Thermodynamics (VIT Bhopal Lecture Notes) PDF
Document Details
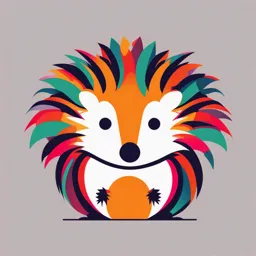
Uploaded by SuccessfulSerpentine2699
VIT Bhopal
Tags
Summary
These lecture notes provide an overview of thermodynamics, explaining key concepts like thermodynamic systems, surroundings, and the universe. The document covers fundamental definitions and principles related to thermodynamics.
Full Transcript
Thermodynamics Thermodynamics Science of thermodynamics: Industrial revolution in the late 1700s or early 1800s Up till then, heavy work was performed by horses: horsepower Fantastic new invention: Heat Engine, thermodynamics: to gain the maximum efficiency from such heat engines Succes...
Thermodynamics Thermodynamics Science of thermodynamics: Industrial revolution in the late 1700s or early 1800s Up till then, heavy work was performed by horses: horsepower Fantastic new invention: Heat Engine, thermodynamics: to gain the maximum efficiency from such heat engines Successful science: modern day heat engines, Bugatti Veyron: world’s fastest production car, 883 kilowatts, 1184 brake horsepower, 268 miles/hour 2 Thermodynamic Definitions Thermodynamic System A thermodynamic system is defined as any part of the Universe under consideration. It may be something as similar as a beaker of water, a chemical reaction taking place in a test tube, oil refinery or as complicated as an entire galaxy. 3 Thermodynamic Definitions Thermodynamic Surroundings Thermodynamics surroundings are defined as everything other than the thermodynamic system. In other words, the entire rest of the universe. The Universe The universe is therefore the system plus the surroundings. 4 Thermodynamic Definitions The Vastness of the Universe Milky Way is just one of billions of galaxies in the universe. Recently, scientists mapped the 100,000 or so galaxies near the Milky Way and found that it's part of a broader supercluster called Laniakea. The observable Universe: Sphere with a radius 4.66 x 1010 light years, a light years is 9.46 x 1015 m. 5 Thermodynamic Definitions Thermodynamic Surroundings Thermite reaction: Intensely exothermic, used even today to weld railway lines in remote location. 𝑭𝒆𝟐 𝑶𝟑 + 𝟐𝑨𝒍 → 𝟐𝑭𝒆 + 𝑨𝒍𝟐 𝑶𝟑 Large amount of energy released, but impact of this on next town is negligible. Supernova explosion: most violent energetic process, dying stars explode The impact on distant galaxies is negligible. How many times you have observed this phenomena in your life? 6 Thermodynamic Definitions Thermodynamic Surroundings Surroundings are assumed to be infinite and remain at constant temperature and pressure. The vast size of the universe validates this assumption. Boundary Conditions The boundary may be actual or notional. It controls transfer of work, heat, and matter from the system to the surroundings and vice-versa. The boundary may or may not impose restrictions on such transfers. 7 Thermodynamic Definitions Open, Closed, and Isolated Systems Open System: An open system may exchange both energy and matter with its surroundings. Changes in composition are therefore possible. Closed System: A closed system may exchange energy but not matter with its surroundings. Pressure build-up is a distinct possibility. Isolated System: An isolated system may exchange neither energy nor matter with its surroundings. Pressure build-up is a possibility. 8 Thermodynamic Definitions Diathermic and Adiabatic Systems/Walls A diathermic system allows heat flow into or out of the system. An adiabatic system prevents heat flow into or out of the system. 