initially acquires specific characteristics within the hypoblast, and which expresses head forming genes. Concurrently to the beginning of the gastrulation (formation of the primitive streak), on the opposite side of the embryo (cranial region), the cells start acquiring specific molecular characteristics that are initially represented by the transcription of 3 transcription factors: OTX2, LIM1, HESX1. These factors are expressed only by the AVE cells, so only by the hypoblast cells that are in the cranial part of the embryo. The selected expression of these factors in that region, regulates the transcription of CER-1 and lefty1, which are secreted factors. So, those cells actively secrets CER-1 and lefty1 determining a gradient of concentration of these factors, that is higher in the cranial part of the embryo and is progressively lower going to the caudal part of the embryo. This gradient of secreted factors can put the cells of the embryo in an environment in which the concentration of these factors is progressively different. Which is the main role of these factors? These are inhibitors of NODAL, WNT and BMP4 signals, this means that the effects of these factors, which can recognize specific receptors on the cells and activate a signal transduction, will be progressively higher toward the caudal part of the embryo (due to lower expression of CER-1 and lefty1). So those 3 factors are highly inhibited in the cranial part of the embryo due to the high concentration of CER-1 and lefty1, this concentration decreases going toward the caudal part, this means that also the inhibition of NODAL, WNT and BMP4 progressively slow down. So, caudal cells highly sense the effects of NODAL, WNT and BMP4. NODAL, WNT, BMP4 are actively produced and secreted by cells of the primitive streak, and in particular, the production of NODAL is a specific competence of the cells of the node (that is why it is called NODAL). The transcription, translation, and secretion of NODAL, WNT and BMP4 are sustained by Fibroblast Growth Factor 8 (FGF8), so cells of the primitive streak and primitive node actively produce FGF8. OTX2, LIM1, HESX1, CER-1 and lefty1 = “cranializing factors” NODAL, WNT and BMP4 = “caudalizing factors” The hypoblast is not involved in gastrulation. Once the primitive streak and the primitive node are formed, all cells of the epiblast start their proliferation and migrate. Migration is one of the main events typical of embryo development (migration is fundamental for the proper embryo development) → the cells of the embryo must acquire the proper position within the embryo. The entire cells of the epiblast start proliferating and migrating toward the primitive streak and the primitive node. In the picture, the black arrow indicates the direction of the migration of cells of the epiblast. So those migrating cells start entering into the primitive streak and into the ancient node in different part of them and, once they entered, they continue their migration underneath the epiblast. Nearly all the cells of the original epiblast migrate toward the primitive streak, to do that the epiblast cells must acquire the ability to migrate, and this ability is achieved through a well-defined cellular event called EMT (Epithelial to mesenchymal transition). The cell-cell adhesion is lost following this kind of transition, that makes the cell able to move from a jointed cell and to start migrating. Migration occurs following specific molecules that are present in the extracellular matrix, such as collagen, fibronectin. All those fibrous proteins, that characterize the extracellular matrix, represent a track on which the cells migrate. The cells must also be able to stop in specific position when they reach the place in which they have to produce a specific generation of cells. This means that the EMT is a reversible process, and the reverse process is called MET (Mesenchymal to epithelial transition). Cells are blocked in a specific position thanks to various types of junctions, but due to external signaling (extracellular signals, epigenetic regulators, cross talk between these two types of signals) the cells lose their cell polarity (for example, epithelial cells of the gut are characterized by a specific polarization with a basal region and an apical region), they meet a huge cytoskeleton remodelling, they start to produce focal adhesion that allow the cells to recognize specific proteins of the extracellular matrix and allow the cells to respond to specific chemical attractants. All of this leads to the final event of the EMT which is the cellular migration. Essentially, 3 types of EMTs have been described: 1. Primary EMT (the one that is present during gastrulation) → ends up in a simple migration of epiblast cells, and when they stop migration, they don’t invade region of the embryo. Obviously, this kind of situation is not present in those cells that, for example, acquire a migratory phenotype and start invading regions of the organism where they are not supposed to go (= that is tertiary EMT case). 2. Second EMT → it occurs during the differentiation of mesoderm and the differentiation of neural crest cells. Secondary EMT is a mechanism in which a cell acquires the ability to migrate reaching its final destination in which will properly differentiate in the cell that are needed for the embryo in that particular region; for example all skeletal muscles derive from mesoderm and, in particular, from the paraxial mesoderm, but at the end of gastrulation the entire paraxial mesoderm is represented by a sort of mesodermal segmented regions in the dorsal part of the embryo, from every single segment, skeletal muscles will form. Once neural crest cells form, they will not never be able to form skeletal muscle for example, so the differentiation ability is restricted; similarly, cells of paraxial mesoderm, that will form skeletal muscles, will no longer be able to differentiate toward, for example, in neurons, so their differentiation faith has been restricted following the gastrulation and the formation of the three germ layers. 