DNA as the Genetic Code (Protein Synthesis) PDF - Biology A2 - CCEA
Document Details
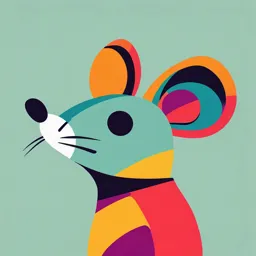
Uploaded by StrongSard1087
CCEA
Tags
Summary
This document covers the genetic code, protein synthesis, including transcription and translation processes. It explores the structure of DNA and mRNA, and also examines epigenetic modifications. Relevant features of the genetic code are explained via key definitions of processes, and clear graphical representations.
Full Transcript
Okay, here's the conversion of the attached text into a structured markdown format. I've focused on retaining the key information, formatting tables, and converting formulas where applicable. ## Chapter 10 - DNA as the Genetic Code (Protein Synthesis) **Students should be able to:** * 5.3.1 Dem...
Okay, here's the conversion of the attached text into a structured markdown format. I've focused on retaining the key information, formatting tables, and converting formulas where applicable. ## Chapter 10 - DNA as the Genetic Code (Protein Synthesis) **Students should be able to:** * 5.3.1 Demonstrate knowledge and understanding of the nature of the genetic code. * 5.3.2 Demonstrate knowledge and understanding of the process of transcription in synthesising proteins. * 5.3.3 Demonstrate knowledge and understanding of the process of translation in synthesising proteins. * 5.3.4 Demonstrate knowledge and understanding of the one gene one polypeptide theory. * 5.3.5 Demonstrate knowledge and understanding of the concept of epigenetics. ## The Nature of the Genetic Code Deoxyribonucleic acid (DNA) is the molecule of inheritance; it is a 'blueprint'. DNA allows traits to pass through generations with limited change. Understanding DNA helps us understand genetic variation. DNA determines the polypeptides (and proteins), particularly enzymes, produced by cells. These enzymes control cell metabolism. ### The nature of the genetic code DNA contains repeating units of deoxyribose sugar and phosphate. The two strands are linked by nitrogenous bases: adenine, guanine, cytosine, and thymine. Adenine always links with thymine, and cytosine with guanine, through hydrogen bonding. The template strand is the linear order of nitrogenous bases that forms the DNA code. Polypeptides and proteins are made from 20 different amino acids. The sequence of amino acids (the primary structure) determines the overall polypeptide structure. DNA controls the primary structure of polypeptides it codes for. The way DNA codes for specific amino acids is the *genetic code*. The length of DNA coding for a polypeptide is a *gene*. ### The genetic code DNA contains four different types of bases, so one base cannot solely code for one amino acid (as there are 20 amino acids). Two consecutive bases are also insufficient, yielding only 16 possible combinations ($4 \times 4 = 16$). **The 16 possible combinations are:** AA, AC, AG, AT, CC, CA, CG, CT, GG, GA, GC, GT, TT, TA, TC, TG However, three consecutive bases yield 64 possible combinations ($4 x 4 x 4 = 64$). It was confirmed that the genetic code involves three consecutive bases coding for an amino acid - the *triplet code*, with each group of three bases called a *base triplet.* The genetic code for each amino acid has been worked out and the 3-base DNA code (base triplet) for each of the 20 amino acids is now known. For example, the DNA sequence of \texttt{AAT} codes for the amino acid leucine and \texttt{GCG} codes for the amino acid arginine. ### Key features of the genetic code: * **Non-overlapping:** Each base in the DNA sequence is read only once. For example, \texttt{AATGCG} codes for leucine and arginine in consecutive positions. * **Degenerate:** Most amino acids have more than one possible code. With 64 possible sequences of three bases, there are duplicates for amino acids. For example, GGA, GGC, GGG and GGT all code for the amino acid proline. * Some base triplets do not code for an amino acid but terminate the coding sequence, they are called *stop base triplets*. Similarly, the sequence \texttt{TAC} codes for methionine but also acts as a *start triplet*. * **Universal:** With few exceptions, the DNA code is present in all living organisms. Chromosomes in the nucleus contain the genetic code, but protein synthesis takes place in the cytoplasm. Messenger RNA (mRNA) carries the code from the nucleus to the cytoplasm. Copying the code from DNA to mRNA is *transcription*. After transcription, the code is 'translated' into a polypeptide (protein). Protein synthesis consists of transcription followed by translation. ## Transcription Transcription is the process of forming complementary copies of mRNA from DNA sequences that code for a polypeptide or protein. ### Messenger RNA * **Why mRNA?