Lecture 2: Embryonic Stem Cells PDF
Document Details
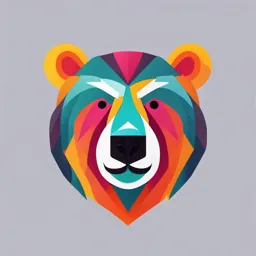
Uploaded by VeritableAmber980
Monash University
Tags
Related
- Intro to Haematopoietic System Part 1 PDF
- FFP1-Cellular Differentiation and Stem Cells PDF
- 2024 Stem Cells, Cell Asymmetry, and Cell Death Notes (BIOL 374)
- Umbilical Cord Stem Cells PDF
- Lecture 37 Second & Third Weeks of Development PDF
- Cellular Mechanisms of Development - Chapter 10: Pregnancy, Development, and Aging
Summary
Lecture 2 details the properties of stem cells, focusing on their self-renewal and differentiation capacities. Different types of stem cells, including totipotent, pluripotent, and multipotent cells, are described along with their potential, source, and examples. The lecture also explores important aspects like embryonic carcinoma (EC) cells and their role in understanding pluripotent cells.
Full Transcript
🥽 Lecture 2: Embryonic stem cells LO: What makes a stem cell a stem cell? Stem cell two properties: 1) Divides and produces identical daughter cells = “self-renewal” 2) Develops into many cell t...
🥽 Lecture 2: Embryonic stem cells LO: What makes a stem cell a stem cell? Stem cell two properties: 1) Divides and produces identical daughter cells = “self-renewal” 2) Develops into many cell types = “specialises” or “differentiates” A stem cell is not terminally differentiated (ie. defined as one that, in the course of acquiring specialised functions, has irreversibly lost its ability to proliferate) It has limitless divisions Asymmetric division results in daughter cells that can become either - stem cells (renewal) OR undergo terminal differentiation (progenitor, or transit amplifying cells) Lecture 2: Embryonic stem cells 1 How can division lead to different fates? Environmental asymmetry External signals direct/influence the fate of the daughter cells Niche can regulate whether a stem cell will self-renew or differentiate, depending on the needs of the tissue Divisional asymmetry Stem cells have internal asymmetry, possessing unevenly distributed cellular components and determinants Daughters receive different internal determinants when dividing LO: The hierarchy of potency Lecture 2: Embryonic stem cells 2 Totipotent cells Potential - highest potency, ie. can differentiate into any cell type in the body, as well as extra-embryonic tissues (like the placenta) Source - zygote Example - zygote (fertilised) Pluripotent cells Potential - can differentiate into almost any cell within the body, including all the cells of the nervous system, but not into extra- embryonic tissues Source - embryonic stem cells derived from inner cell mass of a blastocyst Example - embryonic stem cells Multipotent cells Lecture 2: Embryonic stem cells 3 Potential - can differentiate into a limited range of cell types within a specific tissue or organ Source - embryo adult brain Example - neural stem cells (NSCs); neurons, astrocytes, oligodendrocytes Unipotent cells Potential - capable of differentiating into only one specific cell type Source - regions of the brain Example - neuronal progenitor, etc. LO: Different types of pluripotent stem cells (eg. EC, EG/EpiES, SCN/iPS) Embryonic carcinoma (EC) EC cells are derived from teratocarcinomas - a type of tumour that often forms in the testes or ovaries EC cells are often aneuploidy (abnormal no. of chromosomes) Before the development of human ES cells, EC cells were used to the characteristics of pluripotent cells Teratomas - tumours that can form from parthenogenetically activated oocytes (egg cells that start to divide without being fertilised) Recapitulate development in a disorganised manner (containing a variety of tissue types like hair, muscle, bone) In the ovary = teratomas are generally benign (non-cancerous) In the testes = teratomas become malignant in the testes and are then referred to as teratocarcinomas These tumours contain both differentiated tissues and undifferentiated EC cells, which are believed to be the stem cells of the tumour. Lecture 2: Embryonic stem cells 4 Embryonic germ (EG) cells Embryonic germ (EG) cells - pluripotent stem cells isolated from cultured mouse primordial germ cells (PGCs) PGCs - found in early embryo and eventually develop into sperm or eggs (precursors to gametes) Like embryonic stem cells - EG cells are pluripotent and can differentiate into cells of all 3 germs layers (ectoderm, mesoderm, and endoderm) EG cells can retain some of the germ cell characterisitcs, being able to erase imprinted genes Culture requirement Mouse EG cells are cultured on feeder cell layers, similar to ES cells, to provide the necessary support for their growth In addition to leukemia inhibitory factor (LIF), which is commonly used to maintain the undifferentiated state of mouse pluripotent stem cells, EG cells also require fibroblast growth factor 2 (FGF-2) and Steel factor (also known as stem cell factor, SCF) to proliferate and remain undifferentiated in culture Lecture 2: Embryonic stem cells 5 Human EG cells are less well characterised than human ES cells, but appear to have similar differentiation capacity EG cells taken from fetuses from terminated pregnancy give info about early human reproductive development Somatic