MECH2636: Design and Manufacture 2 - Solid Deformation Processes PDF
Document Details
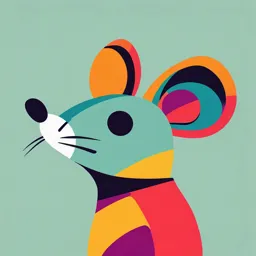
Uploaded by GratefulObsidian5703
University of Leeds
Tags
Summary
This document is a presentation on Solid Deformation Processes in mechanical engineering. It covers topics such as rolling, extrusion, drawing, and forging. The presentation, from the University of Leeds, includes examples and diagrams.
Full Transcript
MECH2636: Design and School of Mechanical Manufacture 2 Engineering FACULTY OF ENGINEERING MECH2636: Design and Manufacture 2 Solid Deformation Processes 2. Rolling MECH2636: Design and Manufacture 2 R...
MECH2636: Design and School of Mechanical Manufacture 2 Engineering FACULTY OF ENGINEERING MECH2636: Design and Manufacture 2 Solid Deformation Processes 2. Rolling MECH2636: Design and Manufacture 2 Rolling (1) Steel is still the most common material used in engineering structures and components The most common ways of producing steel is either casting an ingot or continuous casting Rolling is normally the next step in steel processing. The material is forced through pairs of rolls to generates plates, bars, rods, pipes, and various other shapes Normally rolling will take place on a number of rolls sequentially to get the shape required without excessive force/deformation at each stage MECH2636: Design and Manufacture 2 Rolling (2) ω Most rolling processes are carried out with metal “hot” or “warm”, with finish rolling v2 normally carried out “cold” v1 h1 In a rolling operation, the h2 speed of the slab at exit must be greater than the speed at entrance to roll ω The output speed can be estimated assuming constant volume (& constant width) of workpiece: v1.h1 = v2.h2 Hence v2 = v1.h1/h2 MECH2636: Design and Manufacture 2 Rolling (3) F Contact between roll and R workpiece falls into two zones: A-B where the roll is travelling faster than the workpiece and B-C where A B C the workpiece travels faster than the roll In both zones, there is slip at the interface between the roll and the workpiece F Inbetween these 2 zones is the no-slip point where contact pressure is max. The roll separating force F acts through centre (B) of contact zone which is of length L MECH2636: Design and Manufacture 2 Rolling (4) The length of contact is approximated by: L R (h 1 − h 2) where R is the roll radius and h1, h2 are the workpiece thicknesses before and after rolling The force generated on the rolls increases with the flow stress of the material y , the length of contact L, the width and average thickness of the work w & hav and friction : L F y ( Lw ) 1 + 2 hav MECH2636: Design and Manufacture 2 Rolling (5) If the force generated is high enough to distort the rolls, the workpiece will also be distorted Also a high force implies a high torque & power required to rotate the rolls and deform the workpiece Assuming the force is applied normal to the plane of the workpiece at a moment arm of L/2 to the axis of the rolls, the torque required per roll is given by: T F L / 2 Power required = Torque x Rotational speed (in radians) MECH2636: Design and Manufacture 2 Worked example: power required in rolling (Ref: S & K – example 13.1) A 225 mm wide 6061 aluminium alloy strip is rolled from a thickness of 25 mm to 20 mm between 2 rolls each of radius 300 mm. Estimate the roll separating force and the total power required for this operation if the roll speed is 100 rpm. Assume the material flow stress is 147 MPa and the friction coefficient between workpiece and roll is 0.2. MECH2636: Design and Manufacture 2 First, calculate contact length: Then calculate force: MECH2636: Design and Manufacture 2 Next, calculate torque & power required: Power per roll Total power required = 608 kW (because there are 2 rolls!) MECH2636: Design and Manufacture 2 Rolling Mills (1) Rolling mills can take a number of forms, depending on the number of rolls and their arrangement, most common are: – Two high - simplest – Three high - more productivity per roll – Four high - small inner roll to do the work reduces force and power requirements, backing roll reduces distortion – Sendzimir - lots of rolls, very rigid and accurate, normally cold rolling MECH2636: Design and Manufacture 2 Rolling Mills (2) MECH2636: Design and Manufacture 2 Rolling mills (3) Lubrication is normally used to minimise friction between rolls and workpiece Shape rolling is used to produce a wide range of structural shapes through multiple passes MECH2636: Design and Manufacture 2 Copyright Notice This presentation is protected by UK and international copyright law. Reproduction and distribution of this presentation without written permission from the originator is prohibited. Copyright © 2024 University of Leeds UK. All rights reserved. MECH2636: Design and Manufacture 2 School ofand Extrusion