COOLRO~1.PDF
Document Details
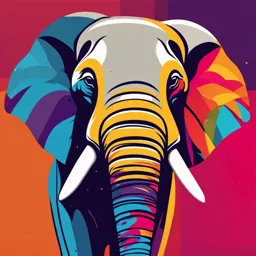
Uploaded by MagnanimousCosmos
2024
Tags
Full Transcript
7/7/24, 1:48 AM Cool Roofs Could Be Most Effective at Reducing Outdoor Urban Temperatures in London (United Kingdom) Compared With Other R… Back Geophysical Research Letters / Volume 51, Issue 13 / e2024GL109634 Research Letter Open Access Cool...
7/7/24, 1:48 AM Cool Roofs Could Be Most Effective at Reducing Outdoor Urban Temperatures in London (United Kingdom) Compared With Other R… Back Geophysical Research Letters / Volume 51, Issue 13 / e2024GL109634 Research Letter Open Access Cool Roofs Could Be Most Effective at Reducing Outdoor Urban Temperatures in London (United Kingdom) Compared With Other Roof Top and Vegetation Interventions: A Mesoscale Urban Climate Modeling Study O. Brousse , C. Simpson, A. Zonato, A. Martilli, J. Taylor, M. Davies, C. Heaviside First published: 04 July 2024 https://doi.org/10.1029/2024GL109634 Abstract Comprehensive studies comparing impacts of building and street levels interventions on air temperature at metropolitan scales are still lacking despite increased urban heat-related mortality and morbidity. We therefore model the impact of 9 interventions on air temperatures at 2 m during 2 hot days from the summer 2018 in the Greater London Authority area using the WRF BEP-BEM climate model. We find that on average cool roofs most effectively reduce temperatures (∼−1.2°C), outperforming green roofs (∼0°C), solar panels (∼−0.5°C) and street level vegetation (∼−0.3°C). Application of air conditioning across London (United Kingdom) increases air temperatures by ∼+0.15°C. A practicable deployment of solar panels could cover its related energetic consumption. Current practicable deployments of green roofs and solar panels are ineffective at large scale reduction of temperatures. We provide a detailed decomposition of the surface energy balance to explain changes in air temperature and guide future decision-making. Key Points City scale deployment of cool roofs leads to the greatest reduction in 2 m air temperature Green roofs do not decrease daily average temperature but have a daytime cooling effect https://agupubs.onlinelibrary.wiley.com/doi/10.1029/2024GL109634#:~:text=We find that on average,∼−0.3°C 1/26 7/7/24, 1:48 AM Cool Roofs Could Be Most Effective at Reducing Outdoor Urban Temperatures in London (United Kingdom) Compared With Other R… Solar photovoltaic panels can reduce temperatures in London by capturing sensible Back heat flux and generate electrical power Plain Language Summary Multiple common city scale passive and active interventions exist to reduce urban population's exposure to extreme heat during hot spells. Nonetheless, a proper comparison of the effect that each of these interventions may have on the temperatures experienced within large cities is missing. Additionally, the radiative and thermal mechanisms that lead to outdoor temperature changes are often not detailed and could lead to detrimental effects for local populations, such as indirect increase of water vapor or reflection of solar radiation. Our study, focusing over London, compares several common interventions through a modeling experiment and finds that cool roofs largely outperform other interventions during the two hottest days of the summer 2018. We also find that green roofs are ineffective on average and that solar panels and tree vegetation would only marginally change temperature exposures. Large scale deployment of air conditioning would lead to increased temperature in the core of London. Solar panels could potentially provide sufficient energy for running air conditioning all over London, creating comfortable indoor environments, and green roofs could reduce temperatures during the day. We argue that such inter-comparisons should guide future decision making. 1 Introduction Cities have become a focus of attention for climate adaptation and mitigation solutions in the face of the increasing challenges induced by global urbanization and climate warming. More specifically, interest in urban temperatures has been growing, as cities are known to alter the local climate to which urban citizens are exposed (e.g., through the Urban Heat Island (UHI) effect (Oke, 1982; Oke et al., 2017) and thereby impact their thermal comfort and climate- related mortality (Arbuthnott et al., 2016; Gasparrini et al., 2015; Masselot et al., 2023). During hot spells, higher mortality and increased likelihood of thermal discomfort are generally observed, hence calling for adaptative and mitigative measures to reduce outdoor air temperatures at the city scale (Heaviside et al., 2016; Iungman et al., 2023; Salmond et al., 2016). To respond to these risks to public health, passive and active strategies have been proposed to cool down the urban indoor and outdoor environments, and to provide indoor sheltering for the most vulnerable. Commonly proposed urban passive