Physiology: Blood Flow, Pressure, and Resistance (PDF)
Document Details
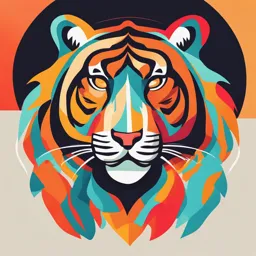
Uploaded by HealthyDragon
Université de Montréal
Tags
Summary
This document explains the relationships between blood flow, pressure, and resistance in the cardiovascular system. It introduces important concepts for understanding these relationships using analogies to electrical circuits and the Poiseuille equation. It also details the concept of vascular compliance and how blood flows as described by stroke volume and cardiac output.
Full Transcript
# Physiology ## Relationships Between Blood Flow, Pressure, and Resistance * Blood flow through a blood vessel is determined by the pressure difference between the two ends of the vessel and the resistance of the vessel to blood flow. * The pressure difference is the driving force for blood flow,...
# Physiology ## Relationships Between Blood Flow, Pressure, and Resistance * Blood flow through a blood vessel is determined by the pressure difference between the two ends of the vessel and the resistance of the vessel to blood flow. * The pressure difference is the driving force for blood flow, and the resistance is an impediment to flow. * The relationship of flow, pressure, and resistance is analogous to the relationship of current $(I)$, voltage $(AV)$, and resistance $(R)$ in electrical circuits as expressed by Ohm's Law: $AV = I \times R$ or $I = AV/R$. * Blood flow is analogous to current flow, the pressure difference or driving force is analogous to the voltage difference, and hydrodynamic resistance is analogous to electrical resistance. * The equation for blood flow is expressed as follows: $Q = AP/R$ where: * $Q = Flow (mL/min)$ * $AP = Pressure difference (mm Hg)$ * $R = Resistance (mm Hg/mL/min)$ * The magnitude of blood flow $(Q)$ is directly proportional to the size of the pressure difference $(AP)$ or pressure gradient. * The direction of blood flow is determined by the direction of the pressure gradient and always is from high to low pressure. * Blood flow is inversely proportional to resistance $(R)$. Increasing resistance (e.g., by vasoconstriction) decreases flow, and decreasing resistance (e.g., by vasodilation) increases flow. * The major mechanism for changing blood flow in the cardiovascular system is by changing the resistance of blood vessels, particularly the arterioles. * The flow, pressure, and resistance relationship can be rearranged to determine resistance. If the blood flow and the pressure gradient are known, the resistance is calculated as $R = AP/Q$. * This relationship can be used to measure the resistance of the entire systemic vasculature (i.e., total peripheral resistance), or it can be used to measure resistance in a single organ or single blood vessel. * **Total peripheral resistance** - the resistance of the entire systemic vasculature is called the total peripheral resistance $(TPR)$ or the systemic vascular resistance $(SVR)$. * **Resistance in a single organ** - the flow, pressure, and resistance relationship can be applied on a smaller scale to determine the resistance of a single organ. ## Resistance to Blood Flow * The blood vessels and the blood itself constitute resistance to blood flow. * The relationship between resistance, blood vessel diameter (or radius), and blood viscosity is described by the Poiseuille equation: $R = \frac{8n l}{\pi r^4}$ where: * $R = Resistance$ * $n = Viscosity of blood$ * $l = Length of blood vessel$ * $r^4 = Radius of blood vessel raised to the fourth power$ * The most important concepts expressed in the Poiseuille equation: 1. Resistance to flow is directly proportional to viscosity $(n)$ of the blood. 2. Resistance to flow is directly proportional to the length $(l)$ of the blood vessel. 3. Resistance to flow is inversely proportional to the fourth power of the radius $(r^4)$ of the blood vessel. ## Compliance of Blood Vessels * The compliance or capacitance of a blood vessel describes the volume of blood the vessel can hold at a given pressure. * Compliance is related to distensibility and is given by the following equation: $C = V/P$ where: * $C = Compliance (mL/mm Hg)$ * $V = Volume (mL)$ * $P = Pressure (mm Hg)$ * The equation for compliance states that the higher the compliance of a vessel, the more volume it can hold at a given pressure. * For each type of blood vessel, volume is plotted as a function of pressure. * The slope of each curve is the compliance. * Compliance of the veins is high. * Compliance of the arteries is much lower than that of the veins. * The difference in the compliance of the veins and the arteries underlies the concepts of unstressed volume and stressed volume. * **Unstressed volume** - veins are most compliant and