Civil Engineering: A Very Short Introduction PDF
Document Details
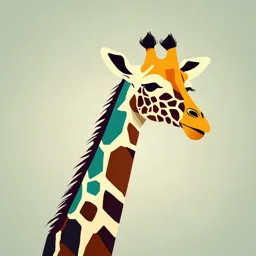
Uploaded by TrustworthySugilite363
2012
David Muir Wood
Tags
Related
- CETE 011 Civil Engineering Technology Module 01-05 PDF
- Geology for Civil Engineers Lecture Notes PDF
- CVE 236: Civil Engineering Materials - Concrete Technology PDF
- Chapter 1 - Introduction to Concrete Technology PDF
- Chapter 1 - Introduction to Concrete Technology PDF
- Advanced Materials and Joining Course 2024/2025 PDF
Summary
This book offers a very short introduction to civil engineering. Written by an expert, it covers various aspects of this field. The book is part of a series of concise overviews about different topics in the humanities, history, philosophy, religion, science, and the social sciences.
Full Transcript
Great Clarendon Street, Oxford, OX2 6DP, United Kingdom Oxford University Press is a department of the University of Oxford. It furthers the University’s objective of excellence in research, scholarship, and education by publishing worldwide. Oxford is a registered trade mark of Oxford University...
Great Clarendon Street, Oxford, OX2 6DP, United Kingdom Oxford University Press is a department of the University of Oxford. It furthers the University’s objective of excellence in research, scholarship, and education by publishing worldwide. Oxford is a registered trade mark of Oxford University Press in the UK and in certain other countries © David Muir Wood 2012 The moral rights of the author have been asserted First Edition published in 2012 Impression: 1 All rights reserved. No part of this publication may be reproduced, stored in a retrieval system, or transmitted, in any form or by any means, without the prior permission in writing of Oxford University Press, or as expressly permitted by law, by licence or under terms agreed with the appropriate reprographics rights organization. Enquiries concerning reproduction outside the scope of the above should be sent to the Rights Department, Oxford University Press, at the address above You must not circulate this work in any other form and you must impose this same condition on any acquirer British Library Cataloguing in Publication Data Data available Library of Congress Cataloging in Publication Data Data available ISBN 978–0–19–957863–4 Printed in Great Britain by Ashford Colour Press Ltd, Gosport, Hampshire CIVIL ENGINEERING: A Very Short Introduction VERY SHORT INTRODUCTIONS are for anyone wanting a stimulating and accessible way in to a new subject. They are written by experts, and have been published in more than 25 languages worldwide. The series began in 1995 and now represents a wide variety of topics in history, philosophy, religion, science, and the humanities. The VSI library now contains more than 300 volumes—a Very Short Introduction to everything from ancient Egypt and Indian philosophy to conceptual Heathrow Airport Terminal 5li: solid black 0.1em; border-art and cosmology—and will continue to grow in a variety of disciplines. Very Short Introductions available now: ADVERTISING Winston Fletcher AFRICAN HISTORY John Parker and Richard Rathbone AGNOSTICISM Robin Le Poidevin AMERICAN IMMIGRATION David A. Gerber AMERICAN POLITICAL PARTIES AND ELECTIONS L. Sandy Maisel THE AMERICAN PRESIDENCY Charles O. Jones ANAESTHESIA Aidan O’Donnell ANARCHISM