cooling strategies, which should be considered as measures that directly lower the temperatures, include increased urban vegetation, roofs incorporating vegetation (known as green roofs), or the deployment of highly https://agupubs.onlinelibrary.wiley.com/doi/10.1029/2024GL109634#:~:text=We find that on average,∼−0.3°C 2/26 7/7/24, 1:48 AM Cool Roofs Could Be Most Effective at Reducing Outdoor Urban Temperatures in London (United Kingdom) Compared With Other R… reflective roofs that can be composed of different roofing materials (e.g., concrete, metal, or Back single-ply membrane), known as cool roofs (Broadbent et al., 2022; Santamouris et al., 2017). Changes to roofs can reduce indoor temperatures or cooling needs in a building (Virk et al., 2014). When deployed at city-scale, they can also reduce the outdoor air temperature and associated heat related mortality (H. Macintyre & Heaviside, 2019). Concerning active strategies, meaning measures that transform the incoming radiation, into energy for example, or that mechanically change the temperatures, air conditioning (AC) is one of the most effective for protection against heat, although it can require large amounts of electrical power and increase outdoor temperatures (Salamanca et al., 2014; Stone Jr et al., 2021). Where passive strategies are not viable or insufficient, AC may be the only option, but it remains the primary solution in some high-income countries despite its problems, like in Phoenix, Arizona (Stone Jr et al., 2021). Lastly, while rooftop solar photovoltaic (PV) panels are primarily considered as a source of electrical power, they can also be considered as a passive-active strategy for impacting outdoor and indoor air temperature by increasing the roofs' albedo and by transforming incoming solar radiation into electrical power that can be used to run the AC system (Ma et al., 2017; Salamanca et al., 2016). Debate on their effectiveness as cooling strategies is currently ongoing (Sailor et al., 2021) and calls for more local studies to understand their costs and benefits at different latitudes. In general, the impact of these strategies has been quantified for a variety of cities across the world (e.g., Chicago (Sharma et al., 2016), Madrid (Salamanca et al., 2014) or Singapore (Yang & Bou-Zeid, 2019)) and physical mechanisms that lead to the cooling/heating of the cities are well understood (Nazarian et al., 2022). Nonetheless, city-specific quantification of these impacts should still be performed to inform the general audience. In addition, few studies have explored in detail how these interventions impact the surface energy balance (SEB) and how these alterations at the city scale can relate to local changes in air temperatures (Broadbent et al., 2020; Krayenhoff et al., 2021). Furthermore, impact studies of urban scale interventions are often challenged by the lack of geographical data on realistic extent at which each intervention could be applied. This means that it is often impossible to give a proper estimate of the potential impact that interventions can have at a city-scale. Large-scale controlled trials of urban interventions are, so far, rare, and much of the evidence for their effectiveness comes from modeling. Urban climate models (UCMs) embedded in regional climate models (RCMs), after proper model evaluation, can provide an accurate estimation of the impact of urban areas on local climates, as well as the impact of interventions (Krayenhoff et al., 2021). Indeed, most UCMs are coupled to a variety of intervention strategies (e.g., green roofs and solar panels (Zonato et al., 2021) or AC systems (Salamanca et al., 2010; Salamanca & Martilli, 2010)). https://agupubs.onlinelibrary.wiley.com/doi/10.1029/2024GL109634#:~:text=We find that on average,∼−0.3°C 3/26 7/7/24, 1:48 AM Cool Roofs Could Be Most Effective at Reducing Outdoor Urban Temperatures in London (United Kingdom) Compared With Other R… In this study, we use detailed urban climate modeling, as well as recent data on the actual Back coverage of passive(-active) interventions, to estimate the impact on outdoor temperature of several interventions during hot summer days on the average temperature within the Greater London Authority (GLA) boundaries. By considering a set of scenarios that include both active and passive heat adaptation strategies, our study makes a comprehensive analysis of the impact of each strategy on the outdoor air temperature and the SEB of the Greater London area. Because we expect some of these strategies to not be applicable to all buildings, like green roofs or solar PV panels, we provide practicable scenarios to compare the hypothetical maximum with more feasible implementation. We also discuss the potential energy costs and benefits of air conditioning and PV, and the advantages and disadvantages of vegetation-based strategies. 