contain the unstressed volume (a large volume under low pressure). * **Stressed volume**- arteries are much less compliant and contain the stressed volume (low volume under high pressure). * The total volume of blood in the cardiovascular system is the sum of the unstressed volume plus the stressed volume (plus whatever volume is contained in the heart). * Changes in compliance of the veins cause redistribution of blood between the veins and the arteries (i.e., the blood shifts between the unstressed and stressed volumes). * If the compliance of the veins decreases, there is a decrease in the volume the veins can hold, and, consequently, a shift of blood from the veins to the arteries: unstressed volume decreases and stressed volume increases. * If the compliance of the veins increases, there is an increase in the volume the veins can hold and, consequently, a shift of blood from the arteries to the veins: unstressed volume increases and stressed volume decreases. ## Pressures in the Cardiovascular System * Blood pressures are not equal throughout the cardiovascular system.If they were equal, blood would not flow, since flow requires a driving force (i.e., a pressure difference). * The pressure differences that exist between the heart and blood vessels are the driving force for blood flow. | Location | Mean Pressure (mm Hg) | |---|---| | **Systemic** | | | Aorta | 100 | | Large Arteries | 100 (systolic, 120; diastolic, 80) | | Arterioles | 50 | | Capillaries | 20 | | Vena Cava | 4 | | Right Atrium | 0-2 | | **Pulmonary** | | | Pulmonary Artery | 15 (systolic, 25; diastolic, 8) | | Capillaries | 10 | | Pulmonary Vein | 8 | | Left Atrium | 2-5 | * Pressures on the left side of the heart are difficult to measure directly. However, left atrial pressure can be measured by the pulmonary wedge pressure. With this technique, a catheter is inserted into the pulmonary artery and advanced into a small branch of the pulmonary artery. The catheter wedges and blocks all blood flow from that branch. Once the flow is stopped, the catheter senses the pressure in the left atrium almost directly. ## Pressure Profile In The Vasculature * Figure 4-8 is a profile of pressures within the systemic vasculature. * Mean pressure is highest in the aorta and large arteries and decreases progressively as blood flows from the arteries, to the arterioles, to the capillaries, to the veins, and back to the heart. * Mean pressure in the aorta is very high, averaging 100 mm Hg. This high mean arterial pressure is a result of two factors: the large volume of blood pumped from the left ventricle into the aorta (cardiac output) and the low compliance of arterial wall. * The pressure remains high in the large arteries, which branch off the aorta, because of the high elastic recoil of the arterial walls. * Beginning in the small arteries, arterial pressure decreases, with the most significant decrease occurring in the arterioles. * Arterioles constitute a high resistance to flow. * In the capillaries, pressure decreases further for two reasons: frictional resistance to flow and filtration of fluid out of the capillaries. * Pressure has decreased even further when blood reaches the venules and veins. * Pressure in the vena cava is only 4 mm Hg, and in the right atrium is even lower at 0 to 2 mm Hg. **Arterial Pressure in the Systemic Circulation** * Further examination of Figure 4-8 reveals that although mean arterial pressure is high and constant, there are oscillations or pulsations of arterial pressure. * These pulsations reflect the pulsatile activity of the heart. * Each cycle of pulsation in the arteries coincides with one cardiac cycle. **Diastolic pressure** * The lowest arterial pressure measured during a cardiac cycle. **Systolic pressure** * The highest arterial pressure measured during a cardiac cycle. **Pulse pressure** * The difference between systolic pressure and diastolic pressure. **Mean arterial pressure** * The average pressure in a complete cardiac cycle. * Calculated by the following formula: $Mean arterial pressure = Diastolic pressure + (1/3) Pulse pressure$ * Mean arterial pressure is not the simple mathematical average of diastolic and systolic pressures. This is because a greater fraction of each cardiac cycle is spent in diastole than in systole. * Thus, the calculation of mean arterial pressure gives more weight to diastolic pressure than systolic pressure. * The pulsations in large arteries are even greater than the pulsations in the aorta. * Systolic pressure and pulse pressure are higher in the large arteries than in the aorta. * Pulse pressure will change if stroke volume changes, or if the compliance of the arteries changes. * Arteriosclerosis - plaque deposits in arterial walls decrease the