** mRNA allows the DNA to remain in the Nucleus and prevents it from being damaged, also mRNA can make many copies of a single gene, and it is less metabolically volatile. * **Structure of mRNA:** mRNA is a single strand, unlike DNA (double helix). mRNA contains ribose instead of deoxyribose, and uracil instead of thymine. mRNA is a complementary copy of the template DNA strand and transports the DNA code from the nucleus to the cytoplasm. Each sequence of three bases along the mRNA strand codes for an amino acid as a *base triplet.* mRNA molecule codes for a relatively short section of DNA. Therefore, mRNA is shorter than DNA. **Main differences between mRNA and DNA:** | Feature | DNA | mRNA | | :---------------------- | :------------------------------------------------------------------------- | :---------------------------------------------------------- | | Relative size | Much longer (millions of nucleotides, 50-250 million base pairs in humans) | Much shorter (75-3000 nucleotides) | | Polynucleotide Arrangement | Double stranded (double helix) | Single stranded (single helix - not twisted) | | Pentose sugar | Deoxyribose | Ribose | | Nitrogenous bases | Adenine (A), guanine (G), cytosine (C), and thymine (T) | Adenine, guanine, cytosine, and uracil (U) | | Location | Mostly in nucleus (some in mitochondria and chloroplasts) | Produced in nucleus, found throughout cell (esp. with ER) | ### The process of transcription The enzyme DNA helicase separates the two DNA strands of the section of DNA to be copied by breaking the hydrogen bonds between the bases, unzipping the double helix. The enzyme RNA polymerase moves along the template (coding) strand linking the (now exposed) nucleotides in that strand with free complementary RNA (ribo) nucleotides from the nucleotide pool within the nucleus. The building up of the mRNA strand alongside its complementary DNA template strand follows the rules of complementary base pairing ensuring that the mRNA bases are aligned in sequence complementary to the template DNA strand. The RNA polymerase joins adjacent mRNA nucleotides to each other by phosphodiester bonds as the mRNA molecule extends. **Note:** During transcription normal C-G, G-C, T-A base pairing arrangements apply. The one exception is that an A base on the DNA template strand will be matched by a U (uracil) base on the mRNA strand. As the mRNA strand is assembled, increasing in length one nucleotide at a time, the unzipped DNA rejoins behind the assembly area. As a result only around 20 base pairs of the DNA are exposed at the one time. When the RNA polymerase reaches a 'stop' triplet code on the DNA, it detaches and the copying of this particular section of DNA is completed. ### Modification of the mRNA DNA is made of sections that code for polypeptide/protein (*exons*) and other sections (*introns*) that do not code for polypeptide or protein directly, but may regulate the activity of coding genes. In practice, introns are removed after transcription. Adjacent exons and introns are copied in the formation of the mRNA from the DNA template strand. Following transcription, the introns are removed from the pre-mRNA and the exons are spliced back together again to produce the coding sequence that actually codes for the required polypeptide or protein. **Note:** Following the removal of introns, the exons can be spliced back together in a range of different combinations allowing a gene to code for a number of different polypeptides or proteins depending on the order in which the exons are recombined. This explains the fact that the 21,000 genes in humans can code for the 100,000 different proteins that occur in the body. Following the removal of introns, the final version of the mRNA (the functional mRNA) moves out of the nucleus through a nuclear pore and into the cytoplasm where the actual formation of new proteins takes place, a process known as *translation*. ## Translation Translation involves the 'translation' of the mRNA code into the polypeptide primary structure (sequence of amino acids). Each sequence of three bases on the mRNA (the base triplet) that codes for a particular amino acid is referred to as a *codon*. As well as the coding mRNA, very important components of the translation process are transfer RNA (tRNA) and ribosomes. These two structures will be discussed in