3. Tertiary EMT → occurs in metastatic cells, those cells that acquire a migratory phenotype following an EMT, but they are no longer able to control this kind of mechanism generating metastasis. During the early phases of gastrulation there are 2 well defined waves of migration: 1. The first one includes the epiblast cells that proliferate and then migrate toward the primitive streak and the ancient node, invaginate within these two structures and start displacing the cells of the underneath hypoblast in the way that we see in the image on the right (= in all directions of the embryo). All this kind of migration of the first wave cells will determine the progressive displacement of hypoblast cells, and at the end of this displacement the entire hypoblast will be replaced by cells of the epiblast that have migrated during the first wave of migration. Those cells of the first migration wave will form the embryonic endoderm, and following this displacement, the epiblast has not been detached by the new formed endoderm. Remember that during this first wave also the cells of the hypoblast that are in closer position with the cells of the epiblast in correspondence of the cloacal membrane and the oropharyngeal membrane are replaced by cells of the endoderm, that maintains this level of attachment. So, the oropharyngeal membrane and the cloacal membrane are maintained also when the embryonic endoderm is fully formed, but in the other part of the embryo, the newly formed endoderm and the epiblast are now detached leaving a space between them. 2. During the second wave of migration occurring always an epiblast cell-shift, that proliferate and migrate toward the primitive streak and the ancient node, filling the space between the endoderm and the epiblast, generating the mesoderm. Concurrently, the remaining cells of the epiblast start differentiating toward ectodermal cells. This kind of invagination between the primitive streak and the ancient node (= Hensen's node) determines the formation of the 3 germ layers: the endoderm at first with the displacing of the hypoblast, the mesoderm which fills the space between the endoderm and the epiblast, and while the mesoderm is forming, the remaining cells of the epiblast start differentiating into the ectoderm. All the cells of the first wave of migration enter within the primitive streak and those cells during the first wave will form the embryonic endoderm. During the formation of the endoderm there are a little percentage of cells coming from the epiblast that enter within the node, once they enter will starts to migrate in longitudinal direction towards the cranial part of the embryo. Those cells → will form the prechordal cells and the prechordal mesoderm. The prechordal cells will displace those cells of the hypoblast that forms the oropharyngeal membrane, and they will attach to the cells of the overlaying epiblast maintaining the attachment of these two structures. Similarly, for what concern the cloacal membrane, some cells that enter the primitive streak will migrate towards the caudal part and will displace the cells of the hypoblast that concur to the formation of the cloacal membrane. Which is the importance of these two membranes? These are the region where anus and mouth will form. During the second wave of migration, that leads to the production of mesoderm, the entire space between the forming ectoderm and the formed endoderm is filled by mesoderm cells, except for the regions of the oropharyngeal membrane and the cloacal membrane, due to the strong attachment of these two regions. Prechordal mesoderm is composed by a little part of cells of the first migration which enter within the node; the very first cells that enter the node during the first wave of migration will form the prechordal cells, that will displace the hypoblast of the oropharyngeal membrane. The second group of cells that enter within the node during the first wave of migration, similarly to the prechordal cells, will migrate longitudinal in cranial direction and they will delineate the regions where a mesodermal structure note as notochord must form during the second wave of migration. This structure is fundamental for the proper development of the embryo, and it acquires its proper position along the embryo since the first wave. The primitive streak proceeds from the cloacal membrane to the middle part of the embryo. The cells that migrate can enter within the streak at different point of the streak; depending on the point in which the cell enters, it will determine the migration that the specific cell will have once invaginated. It means that the closer to the cranial area they enter, the more cranial will be their migration. Similarly, those cells that enter in the middle part of the streak will start migrating laterally and cranially, or completely laterally, or laterally and caudally, and those cells that enter on the most caudal portion of the streak will migrate caudally reaching a position that will determine the future differentiation faith of those cells. Mesodermal cells that migrate on the caudal part will give rise to a progeny of cells that for example will be necessary for caudally structures. Those cells that enter exactly in the node will migrate in correspondence of the main axis of the embryo in a sort of prolongation of the primitive streak. The NOTOCHORD: – the notochord is the first rigid axis of the embryo (primitive streak was not a rigid axis) – establishing the longitudinal axis of the embryo, allowing the lengthening of the embryo in cranial-caudal direction – is involved in paraxial