Cell Nuclear Transfer (SCNT) In SCNT - the nucleus of a somatic cell (a non-reproductive cell, eg. skin, or a diseased cell that could be used for study of the disease) is transferred into an enucleated oocyte The oocyte, now containing the somatic cell nucleus, is stimulated to begin dividing and develop into a blastocyst Lecture 2: Embryonic stem cells 6 From the blastocyst (5-7 days old, longer than that is illegal), the pluripotent stem cells can be harvested These SCNT-derived stem cells are genetically identical to the donor of the somatic cell nucleus, making them useful for personalised medicine and potentially for therapeutic cloning Cloned human embryos as a source of ES cells In this process, the nucleus of a somatic cell is transferred into an enucleated egg cell, which is then stimulated to develop into a blastocyst (an early-stage embryo). From this blastocyst, ES cells can be harvested for research purposes ⇒ SCNT Positives: Self-Renewing Supply: Provides a scalable, renewable source of pluripotent stem cells. Donor Consent: Obtaining donor tissue is relatively straightforward with consent. Patient-Matched Cells: Enables the creation of cells that are genetically identical to the donor, reducing the risk of immune rejection. Lecture 2: Embryonic stem cells 7 Disease Modeling: Useful for creating in vitro models of diseases and exploring potential treatments. Mitochondrial Disorders: Offers potential solutions for disorders involving mitochondrial DNA through techniques like "3-parent embryos." Negatives: Oocyte Requirement: Requires human oocytes, which are difficult to obtain. Efficiency Issues: Reprogramming and expanding cells to the blastocyst stage is inefficient. Ethical Concerns: The creation and destruction of embryos raise significant ethical issues. Reproductive Cloning: There are concerns that the technology could be misused for reproductive cloning, which is widely considered unethical. LO: The basic processes of derivation, culture, and differentiation requirements Embryonic stem cells - processes of derivation, culture, and differentiation requirements Where do human embryonic stem cells come from? Lecture 2: Embryonic stem cells 8 Isolation and culture of ICM from human embryos Zona pellucida digested with pronase, making it easy to access the ICM within the blastocyst Zona pellucida is a glycoprotein layer surrounding the blastocyst - protecting the embryo Immunosurgery is carried out: Lecture 2: Embryonic stem cells 9 Anti-human serum antibody followed by guinea pig complement to remove trophectoderm (while leaving the ICM intact) This causes the immune system to attack and remove the triphectoderm cells, leaving the ICM exposed Isolation of the ICM destroys the human embryo The inner mass cells cultured on mitotically inactivated mouse embryonic fibroblast (MEF) feeder cells MEF - these feeder cells are treated so that they do not divide, but provide essential growth factors and a supportive environment for the ICM cells to grow & maintain their pluripotency Characteristics properties of laboratory-derived human embryonic stem (hES) cells Can be grown indefinitely in the lab in an unspecialised state, ie. self- renewing Maintain a normal diploid karyotype Ie. maintaining a normal diploid karyotype have the correct & stable number of chromosomes (46 in humans) - Capable of differentiation into a wide range of somatic and extraembryonic tissues in vivo and in vitro Immunologically matched to the embryo from which the cells were originally isolated When introduced to immunodeficient mice (so their immune system doesn’t attack the hES) can generate tumours that contain all cells that represent the developing embryo This is a teratoma assay, NOT chimera generation as it is legally restricted in many countries, including Aus) hES-drived tissue cells can restore function in animal models following transaplantation Lecture 2: Embryonic stem cells 10 Culturing Human ES Cells & Differentiation Requirements Subculturing/passaging Refers to the process of transferring a small portion of a cell culture to a new culture dish to allow continued growth - for hES cells, this process is done weekly to maintain the culture and prevent overcrowding Cultivation on MEF Feeder Layers hES cells are typically grown on a layer of MEF MEFs are treated to prevent them from dividing - ensuring they do not overgrow the hES cells but still provide the necessary support Culture medium: DMEM, 20% FCS, 0.1 mM β-mercaptoethanol, 1% non- essential amino acids, 2 mM glutamine + pen./ strep Subculture weekly as clumps rather than single cells (unlike mESCs!) Helps maintain the hES cells’ undifferentiated state, as dissociating them into single cells can lead to differentiation or cell death Differentiated cells are mechanically dissected from undifferentiated cells Lecture 2: Embryonic stem cells 11 LO: Key assessments for pluripotency Assessing the pluripotency of stem cells involve evaluating their ability to differentiate into various cell types & their capacity to contribute to all tissues of an organism: 1) Cloned cell lines and differentiation Stem cells that are cloned & expanded in culture → capable of differentiation into a wide range of somatic & extraembryonic tissues in vivo and in vitro - at high frequency and under a range of conditions 2) Capable of colonising all tissues (including germ line) after blastocyst injection If the injected cells contribute to various tissues in the offspring, the organism is referred to as a chimera. This method is a gold standard for assessing pluripotency in mice. However, due to ethical concerns, this approach is not applied to human cells. 