Colin Ward ANCIENT EGYPT Ian Shaw ANCIENT GREECEPaul Cartledge ANCIENT PHILOSOPHY Julia Annas ANCIENT WARFARE Harry Sidebottom ANGELS David Albert Jones ANGLICANISM Mark Chapman THE ANGLO-SAXON AGE John Blair THE ANIMAL KINGDOM Peter Holland ANIMAL RIGHTS David DeGrazia THE ANTARCTIC Klaus Dodds ANTISEMITISM Steven Beller ANXIETY Daniel Freeman and Jason Freeman THE APOCRYPHAL GOSPELS Paul Foster ARCHAEOLOGY Paul Bahn ARCHITECTURE Andrew Ballantyne ARISTOCRACY William Doyle ARISTOTLE Jonathan Barnes ART HISTORY Dana Arnold ART THEORY Cynthia Freeland ATHEISM Julian Baggini AUGUSTINE Henry Chadwick AUSTRALIA Kenneth Morgan AUTISM Uta Frith THE AZTECS Davíd Carrasco BARTHES Jonathan Culler BEAUTY Roger Scruton BESTSELLERS John Sutherland THE BIBLE John Riches BIBLICAL ARCHAEOLOGY Eric H. Cline BIOGRAPHY Hermione Lee THE BLUES Elijah Wald THE BOOK OF MORMON Terryl Givens BORDERS Alexander C. Diener THE BRAIN Michael O’Shea BRITISH POLITICS Anthony Wright BUDD=as. There HA Michael Carrithers BUDDHISM Damien Keown BUDDHIST ETHICS Damien Keown CANCER Nicholas James CAPITALISM James Fulcher CATHOLICISM Gerald O’Collins THE CELL Terence Allen and Graham Cowling THE CELTS Barry Cunliffe CHAOS Leonard Smith CHILDREN’S LITERATURE Kimberley Reynolds CHINESE LITERATURE Sabina Knight CHOICE THEORY Michael Allingham CHRISTIAN ART Beth Williamson CHRISTIAN ETHICS D. Stephen Long CHRISTIANITY Linda Woodhead CITIZENSHIP Richard Bellamy CIVIL ENGINEERINGDavid Muir Wood CLASSICAL MYTHOLOGY Helen Morales CLASSICS Mary Beard and John Henderson CLAUSEWITZ Michael Howard THE COLD WAR Robert McMahon COLONIAL LATIN AMERICAN LITERATURE Rolena Adorno COMMUNISM Leslie Holmes THE COMPUTER Darrel Ince THE CONQUISTADORS Matthew Restall and Felipe Fernández-Armesto CONSCIENCE Paul Strohm CONSCIOUSNESS Susan Blackmore CONTEMPORARY ART Julian Stallabrass CONTINENTAL PHILOSOPHY Simon Critchley COSMOLOGY Peter Coles CRITICAL THEORY Stephen Eric Bronner THE CRUSADES Christopher Tyerman CRYPTOGRAPHY Fred Piper and Sean Murphy THE CULTURAL REVOLUTION Richard Curt Kraus DADA AND SURREALISM David Hopkins DARWIN Jonathan Howard THE DEAD SEA SCROLLS Timothy Lim DEMOCRACY Bernard Crick DERRIDA Simon Glendinning DESCARTES Tom Sorell DESERTS Nick Middleton DESIGN John Heskett DEVELOPMENTAL BIOLOGY Lewis Wolpert THE DEVIL Darren Oldridge DICTIONARIES Lynda Mugglestone">114–1st their DINOSAURS David Norman DIPLOMACY Joseph M. Siracusa DOCUMENTARY FILM Patricia Aufderheide DREAMING J. Allan Hobson DRUGS Leslie Iversen DRUIDS Barry Cunliffe EARLY MUSIC Thomas Forrest Kelly THE EARTH Martin Redfern ECONOMICS Partha Dasgupta EGYPTIAN MYTH Geraldine Pinch EIGHTEENTH-CENTURY BRITAIN Paul Langford THE ELEMENTS Philip Ball EMOTION Dylan Evans EMPIRE Stephen Howe ENGELS Terrell Carver ENGINEERING David Blockley ENGLISH LITERATUREJonathan Bate ENVIRONMENTAL ECONOMICS Stephen Smith EPIDEMIOLOGY Rodolfo Saracci ETHICS Simon Blackburn THE EUROPEAN UNION John Pinder and Simon Usherwood EVOLUTION Brian and Deborah Charlesworth EXISTENTIALISM Thomas Flynn FASCISM Kevin Passmore FASHION Rebecca Arnold FEMINISM Margaret Walters FILM Michael Wood FILM MUSIC Kathryn Kalinak THE FIRST WORLD WAR Michael Howard FOLK MUSIC Mark Slobin FORENSIC PSYCHOLOGY David Canter FORENSIC SCIENCE Jim Fraser FOSSILS Keith Thomson FOUCAULT Gary Gutting FREE SPEECH Nigel Warburton FREE