mesoderm specification – induces ectoderm differentiation in neuroectoderm This is fundamental because notochordal cells actively participate in the specification, so in the signaling that induces a specific part of the mesoderm to differentiate toward the paraxial mesoderm, which will form on both sides of the notochord. This structure will extend from the node to the oropharyngeal membrane and the prechordal cells will stop the cranial progression of the notochord. The notochord will be formed by the cells that during the second wave of migration invaginated within the node. This structure will be important to induce the paraxial mesoderm specification close to the proximity of the longitudinal axis of the embryo. The mesoderm will differentiate in 3 parts: the paraxial mesoderm, close to the proximity of the longitudinal axis the lateral mesoderm, near the edge of the embryo the intermediate mesoderm, between the first two. Notochord is also fundamental for the specification of the overlaying part of the embryo, which is the neural ectoderm, that will form the brain and the spinal cord. The notochord is fully formed before the end of the 3rd week of development. During the second wave of migration, those cells that invaginated in the node start migrating toward the prechordal plate, and following the track imposed by the prechordal mesoderm, delineate the region where the notochord will form. Following this morphologically signaling, the cells start form a sort of tube that initially is empty. The maturation of the notochord is represented by the subsequent fusion of the ventral part of the initially formed notochord with the endoderm, giving rise to a structure called notochordal plate (placca cordale), a structure that puts in continuity the endoderm with the notochord. Around the 18-19 days of development, the 2 pages of the notochordal plate start to proliferate intensively and fuse ventrally, so the 2 edges of the endoderm previously in contact with the notochord fuse giving rise to a tubular structure that is the final notochord. Once is fully formed, the notochord starts inducing the overlaying part of the ectoderm that will become neural ectoderm. In the following two days (around the 18-19 days of development) the cranial part of the embryo develops more quickly respect to the caudal part, just because the neural ectoderm has been induced and the region that will form the brain starts forming. In fact, initially the embryo proliferates and grows essentially in his cranial part, due to the activity of the fully formed notochord. While the embryo is growing cranially, it also extends in length, but the primitive streak and the node don’t follow the elongation of the embryo, while the embryo is growing, the primitive streak and the node are progressively restricted into the more caudal part of the embryo. The cells that during the 4th week of development (second week of gastrulation) invaginate within the primitive streak, that is progressively restricted to the caudal part of the embryo, will give rise to caudal mesodermal part of the embryo. The main migration of the cells initially is toward the cranial part. So, in the cranial part the mesoderm is fully formed before the end of the 3rd week of development, while in the caudal part, the mesoderm is not fully formed yet. The nervous system is formed starting from the ectoderm. Following the induction of the notochord, a specific portion of the ectoderm is induced to proliferate and start differentiating into the so-called neural ectoderm. The morphological event, that occurs once the notochord is fully formed, is a proliferation of the neural ectoderm, which is not the entire part of the ectoderm, it is only the part of the ectoderm in close correspondence of the notochord. Those cells will sense a specific growth factor, while the cells that are distant from the notochord will not sense the induction by the notochord of this growth factor, so they will acquire a different fate. So, the cells close to the notochord will form the neural ectoderm, while the part of the ectoderm which is not under the induction of the notochord will form the epidermis. While the dermis, which is a connective tissue, will differentiate starting to lateral mesoderm and the paraxial mesoderm. So, the proliferation of the neural ectoderm, under the induction of the notochord, will determine the formation of 2 neural folds divided by a depressed mid-region called neural groove; these structures progressively move one toward the other in ventral position and fuse dorsally to form a tube that runs from the cranial part toward the caudal part of the embryo. T he fusion begins in the cervical region and proceeds cranially and caudally. – The cranial most portion of the neural ectoderm → will form the brain – the middle and caudal most portion of the neural ectoderm → will form the spinal cord and all the typical structures of the nervous system. Concurrently to the differentiation of the ectoderm, the entire mesoderm differentiates toward its typical 3 parts: paraxial mesoderm → in close proximity to the notochord along the entire part of the embryo. This one will form somites lateral mesoderm → the lateral part of the mesoderm will differentiate into the lateral mesoderm, which before the end of the 3rd week splits in 2 portions: ◦ a dorsal portion → lateral somatic mesoderm ◦ a ventral portion → lateral splanchnic mesoderm intermediate mesoderm → it will form the gonads and parts of the urogenital system. At the end of the 3rd week (21 days) of development, while gastrulation is still occurring in the caudal part, the embryo starts to fold ventrally. All the cranial parts of the embryo are proliferating very intensely, and this proliferation determines a driving force that pushes the embryo to fold ventrally. So, at the end of gastrulation (28th day of development), the 2 edges will fuse determining the ventral closure of the embryo, concurrently, the plentiful proliferation of the neural ectoderm in the cranial part, and even later in the caudal part, will produce the driving force that will fold the embryo ventrally. The ventral, cranial and caudal closure of the embryo will determine the acquisition of the fetal position. In the mesoderm there is the formation of segmented structures that identify the initial differentiation of the paraxial mesoderm in segmented structures called somites. While development keeps going on, the 2 edges of the neural bridge start to fuse dorsally, and the fusion occurs more or less in the middle part of the embryo in correspondence of the fourth/fifth somites that are forming in the paraxial mesoderm. The two edges fuse in a way very similar to a zip, and the neural tube starts to close in cranial and caudal directions. The abundant proliferation of the neural tube determines the driving force necessary to fold the embryo caudally and cranially, while the quick proliferation of the paraxial mesoderm provides the driving force necessary for the lateral folding of the embryo. So, the embryo folds in every direction acquiring the fetal position. We are at the beginning of the 4th week of development, the neural tube is closing along the entire length of the embryo and the primitive streak with the node are now restricted to the very caudal most region of the embryo, but gastrulation is still occurring, so cells from the ectoderm are still migrating within the primitive streak and the node, in particular, those cells will give rise to the main part of the mesoderm that must be formed in the caudal part of the embryo. At the end of gastrulation, the entire primitive streak disappears, and the primitive node is reabsorbed by the embryo. Concurrently the notochord follows the very same phases of the primitive streak, with a difference, the entire notochord will be reabsorbed once that has excepted its induction activity. The entire notochord will disappear in the adult, except for some cells that will become the nuclei pulposi of the intervertebral discs. At the end of gastrulation there is no more primitive streak and no more node, but if cells of these two structures are not properly reabsorbed, or if during the fourth week of development, the embryo is exposed to external substances such as alcohol drunk by the mom, the very late migration within the streak, that is required to form the caudal mesodermal portion, cannot occur properly, and some kinds of malformations can occur. SIRENOMELIA is a malformation in which the lower limbs are fused together, taking on the appearance of a mermaid’s tail. Not compatible with life, so the fetus will be aborted. SACROCOCCYGEAL TERATOMA → If cells of the primitive streak and node are not completely reabsorbed, they could start to proliferate in an uncontrolled way, giving rise to a sort of teratoma. It is present in 1 out of 35000 newborns, so it’s quite rare, but it is the main kind of teratoma that depends on a not proper reabsorption of the cells of the primitive streak and node. This kind of birth defect is compatible with life. During neurulation, when the 2 edges of the neural bridge fuse dorsally, in correspondence of the point of fusion, the neural crest cells are fully formed. They will form a sort of cord of cells on top of the neural tube, that will close dorsally and over the neural tube a solid region of cells start forming. This solid region lasts for about few hours and then immediately these cells start migrating in any direction of the embryo following specific signals. So, keep in mind that in every single point in which the neural bridge fuse together, in that specific moment, a group of neural crest cells will form. The closure of the neural tube proceeds cranially and caudally starting from the middle part of the embryo (4th pair of somites). When the closure of the neural tube is about completed, there are 2 pores (the only parts opened of the neural tube): anterior neuropore (cranially) → closed at the 25th day of development posterior neuropore (caudally) → closed at the 28th day of development which corresponds to the end of gastrulation. The gastrulation ends when the neural tube is fully formed and completely close. So, the closure of the neural tube is a very important moment for the proper embryo development, and in fact, the top of the risk of birth defects correspond to the beginning of the fifth week of development (end of gastrulation). Many birth defects arise from the not proper closure of neural tube. ANENCEPHALY → the anterior neural pore doesn’t close properly. This condition isn’t compatible with extrauterine life, this means that an anencephalic embryo can complete the pregnancy, but due to the fact that the anterior neuropore has not closed properly, nearly the 90% of the brain will not form. The embryo is alive during the entire pregnancy, but when the embryo will born he will die → the cause of death will be based on the fact that respiratory centres are not developed and therefore the newborn will not be able to breathe. This is a great problem for the mother because the fetus is alive and then will die. This condition can be diagnosed around the 7th week and law allows the therapeutic abortion. SPINA BIFIDA → In contrast, if the anterior neuropore closes correctly, but the posterior neuropore doesn’t close properly there will be a birth defect that is compatible with life. The spina bifida may have more or less severe impact in the quality of life of the newborn. This kind of defect has an incidence of 1 newborn out of 5.000 and it can be essentially divided in 3 types of spina bifida: Spina bifida occulta: only the posterior access of the vertebrae doesn’t fuse properly, due to the not closure