9 Thermodynamic Definitions Isothermal, Isobaric, and Isochoric Processes Isothermal implies constant temperature, T. Isobaric implies constant pressure, P. Isochoric implies constant volume, V. State Functions A state function describes the state of the system. The following are all state functions: Pressure, P; Volume, V; Temperature, T; Mass, m; Quantity, n Internal Energy, U; Enthalpy, H; Entropy, S; Gibbs Energy, G 10 Thermodynamic Definitions Path Functions Random molecular motion A state function describes the current state of a system. How the system came to be in that particular state is of no consequence. Functions governing transition between the states are called path functions. Heat, q & Work, w: are both forms of energy. State of a system is changed Uniform molecular motion by supply or removal of energy in the form of heat or work. 11 Thermodynamic Definitions Heat and Work Conventions Path functions are positive when energy enters the system. Path functions are negative when energy leaves the system. They are often defined as: Heat supplied to the system, qin Mechanical work done on the system, Won 12 Laws of Thermodynamics The Zeroth Law of Thermodynamics and Temperature Ironically named 0th law; last of the four laws to be discovered. Gives true meaning of temperature. The First Law of Thermodynamics Developed around 1850 by Rudolf Clausius. Adaption of the Law of Conservation of Energy for Thermodynamic Systems. Defined change in the state function, internal energy of the system based on the energy flow into or out of the system Lead to the definition of a new state function called enthalpy. 13 Laws of Thermodynamics The Second Law of Thermodynamics Developed around 1850 by Rudolf Clausius by following initial work of Sadi Carnot in 1824. Describes the direction in which all processes spontaneously occur, e. g. a hot object loses heat to the surroundings. New state function: Entropy, fundamentally measures disorder Third Law of Thermodynamics Developed by German chemist Walter Nernst in 1912; While considering the behaviour of matter at absolute zero. Lead to the definition of zero on the entropy scale; determination of 14 absolute entropy. The Zeroth Law of Thermodynamics Measurement of Temperature Measured routinely by a thermometer; Think how the thermometer works? Zeroth Law When two objects are separately in thermodynamic equilibrium with a third object, they are in thermodynamic equilibrium with each other. Whenever two objects are in contact with one another, energy will flow between them until they reach a state of thermodynamic equilibrium. Upon reaching this state we say that the two objects are at the same temperature. 15 The Zeroth Law of Thermodynamics This sounds obvious but it has vital ramifications for the measurement of temperature by thermometer. The obvious nature of this statement explains why it did not become a law of thermodynamics until well after the other three laws. 16 The First Law of Thermodynamics and Enthalpy Rudolf Clausius, 1850. Law of Conservation of Energy: Energy can neither be created nor destroyed, merely changed from one form to another. Used this law for thermodynamic systems. Every thermodynamic system (even all matter) possesses an internal energy, U. Δ𝑈 = 𝑞𝑖𝑛 + 𝑤𝑜𝑛 Chemical work done during a chemical reaction Emerging gas performs a chemical work 17 The Work Done by an Expanding gas 𝑤 = 𝐹𝑑 𝐹 𝑝= 𝐴 𝐹 = 𝑝𝐴 𝑤 = 𝑝𝐴𝑑 𝑤𝑜𝑛 = −𝑝Δ𝑉 𝑤 = 𝑝Δ𝑉 18 The First Law of Thermodynamics Δ𝑈 = 𝑞𝑖𝑛 + 𝑤𝑜𝑛 𝑤𝑜𝑛 = −𝑝Δ𝑉 Δ𝑈 = 𝑞𝑖𝑛 − 𝑝Δ𝑉 Expansion work clearly depends on 𝑝 and Δ𝑉. Free Expansion Δ𝑈 = 𝑞𝑖𝑛 − 𝑝Δ𝑉; but in space 𝑝 = 0 and Δ𝑈 = 𝑞𝑖𝑛. However, relatively very few experiments are carried out in space. Reactions at Constant Volume (Domain of Heavy Industries) Δ𝑈 = 𝑞𝑖𝑛 − 𝑝Δ𝑉; but at constant volume Δ𝑉 = 0 and Δ𝑈 = 𝑞𝑣. Constant volume reactors must withstand massive pressure changes, heavy chemical industries; very expensive. 19 The First Law of Thermodynamics Reactions at Constant Pressure (Domain of Chemists) Δ𝑈 = 𝑞𝑖𝑛 − 𝑝Δ𝑉 Δ𝑈 + 𝑝Δ𝑉 = 𝑞𝑖𝑛 at constant pressure. Now we define a new state function, enthalpy: 𝐻 = 𝑈 + 𝑝𝑉 ∴ ∆𝐻 = ∆𝑈 + ∆ 𝑝𝑉 , Use product rule ⇒ ∆𝐻 = ∆𝑈 + 𝑝∆𝑉 + 𝑉∆𝑝, but at constant pressure ∆𝑝 = 0, 𝑉∆𝑝 = 0 ∴ ∆𝐻 = ∆𝑈 + 𝑝∆𝑉 ∆𝐻 = 𝑞𝑝 20 Ideal Gas Vs Real Gas Ideal Gas Follows gas laws, completely abide kinetic molecular theory Occupy zero volume; no attractive forces No true ideal gas Real Gas Does not behave according to the kinetic molecular theory Deviation from Ideal Gas; Compressibility Factor (Z) PV/RT plotted against pressure for 1mol of a gas at three different temperatures An ideal gas would have a value of 1 for that ratio