2 Methods We run a set of 11 urban climate simulations to physically represent the potential impact of urban temperature reduction interventions at a high spatio-temporal resolution of 1 km with hourly outputs on the two hottest days of summer 2018, the 26th and the 27th of July— considering the 25th as spin-up time. 2018 is chosen as it was the hottest summer on average for the United Kingdom (McCarthy et al., 2019); see Appendix B in Supporting Information S1 for more information on the background weather conditions. We evaluated our control scenario—consisting of the same model set up as in Brousse et al. (2023) without any heat intervention strategy—over two hot summer days against a set of in situ observations of air temperature, relative humidity and wind speed and found its performance acceptable for our study (more information on the evaluation and its outcomes can be found in Appendix C in Supporting Information S1). 2.1 Model Configuration We use the Weather Research Forecast (WRF) v4.3 RCM following the previous model domain and set up of Brousse et al. (2023). The impact of cities on the local climate is simulated by activating the Building Energy Parameterization (BEP; Martilli et al. (2002)) model with its coupled Building Energy Model (BEM; Salamanca et al. (2010); Salamanca and Martilli (2010)), hereafter referred as BEP-BEM. We use the same model dynamics and physics set up as Brousse et al. (2023). For the urban canopy parameters and the land cover classification we use the default MODIS land cover from WRF and categorize the urban pixels to Local Climate Zones (LCZ; Stewart and Oke (2012)) following the WUDAPT-TO-WRF strategy to provide the WRF model with spatially explicit urban morphological parameters (Brousse et al., 2016; Demuzere et al., 2022). The building characteristics are provided per LCZ (see Figure D1A in Supporting Information S1) and are the same as in Brousse et al. (2023). More information on the model configuration can be found in Appendix A in Supporting Information S1. https://agupubs.onlinelibrary.wiley.com/doi/10.1029/2024GL109634#:~:text=We find that on average,∼−0.3°C 4/26 7/7/24, 1:48 AM Cool Roofs Could Be Most Effective at Reducing Outdoor Urban Temperatures in London (United Kingdom) Compared With Other R… Although our model is run over the whole of south-east England to accurately represent the Back interaction between local interventions and mesoscale atmospheric circulations, we focus our study on the GLA metropolitan area only because our practicable interventions are valid for that specific area. Our study aims at providing a general understanding of how different interventions can impact the average temperature of the GLA area during hot summer days. It does not investigate reasons explaining local impacts despite presenting some observable local features. 2.2 Temperature Interventions We define a set of 10 passive or active interventions that are theoretically applicable over the Greater London metropolitan area. Our scenarios are separated into two groups: (a) where interventions are deployed over the whole city to shed light on the maximum impact achievable by each intervention, and (b) practicable interventions that inform us about the actual potential for heat reduction in London—given the existing built environment. Most of our interventions consist of roof interventions and each intervention is compared to our control run to estimate the impact of each intervention on that urban climate. We also simulate the effect of widespread use of active cooling in the form of air conditioning. Our scenarios consist of: (a) the deployment of cool roofs by increasing the albedo of each urban class to 0.85 (CR100); (b) the deployment of green roofs composed of sedum vegetation in all urban classes by activating the green roof model from Zonato et al. (2021) with its default irrigation hours from 23.00 UTC to 01.00 UTC (GR100); (c) the deployment of solar PV panels on all urban roofs by activating the solar PV model from (Zonato et al., 2021) in its default version (PV100); (d) the installation of air conditioning systems in all buildings and at all floors by activating the air conditioning model embedded in the BEM model in its default version—with a coefficient of performance of 3.5 (see Salamanca et al. (2010); Salamanca and Martilli (2010); AC100); (e) the change of the urban vegetation from cropland and grass land to urban deciduous trees as implemented in Noah-MP (Niu et al. (2011), VG100); (f) the potential for solar PV deployment over roofs of existing buildings in the Greater London taking into account roof slope and overshadowing derived from the London solar opportunity map (PVpot; Steadman et al. (2020); Figure D1C in Supporting Information S1); (g) the potential for retrofit of green roofs on existing buildings in Greater London obtained through a detailed geographical information system analysis (C. Simpson et al. (2022); GRpot; Figure D1B in Supporting Information S1); (h) the installation of cool roofs in compact and