diameter of the arteries and make them stiffer and less compliant. * Systolic pressure, pulses, and mean pressure are increased. * Aortic stenosis - a stenosed aortic valve, which reduces the opening through which blood can be ejected from the left ventricle into the aorta. * Stroke volume, systolic pressure, pulse pressure, and mean pressure are decreased. * Aortic regurgitation - an incompetent aortic valve, which disrupts the normal one-way flow of blood from the left ventricle into the aorta. * Blood flows backward into the ventricle. ## Autonomic Effects on the Heart and Blood Vessels * The autonomic nervous system effects are called chronotropic effects. Sympathetic stimulation increases heart rate, and parasympathetic stimulation decreases heart rate. * **Positive chronotropic effects** - increases in heart rate. * Stimulated by the sympathetic nervous system. * Norepinephrine activate B1 receptors in the SA node. * Increases the rate of phase. 4 depolarization. * **Negative chronotropic effects** - decreases in heart rate. * Stimulated by the parasympathetic nervous system. * Acetylcholine activates muscarinic (M2) receptors in the SA node. * Decreases the rate of phase 4 depolarization. * Increases the outward K+ current (similar to IK1) called IK-Ach. ## Autonomic Effects on Conduction Velocity in the Atrioventricular Node * The effects of the autonomic nervous system on conduction velocity are called dromotropic effects. * **Positive dromotropic effects** - increases in conduction velocity. * Stimulated by the sympathetic nervous system. * Increases Ica, which is responsible for the upstroke of the action potential in the AV node. * **Negative dromotropic effects** - decreases in conduction velocity. * Stimulated by the parasympathetic nervous system. * Decreases Ica (decreased inward current) and increases IK-Ach (increased outward K+ current). ## Stroke Volume, Ejection Fraction, and Cardiac Output * The function of the ventricles is described by the following three parameters: 1. **Stroke volume** - the volume of blood ejected by the ventricle on each beat. 2. **Ejection Fraction** - the fraction of the end-diastolic volume that is ejected in one stroke volume. 3. **Cardiac output** - the total volume ejected by the ventricle per unit time. * Stroke volume is the difference between the volume of blood in the ventricle before ejection (end-diastolic volume) and the volume remaining in the ventricle after ejection (end-systolic volume). * Ejection Fraction is a measure of ventricular efficiency. * Cardiac output is the product of stroke volume and heart rate. ## Frank-Starling Relationship ## Myocardial Oxygen Consumption * Myocardial O2 consumption correlates directly with cardiac minute work. * Of the two components of cardiac minute work, in terms of O₂ consumption, pressure work is far more costly than volume work. * Overall myocardial O2 consumption correlates poorly with cardiac output. ## Integrative Functions of The Cardiovascular System * The cardiovascular system always operates in an integrated manner. * The cardiovascular system response to exercise is a combination of central nervous system (CNS) and local mechanisms. * Local responses in the exercising skeletal muscle are orchestrated by active hyperemia. ## Overall Responses to Exercise * The cardiovascular system response to exercise is a combination of the central command and the effects of local metabolites. The central command directs the sympathetic nervous system to increase heart rate and stroke volume, thereby increasing cardiac output. * Decreased parasympathetic outflow and increased sympathetic outflow produce vasoconstriction in several vascular beds (excluding exercising skeletal muscle, coronary, and cerebral circulations). * Local metabolites produce vasodilation in the exercising skeletal muscle, which overrides the sympathetic vasoconstriction response. * The increase in cardiac output has two components: increased heart rate and increased contractility. * Increased contractility results in increased stroke volume, and is represented by increased pulse pressure. * Increased venous return is possible because of sympathetic constriction of the veins (which reduces unstressed volume) and because of the squeezing action of the exercising skeletal muscle on the veins. * Local metabolic responses in the exercising skeletal muscle produce vasodilation. * Overall, TPR decreases because of this vasodilation in skeletal muscle, even though other vascular beds are vasoconstricted. * There is an increase in systolic arterial pressure and pulse pressure because of the increase in stroke volume. * However, diastolic arterial pressure remains the same or may even decrease secondary to the decrease in TPR.