3) Tetraploid embryo complementation (mouse ES only) A tetraploid embryo (with twice the usual number of chromosomes) is created, which cannot develop into a viable organism on its own. When pluripotent stem cells are injected into this embryo → they can compensate and form a fully developed organism Successfully rescuing a tetraploid embryo in this way demonstrates the complete pluripotency of the stem cells. 4) Teratoma formation (mouse and human ES cells) When pluripotent stem cells are injected into immunocompromised mice, they can form teratomas, which are benign tumors containing differentiated cells from all three germ layers. The presence of a variety of tissue types within the teratoma confirms the pluripotency of the stem cells. Lecture 2: Embryonic stem cells 12 Characterising pluripotent stem cells in vitro (in a laboratory setting): Morphology - cell and colony size/shape/behaviour Pluripotent stem cells typically have high nucleus-to-cytoplasm ratio Grow in colonies that are usually tightly packed, with smooth and well-defined edges Proliferate rapidly while maintaining their undifferentiated state Gene expression - transcript found in pluripotent stem cell populations Many code for proteins with important roles in embryonic development (eg. OCT3/4, Nanog, Cripto, FoxD3) Immunological markers Protein expression for markers associated with pluripotency Biological properties Differentiation into derivatives of all three germ layers LO: The differences between human and mouse ESCs In vivo differentiation of human ES cells Ability of a human ES cell line to form teratomas is the best test of pluripotentiality presentialy available - “gold standard” In vivo teratoma assay Xenografts in SCID mice → implant cells for testing beneath the testis capsule of SCID mice Animals are monitored weekly for tumour development Tumours are removed, fixed in formalin, and sent for histology Assess histological sections for representatives of the germ layers → the process is labour intensive, cumbersome, but is the “gold standard” In the future may be superseded by genomic/bioinformatic platforms Lecture 2: Embryonic stem cells 13 hES cells give rise to disorganised growths called teratomas (when differentiating outside of the controlled environment of an embryo) Unlike in normal embryonic development, teratomas formed from hES cells do not display axis formation or segmentation Without the guidance of the embryonic environment, hES cells cannot establish these axes or segmentation, leading to the disorganized nature of the growth. This lack of organisation is also when ES cells differentiate in vitro Teratomas contain tissues representative of the three embryonic germ layers LO: The hurdles that need to be overcome for stem cell therapeutics to be possible 1) Culture conditions Growth of hES cells in a clinically acceptable wau No exposure to non-human proteins in serum Lecture 2: Embryonic stem cells 14 Such as animal serums, risk of disease contaminant like prion in the early 2000s Good Manufacturing Practice (GMP) guidelines - dictate how cells are cultured, handled, and prepared for transplation 2) Identification and purification of the “right” cells Removal of all undifferentiated hES cells (avoid forming teratoma) Positive selection of differentiated cell type Depletion of undifferentiated stem cells 3) Recipient response Immune rejection of transplated cells or tissue Defined culture systems for propagating hES cells - focusing on creating controlled and consistent environments for stem cell research and clinical applications Semi-defined or defined and xeno-free systems: Semi-defined or defined systems refer to culture environments where most, if not all, of the components are known and controlled, reducing variability. Xeno-free systems are designed to avoid using animal-derived products Opting for using human feeders, serum, albumin Defined & scalable culture systems being trialled Defined culture systems: These involve the use of fully known and controlled components, including chemically defined conditions and small molecules, to precisely regulate stem cell growth. Scalable systems: Designed to be used on a large scale, these systems are important for producing enough cells for clinical applications. Very important for clinical translation of the field Karyotypic abnormalities: Over time, stem cells can accumulate genetic changes. Monitoring these changes is essential to ensure the safety and stability of cells, especially when they are used for therapeutic purposes. Lecture 2: Embryonic stem cells 15 Assessment of pluripotency over long term passage LO: The ethical and legislative implications for the use of human pluripotent stem cells in research, and ultimately the clinic FDA requirements Lecture 2: Embryonic stem cells 16