open mid-rise areas (Local Climate Zones 2 and (e) from the center of London (CRmid); (i) in open low rise areas (Local Climate Zone 6; CRlow) from the residential suburbs of London; and (j) in large lowrises of London (Local Climate Zone 8; CRllr). More information about each model can be found in Appendix A in Supporting Information S1. https://agupubs.onlinelibrary.wiley.com/doi/10.1029/2024GL109634#:~:text=We find that on average,∼−0.3°C 5/26 7/7/24, 1:48 AM Cool Roofs Could Be Most Effective at Reducing Outdoor Urban Temperatures in London (United Kingdom) Compared With Other R… Potential conjoint use of green and cool roofs with solar panels have been proposed to Back increase solar panel efficiency while reducing urban surface temperatures in particular (Fleck et al., 2022; Schindler et al., 2018), but it was not possible to analyze this in our model. Sedum was modeled for the green roofs due to its increasing presence on new buildings in London (C. Simpson et al., 2022), but other plants such as turf may have a different impact. To integrate the spatially-explicit variation in solar PV panels and green roofs coverage from the scenarios PVpot and GRpot, we adapted the code from Zonato et al. (2021) and added a field to the WRF geographical input files. These two scenarios also explain why our study focuses only on GLA area as these are bounded to its administrative boundaries and are not applicable to the whole south-east of England which composes our WRF domain. Lastly, we expect the impacts to be more prominent in London due to its higher UHI intensity (Bassett et al., 2021; Brousse et al., 2022). 3 Results 3.1 Impact of Interventions on the Temperature In general, we find that cool roofs are the more effective interventions to reduce the heat in the GLA boundaries with a 2-day average cooling of ∼1.2°C going up to ∼2.0°C in certain locations (Figures 1 and 2). All cool roofs interventions contribute to a reduction of T2 on average (Figure 2) with major impacts found in the south and the east of GLA if deployed at 100% (CR100; Figure 1). Horizontal advection of cooling depends on the location where cool roofs are implemented. For instance, if cool roofs are implemented in more central and mid-rise areas of London, more open and low-rise residential areas appear to benefit from this cooling by ∼0.15°C on average. Interestingly, implementing cool roofs on residential low-rise buildings only (CRlow) does not cool the central parts of the cities despite providing a substantial outdoor T2 reduction by up to ∼2°C in the south-east. Establishing cool roofs only on large lowrises (CRllr) only results in a marginal heat reduction on average over London but does lead to a heat reduction of up to ∼1°C in large lowrises areas of east-London. Compared to cool roofs, we find more minor decreases in T2 of ∼0.3°C on average related to the change in natural cover from a mixture of croplands and grasslands to deciduous trees (VG100). The installation of solar panels over the whole city (PV100) leads to a reduction of T2 by ∼0.5°C. Stronger cooling effects are found in the east of London for these two scenarios as well as for CR100 and CRllr. Lastly, despite a high spatial heterogeneity of the cooling provided by the remaining interventions (green roofs (GR100 and GRpot); practicable retrofit potential of solar panels (PVpot)), none is found to be beneficial for T2 reduction on average for the whole metropolitan area (Figures 1 and 2). Using air conditioning for maintaining the indoor temperature of all London's building stock to 21°C (AC100) induces a heating of central London by up to ∼1°C and of ∼0.15°C on average for the whole city. https://agupubs.onlinelibrary.wiley.com/doi/10.1029/2024GL109634#:~:text=We find that on average,∼−0.3°C 6/26 7/7/24, 1:48 AM Cool Roofs Could Be Most Effective at Reducing Outdoor Urban Temperatures in London (United Kingdom) Compared With Other R… Back Figure 1 Open in figure viewer PowerPoint Average 2 m air temperature of the control run (T2; top left panel) and the average difference of each intervention (ΔT2). Gray contours are WRF urban pixels (classified as an urban LCZ). https://agupubs.onlinelibrary.wiley.com/doi/10.1029/2024GL109634#:~:text=We find that on average,∼−0.3°C 7/26 7/7/24, 1:48 AM Cool Roofs Could Be Most Effective at Reducing Outdoor Urban Temperatures in London (United Kingdom) Compared With Other R… Back Figure 2 Open in figure viewer PowerPoint Time-averaged impact of each intervention on each surface energy balance component and the respective change in air temperature at 2 m for all urban pixels (small dots). Average impact is represented by the large dots. In addition we find that, independent of the weather conditions, cooling brought by interventions tends to be greater in places that are hotter in the control run; except for CRllr which shows greater cooling in places that are already cooler on average, and AC100 that would increase the heat in hotter parts of London (Figure D2 in Supporting Information S1). The latter could be explained by a higher release of sensible heat in the hottest parts of London under the AC100 scenario and an increase of albedo in large lowrises of CRllr which are already cooler than other parts of London due to their location next to the rivers and canals and their higher roof reflective capacity. These results highlight the complex interactions between local cooling capacity and the total cooling load that can be brought to a city by each intervention. 