Excitation-Contraction Coupling PDF
Document Details
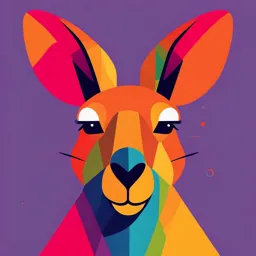
Uploaded by ProficientRapture7037
Robert Gordon University
Stuart Cruickshank
Tags
Summary
This document provides a detailed explanation of excitation-contraction coupling, focusing on the processes involved in muscle contraction, particularly the interaction of actin and myosin. It explores various aspects, including microfilaments, myosin, and the detailed actin-myosin cycle, offering insights into the molecular mechanisms involved.
Full Transcript
Excitation-Contraction Coupling Stuart Cruickshank Actin microfilaments- what and where? Microfilaments: – filaments composed of actin protein, usually found in cross- linked bundles and networks – responsible for many cell moveme...
Excitation-Contraction Coupling Stuart Cruickshank Actin microfilaments- what and where? Microfilaments: – filaments composed of actin protein, usually found in cross- linked bundles and networks – responsible for many cell movements – form a variety of structures within cells: Contractile bundles: Lamellipodia and filopodia: Contractile ring: Microvilli: intestinal cytoplasm leading edge of migrating between dividing cells cells cells lamellipodium filopodium Actin microfilaments- structure Actin filaments: two-stranded helix with a actin molecule repeated twist every 37 nm - end strong interactions between the strands prevent separation like microtubules, actin filaments have a twist structural polarity Filaments can grow by addition of actin monomers at either end but they grow more quickly at the + end + end Actin microfilament assembly microfilaments assemble from a cytoplasmic pool of actin subunits with bound ATP ATP bound to each free actin monomer is hydrolyzed to ADP upon binding (similar to GTP hydrolysis on tubulin), which reduces binding strength and decreases stability of the filament Therefore actin filaments need associated proteins for stability Without them they can dissociate from both ends The rate of assembly is controlled by actin binding proteins At a given time about half of the actin in the cytoplasm is in filaments- rest is free monomer so what controls extent of polymerisation? thymosin and profilin: proteins that bind to actin monomers, preventing their addition to filaments- thus keeps monomer pool in reserve other actin binding proteins bind to filaments to regulate their function e.g. tropomyosin Actin motor proteins -myosins All actin motor proteins belong to the myosin family ATP-binding head ATP provides the energy for myosin movement along microfilaments muscle myosin always move from the - end toward the + end several types: myosin-I and myosin-II are most abundant aggregate of myosin -II molecules with heads pointing in opposite directions Roles of myosin-I and myosin-II myosin-I: moves vesicles along microfilaments by repeated ATP hydrolysis at the globular head region myosin-II: small myosin-II filaments can slide actin filaments across each other, thus shortening an actin bundle heads point in opposite directions myosin-I: moves an actin filament relative to the plasma membrane via ATP hydrolysis But why is this important? Muscle contraction…. Three types of muscle. 1) Skeletal muscle. 2) Cardiac muscle. 3) Smooth muscle. All rely on an interaction by actin and myosin filaments. The actin-myosin cycle: in brief attached: myosin head without ATP attached to an actin subunit released: ATP binds to myosin- releases actin cocked: ATP binding causes myosin head to change shape force-generating: release of Pi from ATP hydrolysis triggers myosin shape change. Myosin loses its ADP, cycle starts again. attached: Myosin reattaches to nearest actin subunit, but it will be about 15 nm away from previously bound subunit The actin-myosin cycle attached: myosin head (without ATP) locked to an actin subunit the rigor configuration- responsible for rigor mortis The actin-myosin cycle released: ATP binds to myosin head changes shape of the actin-binding site on the head releases actin The actin-myosin cycle cocked: A cleft on the mysoin head closes (like a clam shell) around the ATP triggers a shape change causes the head to move ATP hydrolysis occurs but the ADP and Pi remain tightly bound The actin-myosin cycle force-generating: myosin head binds to new site on actin- release of Pi from ATP hydrolysis and tight binding to the actin The release triggers the force-generating shape change (power stroke) the head regains its original shape During the power stroke the head loses its bound ADP thereby returning to the start of the cycle The actin-myosin cycle next binding site previous binding new binding site site attached: The myosin head is again locked tightly to the actin filament Note that the head has moved to a new position on the filament So what regulates this myosin/actin interaction? Three types of muscle. 1) Smooth muscle 2) Cardiac muscle 3) Skeletal muscle. All rely on an interaction by actin and myosin fillaments. Fuelled by ATP and initiated by increasing [Ca2+]i. The effects of drugs on muscle contraction is the basis of many therapeutic applications. Troponin and tropomysin control muscle contraction Ca2+ interacts with a molecular switch made of the accessory proteins tropomysin and troponin tropomysin is a rigid rod-shaped molecule that binds in the groove of the actin helix, preventing the myosin heads from interacting with the actin filament troponin is a protein complex that includes a Ca2+ sensitive protein (troponin-C), which is associated with the end of a tropomysin molecule Controlling muscle contraction when Ca2+ levels increase Ca2+ binds to troponin causing the tropomysin molecules to shift position, permitting the actin-myosin interaction required for contraction but what STOPS contraction? When nerve signal stops so does the increase in Ca2+ because specialized pumps move Ca2+ back into the sarcoplasmic reticulum as soon as resting Ca2+ levels restored, the troponin and tropomysin move back to original positions- prevent myosin binding- ends contraction Skeletal Muscle 1) Possesses array of transverse T-Tubules 2) T-tubule possesses proteins (dihydropyridine receptors (DHPR)) 3) DHPR NOT a channel but connected to the SR. 4) Depolarization activates ryanodine receptors in the SR 5) Releases Ca2+ into sarcoplasm. 6) Ca2+ bind to protein TROPONIN 7) Troponin hold tropomyosin in place. 8) Tropomyosin normally blocks actin/myosin interaction 9) Ca2+/troponin complex displaced and actin and myosin interact. 10) Muscle responds with rapid twitch. Cardiac Muscle 1) Possesses large transverse T-Tubules 2) T-tubule L-Type Ca2+ channels 3) L-Type is a channel but is NOT connected to the SR. 4) Depolarization activates Ca2+ influx. 5) Ryanodine receptors in the SR release Ca2+ in Ca2+-induced Ca2r release. 6) Releases Ca2+ into sarcoplasm. 7) Ca2+ bind to protein TROPONIN 8) Troponin/tropomyosin normally blocks actin/myosin interaction 9) Ca2+/troponin complex displaced and actin and myosin interact. 10) Muscle responds with rapid twitch. Smooth muscle 1) Ca2+ increases in cytosol. (entry either by GPCR, or LGCC or VGCC) 2) Ca2+ binds to protein CALMODULIN. 3) Ca2+-calmodulin complex activates Myosin Light Chain Kinase (MLCK) 4) MLCK phosphorylates MLC. 5) Allows actin/myosin interaction (contraction) 6) Myosin phosphatase (MP) reverses the phosphorylation and causes relaxation. 7) cAMP and cGMP regulate MLCK and MP activity. Elevated [Ca2+]i initiated by either a)Receptor mediated pathway (including G-protein signalling pathways). b)Receptor independent (e.g. ion channel activation).