Mechanical Drawing Engineering FACULTY OF ENGINEERING MECH2636: Design and Manufacture 2 Solid Deformation Processes 3. Extrusion & Drawing MECH2636: Design and Manufacture 2 Extrusion and Drawing Extrusion & Drawing Extrusion and drawing are two related processes used to produce relatively long lengths of material with a certain cross-sectional shape Extrusion involves “pushing” a billet of material through a shaped or tapered die whereas drawing involves “pulling” the material through the die In both cases, the cross-sectional area of the billet is reduced and the extruded or drawn material emerges with the cross-sectional shape of the die. MECH2636: Design and Manufacture 2 Extrusion and Drawing Metal Extrusion (1) In its simplest form (known as forward extrusion), pressure is applied to a metal billet to force it through a die, normally with a much smaller cross sectional area than the billet, with the metal emerging with the shape of the die MECH2636: Design and Manufacture 2 Extrusion and Drawing Metal Extrusion (2) For an “ideal” extrusion process (assuming no losses), ideal plastic work considerations suggest that the minimum force (F) exerted by the ram on the back of the billet is given by: Ao F = Ao y ln A f where y is the material flow stress, and Ao and Af are the initial and final cross-sectional areas of the billet respectively MECH2636: Design and Manufacture 2 Extrusion and Drawing Metal Extrusion (3) Two factors act to increase this ideal force in reality: friction and redundant work If friction is high, it is easier for the material to internally shear rather than flow over the die surface - this can be reduced by using a lubricant or a tapered die Direction of Extrusion MECH2636: Design and Manufacture 2 Extrusion and Drawing Metal Extrusion (3) Redundant work is the plastic work used in unnecessary shearing of the material - this normally increases with increasing extrusion die angle α Die Tapered Die α Extrusion Direction of Force Billet Extrusion However friction force reduces with increasing die angle due to reduced area of contact MECH2636: Design and Manufacture 2 Extrusion and Drawing Metal Extrusion (5) Minimum total force defines an optimum die angle The sum of the ideal, frictional and redundant work gives the total extrusion force which can be minimised to give an optimum die angle MECH2636: Design and Manufacture 2 Extrusion and Drawing Metal Extrusion (6) Backward extrusion is a variant of forward Backward extrusion, where the extrusion direction is reversed Hydrostatic extrusion uses a pressurised fluid to force the billet through a tapered die – this reduces friction MECH2636: Design and Manufacture 2 Extrusion and Drawing Example of Backward Extrusion Extruded Blank shell => MECH2636: Design and Manufacture 2 Extrusion and Drawing Metal Extrusion (7) Extrusion defects: – surface cracking (speed too high) – skin inclusion (friction too high) Design rules: – Solid shapes easiest and cheapest – Uniform wall thickness – Fillet radii on corners – Complicated shapes may be best achieved by joining two simpler extrusions (see next slide) MECH2636: Design and Manufacture 2 Extrusion and Drawing Example: Aluminium Window Frame made from 2 Extrusions joined with Sealing Strip MECH2636: Design and Manufacture 2 Extrusion and Drawing Die Drawing (1) Very similar to forward extrusion except the work is pulled through the die rather than pushed through it Normally done cold so improved material properties result Normally a finishing operation Can be used for small cross sectional areas Almost exclusively used for tube, wire and rod MECH2636: Design and Manufacture 2 Extrusion and Drawing Die Drawing (2) Die drawing of circular rod Tube drawing MECH2636: Design and Manufacture 2 Extrusion and Drawing Die Drawing (3) Similar to forward extrusion, the ideal drawing force can be calculated from: Ao F = A f y ln A f where y is the material flow stress, and Ao and Af are the initial and final cross- sectional areas of the billet respectively Again actual force will be greater due to redundant work and friction! MECH2636: Design and Manufacture 2 Extrusion and Drawing Die drawing –worked example A round rod of annealed 302 stainless steel is being drawn from a diameter of 10 mm down to 8 mm at a speed of 0.5 m/s. Assuming that the frictional and redundant work together are equal to 40% of the ideal work of deformation, calculate the total power required to perform this operation. The flow stress of the material is 785 MPa. MECH2636: Design and Manufacture 2 Extrusion and Drawing Diagram of process: MECH2636: Design and Manufacture 2 Extrusion and Drawing Calculate area reduction: Now calculate true strain: MECH2636: Design and Manufacture 2 Extrusion and Drawing Calculate “ideal” (minimum) draw load from: Ao F = A f y ln A f We have: 𝐴𝑓 = 𝜋𝑟 2 = 𝜋42 ≈ 50.3 mm2 Also: σ𝑦 = 785 𝑀𝑃𝑎 = 785 𝑁/mm2 Therefore: F = 50.3 × 785 × 0.445 = 17,571 𝑁 𝐹 ≈ 1.75 𝑡𝑜𝑛𝑛𝑒𝑠 MECH2636: Design and Manufacture 2 Extrusion and Drawing Now calculate the ideal (minimum) power required (without redundant work/friction): “Ideal” power: Fv = 17500 × 0.5 = 8750 𝑊 (remember Power = Force x Velocity in a linear system) But frictional and redundant work together equal 40% of the ideal work. Therefore: Actual power MECH2636: Design and Manufacture 2 Extrusion and Drawing In reality, amount of area reduction in die drawing limited by tensile strength of drawn product rather than power required. In the above example, we can calculate the tensile stress in the drawn rod as follows: CSA of die drawn rod: 𝐴𝑓 =50.3 mm2 Ideal drawing force: F = 17 571 𝑁 Actual drawing force: F = 1.4 × 17 571 = 24 600 𝑁 Therefore, tensile stress in drawn rod given by: 24600 σ= = 489 𝑁/mm2 = 489 MPa 50.3 Is this stress sufficient to cause tensile failure of the drawn rod? MECH2636: Design and Manufacture 2 Extrusion and Drawing Copyright Notice This presentation is protected by UK and international copyright law. Reproduction and distribution of this presentation without written permission from the originator is prohibited. Copyright © 2024 University of Leeds UK. All rights reserved. MECH2636: Design and Manufacture 2 School Solid of Mechanical Deformation Engineering Processes FACULTY OF ENGINEERING MECH2636: Design and Manufacture 2 Solid Deformation Processes 1. Forging MECH2636: Design and Manufacture 2 Solid Deformation Processes Learning objectives By the end of these screencasts, you should be able to describe the fundamental principles of some important solid deformation processes (forging, rolling, extrusion, drawing) and also be able to carry out some simple calculations related to these processes. Refer: Schmid & Kalpakjian - Chapters 13, 14 & 15 MECH2636: Design and Manufacture 2 Solid Deformation Processes Solid Deformation Processes This section concerns the forming of parts which have relatively high volume to surface area ratio by the following processes: – Forging – Rolling – Extrusion – Drawing All these processes basically involve deforming material in the solid phase (but can be either “cold” or “hot” forming) MECH2636: Design and Manufacture 2 Solid Deformation Processes Cold Working “Cold” working is carried out at temperatures below about 30% of the absolute melting temperature of the material Cold working can improve the strength & hardness of materials (but reduces ductility!) Cold working also gives better dimensional accuracy and surface finish than hot working (no thermal contraction or residual thermal stresses, no oxide surface layer) MECH2636: Design and Manufacture 2 Solid Deformation Processes Hot Working “Hot” is normally defined as being at a temperature above 60% of the melting point of the material (absolute Kelvin scale) where the material is able to recrystallise Hot working can give improved mechanical properties as the grain structure is refined, and also reduces the forces required as the metal flows more easily at the high temperature (reduced flow stress) “Warm” working is between 30% and 60% of the absolute melt temperature MECH2636: Design and Manufacture 2 Solid Deformation Processes Metal forging Origins of forging lie with blacksmiths’ trade - bulk deformation process normally involving the compression of metal between two surfaces Forging can be carried out with the metal at room temperature or at elevated temperature - depends on the mechanical properties required and the forces available MECH2636: Design and Manufacture 2 Solid Deformation Processes Open die forging (1) Workpiece (normally cylindrical) placed between two die surfaces and compressed Most common process known as “upsetting” The shape that results from upsetting depends on the friction between the die surfaces Low friction results in more homogeneous deformation MECH2636: Design and Manufacture 2 Solid Deformation Processes Upsetting (1) MECH2636: Design and Manufacture 2 Solid Deformation Processes Upsetting (2) High friction results in barrelling of workpiece Barrelling can also occur as a result of upsetting a hot workpiece between cold dies For a cylindrical workpiece of instantaneous radius & height r & h, plastic flow stress y and coefficient of friction , the upsetting force (F) can be estimated from: ( ) F y r 1 + 2 2r 3h MECH2636: Design and Manufacture 2 Solid Deformation Processes Upsetting – worked example (Ref: K & S – example 14.1) A cylindrical specimen made of annealed 3135 steel has an initial diameter of 150 mm and is 100 mm high. It is upset at room temperature by open-die forging with flat dies to a height of 50 mm. Assuming that the material flow (yield) stress is 950 MPa and the coefficient of friction is 0.2, estimate the upsetting