at all temperatures and pressures and the graph would simply be a horizontal line. The magnitude of the deviations from ideality is greatest for the gas at 200 K and least for the gas at 1000 K. Reversible Isothermal Expansion of an Ideal Gas ❑ Leads to maximum amount of work performed by the gas. Ideal Gas Equation: p𝑉 = 𝑛𝑅𝑇 : p = Pressure; V = Volume; T = Absolute temperature measure in K; n = quantity; R = Ideal gas constant, 8.314 J K-1 mol-1 𝑛𝑅𝑇 ⇒p= 𝑣 Consider 1 mol an ideal gas at 298 K p = 2477.572 J/V Using the above equation, calculate the pressure of the gas for volumes 1, 3, 5, 7, 9, and 11 m3. Isothermal Expansion External pressure, p = 2478 Pa Volume = 1 m3. Now imagine the gas pressure suddenly drops to 826 Pa. What happens to the gas? 24 Isothermal Expansion ❑ Fits ideal gas equation perfectly ❑ The gas is performing work as it expands; the system is performing work: Won = ?? 25 Isothermal Expansion ❑How to change the expansion, so that the gas performs more work? 26 Reversible Isothermal Expansion 27 won for Reversible Isothermal Expansion 𝑤𝑜𝑛 = −𝑝𝑑𝑉 From previous slides But, p𝑉 = 𝑛𝑅𝑇 𝑛𝑅𝑇 So, 𝑝= 𝑣 𝑛𝑅𝑇 𝑤𝑜𝑛 = − 𝑑𝑉 𝑣 Now consider an expansion from Vi to Vf 𝑉𝑓 𝑑𝑉 𝑤𝑜𝑛 = −𝑛𝑅𝑇 න 𝑉 𝑉𝑖 28 won for Reversible Isothermal Expansion 𝑉𝑓 𝑑𝑉 𝑤𝑜𝑛 = −𝑛𝑅𝑇 න 𝑉 𝑉𝑖 𝑉𝑓 𝑤𝑜𝑛 = −𝑛𝑅𝑇 ln 𝑉𝑖 𝑤𝑜𝑛 = - 1 mol × 8.314 J K-1 mol-1 × 298 K × ln(11) 𝑤𝑜𝑛 = ? ? ❑Reversible change is a theoretical construct. ❑Determines maximum possible expansion work. 29 Heating Objects: Heat Capacity Heat capacity, C, is the heat energy required to raise the temperature of an object by 1o C or 1 K. Measured in J/K. 𝐶 = 𝑞ΤΔ 𝑇 30 31 32 33 34 35 36 37 38 39 Spontaneous Change A spontaneous change is one that occurs without a continuous input of energy from outside the system. All chemical processes require energy (activation energy) to take place, but once a spontaneous process has begun, no further input of energy is needed. A nonspontaneous change occurs only if the surroundings continuously supply energy to the system. If a change is spontaneous in one direction, it will be nonspontaneous in the reverse direction. Spontaneous Processes Processes that are spontaneous in one direction are nonspontaneous in the reverse direction. Processes that are spontaneous at one temperature may be nonspontaneous at other temperatures. DH Does Not Predict Spontaneous Change A spontaneous change may be exothermic or endothermic. Spontaneous exothermic processes include: freezing and condensation at low temperatures, combustion reactions, oxidation of iron and other metals. Spontaneous endothermic processes include: melting and vaporization at higher temperatures, dissolving of most soluble salts. The sign of ΔH does not by itself predict the direction of a spontaneous change. 43 Entropy Entropy (S) is a term coined by Rudolph Clausius in the 19th century. Entropy can be thought of as a measure of the randomness of a system. It is related to the various modes of motion in molecules. 44 The Second Law of Thermodynamics The sign of ΔS for a reaction does not, by itself, predict the direction of a spontaneous reaction. If we consider both the system and the surroundings, we find that all real processes occur spontaneously in the direction that increases the entropy of the universe. For a process to be spontaneous, a decrease in the entropy of the system must be offset by a larger increase in the entropy of the surroundings. DSuniv = DSsys + DSsurr > 0 For enthalpy there is no zero point; we can only measure changes in enthalpy. For entropy there is a zero point, and we can determine absolute entropy values. 