Energy Storage Systems PDF
Document Details
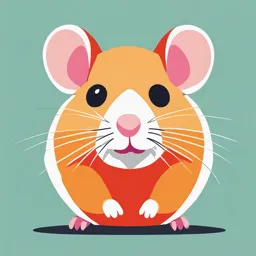
Uploaded by FresherHeliotrope6307
Ontario Tech University
Dr. Ibrahim Dincer
Tags
Summary
This document provides an outline of energy storage techniques, including thermal energy storage methods, applications, and technical aspects of cold TES systems. It details case studies related to energy storage and includes a deep dive into distributed energy storage.
Full Transcript
Faculty of Engineering and Applied Science MECE3260U-Introduction to Energy Systems Energy Storage Systems Dr. Ibrahim Dincer Professor of Mechanical Engineering OUTLINE Introduction Energy Storage Techniques Thermal Energy Storage (TES) Metho...
Faculty of Engineering and Applied Science MECE3260U-Introduction to Energy Systems Energy Storage Systems Dr. Ibrahim Dincer Professor of Mechanical Engineering OUTLINE Introduction Energy Storage Techniques Thermal Energy Storage (TES) Methods TES Applications Technical Aspects of Cold TES Systems Case Studies Closing Remarks 2 https://www.huntkeyenergystorage.com/distributed-energy-storage/ 3 WHY ENERGY STORAGE? One of the advanced energy technologies. One of the potential solutions for reducing the environmental impact. An efficient and effective energy saving method. Important mechanism to offset the mismatch of availability and demand. An enormous potential for an effective use of thermal energy equipment and for facilitating large-scale energy substitutions in the economic perspective. Application possibility in several sectors of energy systems. 4 SOME ENERGY STORAGE TECHNIQUES Pumped storage Electro-chemical batteries Flywheels Compressed air Biological storage Magnetic storage Chemical storage THERMAL STORAGE 5 6 https://www.sandia.gov/ess-ssl/lab-pubs/doeepri-electricity-storage-handbook/ 7 Compound annual growth rate: 8 https://www.nature.com/articles/nenergy2017110 (by Schmidt et al.) 9 https://www.visualcapitalist.com/sp/countries-ranked-by-battery-capacity-in-2023/ 10 https://www.storagevet.com/home/ 11 Compressed Air Energy Storage System https://www.oilfree-air.eu/compressed-air-energy-storage-caes/ 12 Pumped Hydro Storage System Source: http://www.ee.co.za/article/small-pumped-water- storage-systems-new-partner-renewable-energy.html 13 Source: Journal of Solar Energy Engineering 14 TES PERIODS Temporary storage of high- or low-temperature energy for later use. Short-term storage (e.g., diurnal): storage of solar energy for overnight heating storage of heat and coolness generated electrically during off-peak hours for use during subsequent peak hours Long-term storage (e.g., seasonal): storage of summer heat for winter use 15 TES METHODS Sensible heat storage (SHS): By increasing the temperature of storage medium temperature without phase change. Example storage media: water tanks, rock bins, earth beds, rock beds, solar ponds, aquifers. Latent heat storage (LHS): By phase change of storage materials (so-called: phase change materials or PCMs). Examples: salt hydrates and organic materials. 16 BTES Features: Heat transfer fluid: a 15% glycol/water mix, circulating through over 150 km of 4” polypropylene piping which was pressure tested to 300 psi. Summer heat absorbed from the geothermal loop into the ground during summer is drawn in the winter to provide low temperature hydronic heating at 52°C. Biggest drawback was the higher capital cost for high efficiency HVAC equipment and geothermal field. UOIT Borehole Annual energy savings: 40% in heating and over 20% in cooling. Thermal Storage System (BTES) Payback on high efficiency HVAC equipment: 3 to 5 years. Payback on well field: 7.5 years. Mechanical rooms: 2 (one for boiler system and geothermal