more detail in the next section. ### Transfer RNA and ribosomes tRNA is a small molecule consisting of around 70-80 nucleotides. It has a clover leaf shape. The two key parts are: * a sequence of three bases (*anticodon*) at one end that form complementary base pairs with a mRNA codon * the 'exposed' nucleotide section to which an amino acid can attach Enzymes help attach amino acids in the cytoplasm to the appropriate tRNA molecule. There is a different tRNA molecule for each of the 20 different types of amino acid. Each of the different types of tRNA has a particular anticodon that is unique to that type of tRNA, and also matches with a particular codon on the mRNA. The role of the tRNA is to: 1. Transport amino acids to the site of protein synthesis. 2. Ensure that each mRNA codon codes for a particular amino acid. Ribosomes - are small organelles (up to about 30 nm in diameter) that contain a large and a small sub-unit, that are made of protein and ribosomal RNA. They are assembled in the nucleolus within the nucleus and subsequently transported into the cytoplasm. At the start of translation the two sub-units link together as they lock on to the start of the mRNA strand to be copied. The ribosome contains two sites, each of which can cover a codon. The first codon-linking site is the *aminoacyl* (A) site and the second is the *peptidyl* (P) site. The A site, sometimes called the *acceptor site*, is where the tRNA molecules link together in the correct position on the mRNA strand through the linking of complementary anticodons and codons. The P site is where adjacent amino acids are linked together by peptide bonds. **Note: In summary**, the role of the ribosome is to hold the mRNA, the tRNA and the enzymes involved in protein synthesis in place. ## The process of translation The process of translation can be summarised as: * A ribosome attaches to the initial or *start codon* (AUG) on the mRNA by its A (acceptor) site. * The tRNA molecule with the complementary anticodon (UAC) moves to the ribosome and pairs up with its complementary codon (AUG) following complementary base pairing rules. This tRNA carries the amino acid methionine to the ribosome. Polypeptide chains usually begin with methionine. * As the ribosome moves along the mRNA to cover two codons, a tRNA molecule with a complementary anticodon pairs up with the next (second) codon on the mRNA strand. Again this tRNA carries its particular amino acid. By this stage the AUG (methionine) will now be in the P site, leaving the A site free for the second amino acid (serine in the diagram). * A *peptide bond* links the first two amino acids together to form a dipeptide. * As the ribosome notches along another codon, the tRNA for the methionine is set free and the tRNA for the second amino acid (serine) now occupies the P site. Again this leaves the A site free and the third amino acid (threonine) is brought into place by its tRNA molecule, again following base pairing rules. * The process continues and a polypeptide sequence of many amino acids is built up. * The process continues until a stop codon is reached. **Note 1:** Although AUG is the start codon necessary to start the translation process, not all polypeptides or proteins contain the amino acid methionine at the start. In many proteins the methionine is subsequently removed following translation. **Note 2:** Many ribosomes can operate in concert along the same strand of mRNA. This process is called a *polyribosome.* The following table shows a genetic dictionary - the mRNA codons involved in translation and the amino acids or actions they code for. | | U | C | A | G | | | :---- | :------------- | :------------ | :------------ | :------------ | :---- | | **U** | phenylalanine | serine | tyrosine | cysteine | **U** | | | phenylalanine | serine | tyrosine | cysteine | **C** | | | leucine | serine | stop | stop | **A** | | | leucine | serine | stop | tryptophan | **G** | | **C** | leucine | proline | histidine | arginine | **U** | | | leucine | proline | histidine | arginine | **C** | | | leucine | proline | glutamine | arginine | **A** | | | leucine | proline | glutamine | arginine | **G** | | **A** | isoleucine | threonine | asparagine | serine | **U** | | | isoleucine | threonine | asparagine | serine | **C** | | | isoleucine | threonine | lysine | arginine | **A** | | | methionine and start | threonine | lysine | arginine | **G** | | **G** | valine | alanine | aspartate | glycine | **U** | | | valine | alanine | aspartate | glycine | **C** | | | valine | alanine | glutamate | glycine | **A** | | | valine | alanine | glutamate | glycine | **G** | Differences between mRNA and tRNA: | Feature | mRNA | tRNA | | :---------------------- | :---------------------------------------------------------- | :--------------------------------------- | | Relative size | Larger molecule (75-3000 nucleotides) | Smaller molecule (70-80 nucleotides) | | Polynucleotide Arrangement | Single stranded (not twisted) | Clover shaped molecule | | Pentose sugar | Ribose | Ribose | | Nitrogenous bases | A, G, C, U | A, G, C, U | | Location | Produced in nucleus but found throughout cell (esp. with ER) | Produced in nucleus but found throughout cell | ## The one gene one polypeptide theory Francis Crick and James Watson worked out the structure of DNA around 60 years ago. Crick produced the 'central dogma of molecular biology' which can be summarised as: $DNA \implies RNA \implies PROTEIN $. or taking reverse transcriptase into account as: $DNA \implies RNA \implies PROTEIN $. DNA codes for a polypeptide or a particular polypeptide (the primary structure). Before the term 'one gene one polypeptide' was widely used, the terms 'one gene one protein' or 'one gene one enzyme' were used. The term 'one gene one protein' is appropriate for proteins with only one polypeptide. For proteins formed of more than one polypeptide, there is often a different gene that codes for each polypeptide. 'One gene one enzyme' may be regarded as a simplification because not all proteins are enzymes. DNA is able to control overall development in organisms through its control of enzyme activity. ## Epigenetics Epigenetics is the study of heritable modifications of the genome that do not involve changes to the DNA base sequence. Epigenetics affects gene activity (expression) without changing the DNA sequence. These changes are referred to as epigenetic modifications. **Note 1:** Epigenetic modification does not change the DNA sequence itself but increases or decreases the likelihood of a gene making a protein. **Note 2:** Mutations are not epigenetic modifications; mutations (permanently) alter the DNA sequence. Two important ways in which epigenetic modification occurs are DNA methylation and histone modification. *DNA methylation* - Methyl (CH3) can be added to cytosine (C) bases in the DNA in places where the C base is immediately followed by a G base (CpG sequences). If a critical number of CpG 'islands' are methylated, the DNA is switched off in that region and transcription cannot take place. DNA methylation is long term and it is stable. **Note:** The CG bases involved in methylation are in sequence along the coding strand DNA methylation can explain why proteins are only produced in specific parts of the body. In all other parts of the body, the genes involved are methylated and therefore 'switched off'. Epigenetic modifications involving DNA methylation can pass during mitosis. *Histone modification* - DNA is wrapped around bundles of eight histone proteins, The histones can be modified by adding chemical groups to them. Epigenetic modification involving histone modification is relatively short term and is important for both increasing and decreasing the expression levels of particular genes. Histone modification is more complex than DNA methylation. Many epigenetic modifications pass during mitosis. Epigenetics allows the environment to influence gene activity. ### Case Study - Holland in the winter of 1944-1945 During the final winter of the Second World War, Holland experienced near starvation conditions due to the Nazi blockade. Subsequent research showed that: * If near starvation coincided with the mother's last few months of pregnancy, the baby was likely to be born with a lower birthweight than normal. * If the mother suffered malnutrition only for the first few months, the baby was likely to have a normal birthweight. Further research showed that malnourished babies in early pregnancy were likely to be overweight or obese as adults. Explanation: Many genes were not switched off in malnourished children, remaining on through life. Note: Conditions very early in development can have lifelong consequences. Epigenetic modifications also occur in plants. In low light there is increased gene expression in light harvesting genes while there is also decreased expression in genes associated with reproduction (sacrificing reproduction for growth). **Note 1:** Epigenetic modification significantly increases flexibility. **Note 2:** Epigenetic modifications can change as an individual ages, and between tissues.