3.2 Temperature and Surface Energy Balance Changes By relating the change in T2 of all urban pixels in the GLA area to their relative SEB components (Figure 2) we find that the large decrease in T2 induced by the full scale deployment of cool https://agupubs.onlinelibrary.wiley.com/doi/10.1029/2024GL109634#:~:text=We find that on average,∼−0.3°C 8/26 7/7/24, 1:48 AM Cool Roofs Could Be Most Effective at Reducing Outdoor Urban Temperatures in London (United Kingdom) Compared With Other R… roofs (CR100) is linked to an increase in albedo by ∼0.3 and a reduction of incoming net solar Back shortwave radiation by ∼50 W m−2. This is accompanied by a reduction of sensible heat fluxes of ∼30 W m−2, on average. Notably, we also observe an average increase of net longwave radiation budget by ∼10 W m−2 when deploying cool roofs over the whole city (CR100) and in open residential areas (CRlow), which could be caused by less negative net longwave radiation during the day due to reduced upward emission from the lower temperature surface. Higher emissions of sensible heat fluxes by ∼5 W m−2 are found in both the air conditioning (AC100) and the deciduous tree (VG100) scenarios. While the former is expected as AC systems remove the accumulated indoor heat outside mostly in the form of sensible heat, the latter is counter intuitive as more vegetated areas are expected to mostly transform energy in the form of latent heat fluxes via evapotranspiration—something that is modeled with an average increase of latent heat fluxes by ∼12 W m−2. We however observe a decrease in surface albedo by 0.05 and an increase of incoming net solar shortwave radiation of ∼5 W m−2, on average, which could explain this phenomenon. Despite an increase of T2 induced by green roofs of less than 0.1°C on average, hourly spatial averages of changes in T2 show that if green roofs were implemented across the whole city (GR100), temperatures could be decreased by ∼0.5 to ∼0.8°C during hot hours of the day (11.00 UTC to 14.00 UTC; Figure 3 and Figures D3 and D4 in Supporting Information S1). Nonetheless, temperatures would also be increased at times of daily minimum T2 by ∼0.5°C (03.00 UTC to 06.00 UTC, Figure 3). This is consistent with findings by Zonato et al. (2021) and explained by an increased release of latent fluxes during the day but a higher storage of heat fluxes at night due to green roofs' thermal mass. The current potential for green roofs in London (GRpot) would not reduce the temperature by more than 0.1°C on average across the period. During the hotter hours of the day, the impact of cool roofs (CR100) can be 2.5 times, and the one from cool roofs over open-low rises (CRlow) 2.0 times, greater than the one of green roofs. Both these scenarios (CR100 and CRlow) also contribute to a constant reduction of temperature of at least 0.5°C throughout the day. This is correlated to a high reduction of emitted sensible heat fluxes and of the positive intakes of ground fluxes throughout daytime hours (Figures D3 and D4 in Supporting Information S1). Notably, the temperature reduction across the whole city induced by cool roofs over central mid-rises from London (CRmid) reaches a peak at later hours, around 15.00 UTC, a few hours after the peak in reduction of sensible heat fluxes reached at noon. Higher temperature reduction by ∼0.8°C is observed in the evening if vegetation is changed to deciduous trees (VG100). During the day, no tangible impact is found in this case, as half of the urban pixels observe an increase in temperature while the other half observes a decrease. This may be because higher levels of sensible heat fluxes are emitted; more latent heat fluxes are also emitted throughout the day. Both full scale and practicable solar panel implementation (PV100 and PVpot) behave similarly to cool roofs by decreasing the emitted sensible heat fluxes and reducing the intake of fluxes to the ground during daytime hours. The temperature https://agupubs.onlinelibrary.wiley.com/doi/10.1029/2024GL109634#:~:text=We find that on average,∼−0.3°C 9/26 7/7/24, 1:48 AM Cool Roofs Could Be Most Effective at Reducing Outdoor Urban Temperatures in London (United Kingdom) Compared With Other R… reduction of up to ∼1.0°C, for the full-scale deployment, however, only happens during the late Back afternoon and the evening. At time of daily maximum temperatures, PV100 reduces the temperature in similar ways to GR100 (Figure 3 and Figures D3 and D4 in Supporting Information S1). Figure 3 Open in figure viewer PowerPoint Cumulative distributions of change in air temperature at 2 m for different intervention scenarios. Distributions are of change relative to the reference scenario in average daily mean, daily minimum, and daily maximum 2 m air temperature across grid cells. Distributions are from 200 bin histograms, normalized to unity and based on modeled air temperature on 26th and the 27th of July 2018 in London. The horizontal dashed line indicates the median change. The vertical dashed line indicates zero change. 