pumps and one for chillers). Two Multistack chillers (each having seven 9-ton modules) and two sets of Multistack geothermal heat pumps with seven 50-ton modules each. 17 SOME APPLICATIONS Macro-Scale Application: A unique borehole TES at UOIT UOIT BTES Borehole grids and interconnecting piping 18 SELECTION CRITERIA FOR TES Storage duration (short- or long-term) Technical availability Integrability with other thermal system(s) Reliability Applicability Commercial viability Cost Efficiency Environmental impact Operating strategy Operating conditions etc. 19 (a) Conventional System Schematic representation of two building Chiller Distribution Building cooling systems: (a) conventional system and (b) TES system. (b) TES System Chiller Distribution Building Storage Charging Storing Discharging The three processes in a general CTES system: charging (left) storing (middle) and discharging (right). The heat leakage into the system Ql is illustrated for the storing process, but can occur in all three processes. 20 BUILDING LOAD (%) Comparison of conventional and TES systems for 100 electricity use for cooling 75 50 25 Operating Strategies: 0 TIME a) Full-storage, 6:00am 12:00am 6:00pm 12:00pm b) partial-storage load levelling, and Conventional (without storage) c) partial-storage demand-limiting. Full storage Near full storage Load Partial storage Chiller on B Chiller meets load directly A Storage meets load C Chiller charging storage Reduced on-peak demand Tons Tons Tons C C A A A C C C B C B B B 24-hour period 24-hour period 24-hour period (a) (b) (c) 21 Chiller Storage Distribution Some major cold TES cooling system types Conventional Chilled water Conventional water Conventional Eutectic salt/water solution Conventional water Ice-making Ice Conventional water Ice-making Ice Cold air Ice-making Ice Unitary (Rooftop) The capsules are filled with deionized water and a nucleating agent and stored in a tank. A glycol/water brine solution is circulated around the capsules. (From CRYOGEL) 22 https://thermtest.com/phase-change-material-pcm 23 Thermal Management of Batteries Battery Technology: Lithium-ion Batteries Specific Energy Specific Power Battery (Wh/kg) (W/kg) Pb-acid 30 – 40 80 – 300 NiCd 50 – 60 200 – 500 NiMH 60 – 70 200 – 1500 Li-ion 60 – 150 800 – 2000 1. Refrigeration Cycle TMS 2. Cabin Air TMS 3. Passive TMS with PCM 24 25 SAVINGS BY TES SYSTEMS Utilization of waste or surplus energy: To reduce the consumption of purchased energy by storing waste or surplus thermal energy available at certain times for use at other times, e.g., solar energy storage during the day for heating at night. Reduction of demand charges: To reduce the demand of purchased electrical energy by storing electrically produced thermal energy during off-peak periods to meet the thermal loads that occur during high demand periods. Deference to equipment purchases: To defer the purchase of additional equipment for thermal applications and reduce the equipment size/capacity in new facilities. 26 CASE STUDIES Objectives: To conduct energy, exergy and exergoeconomic analyses of various TES systems. To develop energy and exergy efficiency models, depending on the measures of merit desired. To compare the calculated energy and exergy efficiencies with actual TES data and evaluate them for different operating conditions/parameters. To interpret the results, and draw appropriate conclusions and recommendations, relating to such issues as design changes, retrofit plant modifications, etc. 27 Case Study 1: Actual load profile of the CTES system (Carrier, US). 