45 The Third Law of Thermodynamics A perfect crystal has zero entropy at absolute zero. Ssys = 0 at 0 K A “perfect” crystal has flawless alignment of all its particles. At absolute zero, the particles have minimum energy, so there is only one microstate. S = k lnW = k ln 1 = 0 Factors Affecting Entropy Entropy depends on temperature. For any substance, S°increases as temperature increases. Entropy depends on the physical state of a substance. S°increases as the phase changes from solid to liquid to gas. The formation of a solution affects entropy. Entropy is related to atomic size and molecular complexity. Remember to compare substances in the same physical state. The increase in entropy during phase changes from solid to liquid to gas. Entropy and Structure For allotropes, S°is higher in the form that allows the atoms more freedom of motion. S°of graphite is 5.69 J/mol·K, whereas S°of diamond is 2.44 J/mol·K. Predicting Relative Entropy Values PROBLEM: Choose the member with the higher entropy in each of the following pairs, and justify your choice [assume constant temperature, except in part (e)]: (a) 1 mol of SO2(g) or 1 mol of SO3(g) (b) 1 mol of CO2(s) or 1 mol of CO2(g) (c) 3 mol of O2(g) or 2 mol of O3(g) (d) 1 mol of KBr(s) or 1 mol of KBr(aq) (e) seawater at 2°C or at 23°C PLAN: In general, particles with more freedom of motion have more microstates in which to disperse their kinetic energy, so they have higher entropy. Raising the temperature or having more particles increases entropy. SOLUTION: (a) 1 mol of SO3(g). For equal numbers of moles of substances with the same types of atoms in the same physical state, the more atoms in the molecule the higher the entropy. (b) 1 mol of CO2(g). For a given substance, entropy increases as the phase changes from solid to liquid to gas. (c) 3 mol of O2(g). The two samples contain the same number of oxygen atoms but different numbers of molecules. Although each O3 molecule is more complex than each O2 molecule, the greater number of molecules dominates because there are many more microstates possible for 3 mol of particles than for 2. (d) 1 mol of KBr(aq). The two samples have the same number of ions, but their motion is more limited and their energy less dispersed in the solid than in the solution. An ionic substance in solution usually has a higher entropy than the solid. (e) Seawater at 23°C. Entropy increases with rising temperature. Entropy Changes in the System The standard entropy of reaction, ΔS°rxn, is the entropy change that occurs when all reactants and products are in their standard states. DS°rxn = SmS°products - SnS°reactants where m and n are the amounts (mol) of products and reactants, given by the coefficients in the balanced equation. Calculating the Standard Entropy of Reaction, DS°rxn PROBLEM: Predict the sign of ΔS°rxn and calculate its value for the combustion of 1 mol of propane at 25°C. C3H8(g) + 5O2(g) → 3CO2(g) + 4H2O(l) SOLUTION: ΔS°rxn = [(3 mol CO2)(S° of CO2) + (4 mol H2O)(S° of H2O)] - [(1 mol C3H8)(S° of C3H8) + (5 mol O2)(S° of O2) = [(3mol)(213.7J/K·mol) + (4 mol)(69.9J/K·mol)] – [(1mol)(269.9 J/K·mol) + (5 mol)(205.0 J/K·mol) = -374 J/K Entropy Changes in the Surroundings A decrease in the entropy of the system is outweighed by an increase in the entropy of the surroundings. The surroundings function as a heat source or heat sink. In an exothermic process, the surroundings absorbs the heat released by the system, and Ssurr increases. qsys < 0; qsurr > 0 and ΔSsurr > 0 In an endothermic process, the surroundings provides the heat absorbed by the system, and Ssurr decreases. qsys > 0; qsurr < 0 and ΔSsurr < 0 Temperature at which Heat is Transferred For any reaction, qsys = -qsurr, the heat transferred is specific for the reaction and is the same regardless of the temperature of the surroundings. DSsurr = - qsys T DSsurr = - DHsys for a process at constant P. T Determining Reaction Spontaneity PROBLEM: At 298 K, the formation of ammonia has a negative DS°sys; N2(g) + 3H2(g) → 2NH3(g); DS°sys = -197 J/K Calculate DS°univ, and state whether the reaction occurs spontaneously at this temperature. SOLUTION: DH°rxn = [(2 mol)(DH°f of NH3)] - [(1 mol)(DH°f of N2) + (3 mol)(DH°f of H2)] = [(2 mol)(-45.9 kJ/mol)] - [(1 mol)(0 kJ/mol) + (3 mol)(0 kJ/mol)] = - 91.8 kJ -91.8 kJ x 1000 J DHsys 1 kJ DSsurr = - =- = 308 J/K T 298 K DS°univ = DS°sys + DS°surr = -197 J/K + 308 J/K = 111 J/K 57 58 59 60 61 62 63 64 65 66 67 68 69 70 71 72 73 74 75 76 77 78 79 Hess’s Law 80 81 82 83 84 85 86 87 88 89 90 Self-Study: Maxwell Relations and Gibbs Helmholtz Equation 91 Thank you! 92