4 Discussion Using the WRF BEP-BEM mesoscale urban climate over the Greater London metropolitan area during the two hottest days of 2018, we performed one of the first comprehensive evaluations of the impact of building and street level interventions tailored at reducing outdoor and indoor heat on the outdoor temperature and the SEB in London. We find that interventions such as cool roofs, green roofs, rooftop solar PV, and changing natural spaces to deciduous woodland can contribute to a cooling of outdoor temperatures at hyper-local or city scales (also see Figures D5–D10 in Supporting Information S1, while https://agupubs.onlinelibrary.wiley.com/doi/10.1029/2024GL109634#:~:text=We find that on average,∼−0.3°C 10/26 7/7/24, 1:48 AM Cool Roofs Could Be Most Effective at Reducing Outdoor Urban Temperatures in London (United Kingdom) Compared With Other R… widespread use of air conditioning could lead to substantial increases in outdoor temperature Back in the center of London. We find that this cooling is on average stronger in East London, suggesting that complex dynamics in atmospheric circulation and heat advection could be at play (see Brousse et al. (2022)) and would require further studies to disentangle when certain boroughs of London observe greater cooling reductions. This outcome could also simply be related to the short simulation period over which we average the results and be related to specific daily conditions (see Figure D11–D24 in Supporting Information S1). On average, cool roofs would be the most effective way of reducing outdoor temperature at the city scale, in line with other modeling studies (e.g., Sharma et al., 2016; Tan et al., 2023; Yang & Bou-Zeid, 2019). At the maximum, this temperature reduction is between 3.2°C and 2.8°C at times when daily spatial average temperatures reached 33°C and 37°C, respectively (Figure D4 in Supporting Information S1), similar to the cooling found in Phoenix and New York (Sinsel et al., 2021). In comparison, if green roofs or rooftop solar PV panels were deployed over all buildings in the Greater London area, the temperature reduction would have never reached more than ∼1.0°C for both days; considering that overnight, green roofs would increase the average temperature due to their increased heat-storage capacity that increases heat release at night (Tan et al., 2023). Of note, our green roofs are modeled with sedum vegetation as they are the most common in London; other designs may lead to other effects as Zonato et al. (2021) found an increased reduction using grass-based green roofs. Conversely, we find that changing the modeled vegetation type of urban natural spaces from grasslands/croplands to deciduous trees would reduce outdoor temperatures only during the night and found mixed effects during the day, possibly due to an increase in the surface albedo which leads to an increased sensible heat emission during the day. Latent heat would also increase as expected from more evapotranspiration and would be equivalent to the increase in latent heat from green roofs deployed at full scale. Although cooling via evapotranspiration reduces air temperature, it increases water vapor in the atmosphere; this could be beneficial for avoiding water stress of vegetation but affect the heat stress from city's inhabitants and should be evaluated using a set of multiple heat stress metrics (C. H. Simpson et al., 2023). City scale impacts of any intervention are reduced when applied to a smaller area or fewer buildings. For cool roofs, we find that although major impacts are found when applied over low-rises residential areas of London, already applying them over central mid-rises of the city could reduce the temperature in surrounding residential areas. Because of the relatively small area covered by large lowrises in London, applying cool roofs over them would only marginally contribute to a heat reduction of the whole city, contradictory to results in Birmingham where commercial and industrial areas cover an important part of the city center (H. Macintyre & Heaviside, 2019). They could also be considered less effective as large lowrise buildings already observe higher albedo than more residential areas in our model (Brousse et al., 2023). Cool roofs may act to reduce indoor temperatures in summer, but also