force at the end of the stroke. MECH2636: Design and Manufacture 2 Solid Deformation Processes Use constancy of volume to calculate final diameter of billet (ignoring “barrelling”): MECH2636: Design and Manufacture 2 Solid Deformation Processes Use standard formula to calculate upsetting force: MECH2636: Design and Manufacture 2 Solid Deformation Processes Open die forging (2) Other common open die processes are: – drawing out – piercing – cutting Geometries created by open die forging will often be post-processed by closed die forging to create the final shape MECH2636: Design and Manufacture 2 Solid Deformation Processes Closed die forging (1) More accurate and better surface finish than open die forging Two forms: conventional and precision forging In conventional forging, excess material is provided in the die and a flash is produced The flash is generally under a great deal of pressure giving a large resistance to radial flow - this pressure encourages the filling of die cavities MECH2636: Design and Manufacture 2 Solid Deformation Processes Conventional closed die forging (2) MECH2636: Design and Manufacture 2 Solid Deformation Processes Conventional Forged Synchroniser Ring Raw Material Flash As forged Trimmed – flash removed MECH2636: Design and Manufacture 2 Solid Deformation Processes Precision die forging (1) In precision die forging, the amount of material processed is precisely that required to fill the die cavity Precision forging creates no flash, so the volume of metal used needs to be accurately controlled Precision forging also involves higher loads and harder tooling than conventional forging, but reduces subsequent machining MECH2636: Design and Manufacture 2 Solid Deformation Processes Precision die forging (2) Because of these higher loads, precision forging tends to be used most frequently with the softer Al & Mg alloys rather than steels The forces required in precision die forging are difficult to determine because of the complex deformation taking place, but broadly they increase with the die cross-sectional area and the flow stress of the material As with open die forging, friction must be minimised in precision die forging MECH2636: Design and Manufacture 2 Solid Deformation Processes Sequential Forging Where a press in incapable of supplying the load required to form a component in one go, a number of forging operations may be carried out sequentially to gradually change the shape of the component MECH2636: Design and Manufacture 2 Solid Deformation Processes Example of Sequential Forging: Upsetting followed by Precision Forging Upsetting with patterned dies: Followed by precision die forging: MECH2636: Design and Manufacture 2 Solid Deformation Processes Heading and Coining Other forging operations: – Coining - origins in ancient Rome Coining – Heading - commonly used to form bolt heads Heading MECH2636: Design and Manufacture 2 Solid Deformation Processes Flaws in Forging Most common flaws are: – surface cracks where the material has either been cooled too quickly or over- strained – “laps” arising from buckling of thin sections of the forging – “cold shuts” where the die design allows the metal to fold back on itself away from the heated die MECH2636: Design and Manufacture 2 Solid Deformation Processes Laps and Cold Shuts Bigger fillet radius avoids possible cold shut Possible cold shut MECH2636: Design and Manufacture 2 Solid Deformation Processes Design rules for forgings (1) Choose appropriate fillet and corner radii to allow metal to flow more easily Specify reasonable draft angles to allow easy removal of forging from die All forged components will have somewhat anisotropic material properties, caused by the flow of grains during the process - die parting line should be chosen with this in mind MECH2636: Design and Manufacture 2 Solid Deformation Processes Metal Flow in Forging Upper figures compare grain structure from forged and machined parts Lower left figure shows grain structure from good choice of die parting line. Lower right figure shows less ideal grain structure + possible weakness at parting line from poor selection of its location. MECH2636: Design and Manufacture 2 Solid Deformation Processes Design rules for forgings (2) Parting line should usually pass through the maximum area possible (easier for metal to flow outwards rather than up) There are minimum recommended thicknesses for ribs and webs dependent on material Punchout holes can be used to reduce weight and/or provide clearance MECH2636: Design and Manufacture 2 Solid Deformation Processes Copyright Notice This presentation is protected by UK and international copyright law. Reproduction and distribution of this presentation without written permission from the originator is prohibited. Copyright © 2024 University of Leeds UK. All rights reserved.