28 Actual data and comparison between energy and exergy efficiencies Hour Process Load (Tons*) Melted Efficiency (%) fraction (%) Storage Building Chiller Exergy Energy 1 Charging 270 0 270 48.55 88.1 99.7 2 Charging 270 0 270 41.46 87.0 99.7 3 Charging 270 0 270 34.36 85.9 99.7 4 Charging 270 0 270 27.27 84.8 99.7 5 Charging 270 0 270 20.17 83.7 99.7 6 Charging 270 0 270 13.08 82.6 99.7 7 Charging 270 0 270 5.99 81.6 99.7 8 Charging 170 100 270 1.53 80.4 99.5 9 Storing 0 385 385 1.55 99.9 99.9 10 Discharging 175 580 405 6.12 66.0 99.7 11 Discharging 375 780 405 15.96 63.3 99.9 12 Discharging 490 895 405 28.83 59.9 99.9 13 Discharging 635 1040 405 45.53 58.5 99.9 14 Discharging 670 1075 405 63.15 57.1 99.9 15 Discharging 685 1090 405 81.16 55.7 99.9 16 Discharging 475 880 405 93.63 52.9 99.9 17 Discharging 175 580 405 98.21 63.9 99.7 18 Storing 0 380 380 98.22 99.9 99.9 19 Charging 270 0 270 91.13 93.5 99.7 20 Charging 270 0 270 84.03 92.6 99.7 21 Charging 270 0 270 76.94 91.8 99.7 22 Charging 270 0 270 69.84 90.9 99.7 23 Charging 270 0 270 62.74 90.1 99.7 24 Charging 270 0 270 55.65 89.3 99.7 * 1 Ton of refrigeration = 3.517 kW. Rosen and Dincer (2002) 29 Case Study 2: 30 An ice-slurry system (Courtesy of Mueller) Case Study 3: Operation: A compressor/condenser (1) supplies refrigerant to the evaporator. An ice orbital rod evaporator (2) uses a freeze-depressant solution to produce a pumpable ice slurry. (Low- temperature slurry is ideal for use with low-temperature air systems.) An insulated ice storage tank (3) separates ice manufacturing from ice usage. The tank contains a freeze- depressant solution which is converted to an ice slurry in the evaporator. The slurry melts as the stored ice absorbs the heat of the cooling load. A load control pump and valve (4) control the supply temperature to the load. A plate heat exchanger (5) separates the storage tank from the cooling load and prevents cross-contamination between the ice-melting loop and the cooling load. The solution can be supplied from the ice storage tank to the load at various temperatures to satisfy specific application needs. 31 90 10 Tamb=30 [C] ─ Tamb = 20°C ─ Tamb = =30 Tamb 20°C[C] 80 -- Tamb = 30°C Tamb =20 [C] -- Tamb T = 30°C =20 [C] amb 5 70 Variation of storage temperature Ts with time t 60 0 d [%] 50 Ts [C] -5 40 -10 30 Variation of energy efficiency of storage tank with time 20 -15 10 -20 0 0 4 8 12 16 20 24 0 4 8 12 16 20 24 t [hour] t [hour] 30 16 Tamb=30 [C] Tamb ─ Tamb = =30 20°C[C] ─ Tamb = 20°C 25 -- Tamb = 30°C 14 -- Tamb = 30°C Tamb =20 [C] Tamb =20 [C] 12 20 system [%] 10 tank [%] 15 8 Variation of exergy efficiency of overall system with time 10 Variation of exergy efficiency of storage tank with time 6 4 5 2 0 0 0 4 8 12 16 20 24 0 5 10 15 20 25 32 t [hour] t [hour] Case Study 4: Annual energy savings and emission reductions*. Commodity Conventional system TES-based system Reduction for TES-based system Consumptions Natural gas (m3) 215,800 95,500 120,300 (56%) Electricity (kWh) 395,550 511,500 -84,000 (-21%) Primary energy (m3)a 322,000 179,000 143,000 (44%) Emissions CO2 (kg) 608,000 346,000 262,000 (43%) NOx and SO2 -- -- -- (40%) a Primary energy is calculated as the equivalent amount of natural gas based on the assumption that 3 0.25 m gas is used in the generation of 1 kWh electricity. * Adapted from IEA , where further details are available. 33 CLOSING REMARKS TES plays a significant role in meeting society's need for efficient, environmentally benign energy use, and offsets the mismatch between supply and demand for thermal energy. TES is one of the most significant energy conservation technologies, particularly for building HVAC applications. It is very critical for renewable energy systems. Exergy analysis is a key tool for design, analysis, optimization, and performance improvement of TES systems. 34 35