potentially in winter, thus https://agupubs.onlinelibrary.wiley.com/doi/10.1029/2024GL109634#:~:text=We find that on average,∼−0.3°C 11/26 7/7/24, 1:48 AM Cool Roofs Could Be Most Effective at Reducing Outdoor Urban Temperatures in London (United Kingdom) Compared With Other R… increasing winter heating demand. Therefore, it is important that they are installed in Back conjunction with insulation in homes (Taylor et al., 2018). However, in winter, cool roofs have less of a cooling effect on outdoor temperatures than they do in summer (H. L. Macintyre et al., 2021). While we find that full application of rooftop solar PV leads to some cooling on average, practicable application, constrained by existing building characteristics, is not enough to have an appreciable effect on the temperature of the GLA. Similarly, full application of sedum green roofs can reduce daytime temperatures, but the potential for retrofit of existing buildings with sedum green roofs is not enough to produce a substantial cooling effect at the city scale. Although air conditioning would increase the outdoor temperature, it could still protect vulnerable populations from overheating indoors. Through our modeling experiment, we find that if solar panels were implemented in a practicable manner on the rooftops of existing buildings, the energy production, peaking at 15.15 MW at time of higher solar incidence (11.00 UTC) and with an average production of 4.70 MW, would cover the energetic consumption induced by AC systems which never goes above 8.78 MW during the hottest hours (15.00 UTC to 17.00 UTC), averaging to 4.61 MW throughout the day. At night, energy production being null by solar panels, the necessary energy for AC systems would have ideally been stored in batteries. Under the assumption that deployment of solar panels is not constrained by existing buildings, electric power production could go to 8.79 MW on average, and outdoor average temperature could be reduced by ∼0.3°C, something negligible in the practicable scenario. This temperature reduction should be carefully interpreted as several studies have found that solar panels could heat up the surrounding environment (Sailor et al., 2021). Nonetheless, these local impacts may be dependent on many factors, including the building height and the building plan area fraction (Zonato et al., 2021), or the increase in rooftop albedo. In our case, we argue that the capture of solar radiation by the solar PVs is sufficiently important to lower the expected intake of radiation by the building and that most of it is transformed in the form of electric energy rather than in sensible heat over the roof. In the evening and at night, the low thermal capacity of solar PVs explains the higher cooling capacity of the urban environment. In any case, our results call for more investigation on this specific topic as other studies, like (Tan et al., 2023), found a small night-time increase in temperature over Chicago when implementing solar PV over buildings' roofs. Hence mechanisms explaining solar PV efficiency in our model need to be further explored. 5 Limitations and Future Work Several limitations apply to this study. First, we used only one UCM, and another UCM may produce different results depending on its dynamics and physics (Krayenhoff et al., 2021). Second, we focused our study only over two hot summer days due to computational https://agupubs.onlinelibrary.wiley.com/doi/10.1029/2024GL109634#:~:text=We find that on average,∼−0.3°C 12/26 7/7/24, 1:48 AM Cool Roofs Could Be Most Effective at Reducing Outdoor Urban Temperatures in London (United Kingdom) Compared With Other R… limitations. In general, performing such comprehensive analysis with multiple scenarios comes Back at high computational costs, which often prevents the generalization of the results to expected average seasonal impacts of these interventions or to other cities at similar latitudes. Third, despite the fact that the 26th and the 27th of July were extremely hot days during the summer 2018 in the United Kingdom, full clear-sky conditions are absent from our model simulations. Some of the results observed in this study concerning the SEB may therefore simply be related to the overpass of clouds—although we did not find a particular difference in cloud coverage between our control simulation and our scenarios—and would be difficult to demonstrate as urban scale SEB products do not exist at hourly time step. Fourth, as discussed above, our study only focuses on outdoor temperatures and does not consider the benefits or the detriments caused by these interventions on other aspects (e.g., biodiversity, indoor temperature, increase in relative humidity, wind circulation, etc.). Fifth, the estimated impacts presented in this study assume a single type of application per intervention (e.g., green roofs are only composed of sedum vegetation, solar panels are based on a single type of photovoltaic materials, cool roofs are simply concrete with high albedo, for example) and do not explore the benefits provided by more recent technological advancement in each of these categories. For instance, our study did not look at the local climate effect of heat pumps turned on cooling mode as this feature is not yet available in BEP-BEM. Sixth, although evident average spatial features are observable for some interventions (e.g., increased temperature in the center of London under AC100) others may not have been captured due to random local effects dependent on weather conditions during the short period of study. Seventh, our VG100 scenario does not account for the radiative and thermal effects of trees within the urban canyon (like in BEP-Tree (Krayenhoff et al., 2020), for example,) but simply changes the bulk physical properties of the natural tile handled by the Noah-MP model. In the model, the natural tile in each model grid cell is subsequently weighted averaged with the urban tile, depending on the urban fraction. Lastly, all our estimated impacts should be interpreted cautiously as the average temperature differences resulting from the interventions are smaller than the model MAE with respect to observations (Krayenhoff et al., 2021) and are dependent on 2 days with different cloud coverage and weather conditions. Despite these limitations, we expect our results to be at the least indicative of which interventions are capable of producing the greatest cooling effect in London during hot summer days. To address the limitations of our study and push the research on the benefits of mitigative and adaptive strategies, future research should: (a) estimate the impact of each of these strategies on longer time periods (e.g., monthly or seasonal); (b) perform multi-model simulations over the same area to better quantify the uncertainty around the impact of each intervention; (c) investigate the complex interactions between local and city-scale impacts of tailored interventions; (d) estimate the costs related to the deployment of each of these interventions to perform cost-benefit analyses; (e) foster the deployment of urban scale SEB monitoring https://agupubs.onlinelibrary.wiley.com/doi/10.1029/2024GL109634#:~:text=We find that on average,∼−0.3°C 13/26 7/7/24, 1:48 AM Cool Roofs Could Be Most Effective at Reducing Outdoor Urban Temperatures in London (United Kingdom) Compared With Other R… systems to validate model simulations—similar to crowd-sourcing of air temperature; (f) Back encourage the installation of monitoring systems of weather variables and SEB over more recent or recently retrofitted buildings which often integrate new roof designs, for example. 6 Conclusions Adaptation to urban heat is of increasing priority. Our modeling study suggests that widespread adoption of cool roofs could be efficient at reducing urban temperatures, and therefore adverse health impacts, during hot spells. Rooftop solar PV may also provide a small amount of cooling as an additional benefit to power production; green roofs appear less effective in reducing temperatures but have other environmental benefits. Furthermore, relying on AC will lead to increased outdoor temperatures, despite improved thermal comfort indoors. It is reasonable to think that there will be a mixture of adaptation measures implemented across the city of London, and their applicability and impact should be further investigated. Our research is a first step toward this and will be of interest to city planners and decision-makers within the built environment sector. Acknowledgments CH is supported by a NERC fellowship (NE/R01440X/1) and acknowledges funding for the HEROIC project (216035/Z/19/Z) from the Wellcome Trust, which funds OB and CS. OB designed the study and led the conception of the manuscript with the support of CH and CS. OB was responsible for the WRF modeling, the model evaluation and the analysis. CS provided support in the integration of green roofs and solar PV inputs into WRF. AZ implemented a spatially- explicit input of solar PV and green roofs coverages in WRF. AZ and AM offered guidance in the set-up of the WRF model v4.4 and in the analysis of the outputs. JT and MD provided support in the design of the study and in the acquisition of knowledge about building interventions. All authors contributed to the writing of the manuscript. The authors thank Copernicus for the ERA5 reanalysis data, the UK MetOffice for the MIDAS Open data and the Netatmo company for the personal weather stations data. Open Research Data Availability Statement The simulations done in this research were performed using the WRF model v4.4 ( https://github.com/wrf-model/WRF.git ). The related outputs presented in this research and codes used to plot them are available at Brousse et al. (2024 ). The data used in the Supporting Information https://agupubs.onlinelibrary.wiley.com/doi/10.1029/2024GL109634#:~:text=We find that on average,∼−0.3°C 14/26