WILL Thomas Pink FRENCH LITERATURE John D. Lyons THE FRENCH REVOLUTION William Doyle FREUD Anthony Storr FUNDAMENTALISM Malise Ruthven GALAXIES John Gribbin GALILEO Stillman Drake GAME THEORY Ken Binmore GANDHI Bhikhu Parekh GENIUS Andrew Robinson GEOGRAPHY John Matthews and David Herbert GEOPOLITI">CIVIL ENGINEERINGthMCS Klaus Dodds GERMAN LITERATURE Nicholas Boyle GERMAN PHILOSOPHY Andrew Bowie GLOBAL CATASTROPHES Bill McGuire GLOBAL ECONOMIC HISTORY Robert C. Allen GLOBAL WARMING Mark Maslin GLOBALIZATION Manfred Steger THE GOTHIC Nick Groom THE GREAT DEPRESSION AND THE NEW DEAL Eric Rauchway HABERMAS James Gordon Finlayson HEGEL Peter Singer HEIDEGGER Michael Inwood HERODOTUS Jennifer T. Roberts HIEROGLYPHS Penelope Wilson HINDUISM Kim Knott HISTORY John H. Arnold THE HISTORY OF ASTRONOMY Michael Hoskin THE HISTORY OF LIFE Michael Benton THE HISTORY OF MATHEMATICS Jacqueline Stedall THE HISTORY OF MEDICINE William Bynum THE HISTORY OF TIME Leofranc Holford-Strevens HIV/AIDS Alan Whiteside HOBBES Richard Tuck HUMAN EVOLUTION Bernard Wood HUMAN RIGHTS Andrew Clapham HUMANISM Stephen Law HUME A. J. Ayer IDEOLOGY Michael Freeden INDIAN PHILOSOPHY Sue Hamilton INFORMATION Luciano Floridi INNOVATION Mark Dodgson and David Gann INTELLIGENCE Ian J. Deary INTERNATIONAL MIGRATION Khalid Koser INTERNATIONAL RELATIONS Paul Wilkinson ISLAM Malise Ruthven ISLAMIC HISTORYAdam Silverstein ITALIAN LITERATURE Peter Hainsworth and David Robey JESUS Richard Bauckham JOURNALISM Ian Hargreaves JUDAISM Norman Solomon JUNG Anthony Stevens KABBALAH Joseph Dan KAFKA Ritchie Robertson KANT Roger Scruton KEYNES Robert Skidelsky KIERKEGAARDPatrick Gardiner supply of fresh water is oneMA. There THE KORAN Michael Cook LANDSCAPES AND GEOMORPHOLOGY Andrew Goudie and Heather Viles LANGUAGES Stephen R. Anderson LATE ANTIQUITY Gillian Clark LAW Raymond Wacks THE LAWS OF THERMODYNAMICS Peter Atkins LEADERSHIP Keith Grint LINCOLN Allen C. Guelzo LINGUISTICS Peter Matthews LITERARY THEORY Jonathan Culler LOCKE John Dunn LOGIC Graham Priest MACHIAVELLI Quentin Skinner MADNESS Andrew Scull MAGIC Owen Davies MAGNA CARTA Nicholas Vincent MAGNETISM Stephen Blundell THE MARQUIS DE SADE John Phillips MARTIN LUTHER Scott H. Hendrix MARX Peter Singer MATHEMATICS Timothy Gowers THE MEANING OF LIFE Terry Eagleton MEDICAL ETHICS Tony Hope MEDIEVAL BRITAIN John Gillingham and Ralph A. Griffiths MEMORY Jonathan K. Foster METAPHYSICS Stephen Mumford MICHAEL FARADAY Frank A. J. L. James MODERN ART David Cottington MODERN CHINA Rana Mitter MODERN FRANCE Vanessa R. Schwartz MODERN IRELAND Senia Pašeta MODERN JAPAN Christopher Goto-Jones MODERN LATIN AMERICAN LITERATURE Roberto González Echevarría MODERNISM Christopher Butler MOLECULES Philip Ball THE MONGOLS Morris Rossabi MORMONISM Richard Lyman Bushman MUHAMMAD Jonathan A. C. Brown MULTICULTURALISM Ali Rattansi MUSIC Nicholas Cook MYTH Robert A. Segal NATIONALISM Steven Grosby NELSON MANDELA Elleke Boehmer NEOLIBERALISM Manfred Steger and Ravi Roy THE NEW TESTAMENT Luke Timothy Johnson THE NEW TESTAMENT AS LITERATURE Kyle Keefer NEWTON Robert Iliffe NIETZSCHE Michael Tanner NINETEENTH-CENTURY BRITAIN Christopher Harvie and H. C. G. Matthew THE NORMAN CONQUEST George Garnett NORTH AMERICAN INDIANS Theda Perdue and Michael D. Green NORTHERN IRELAND Marc Mulholland NOTHING Frank Close NUCLEAR POWER Maxwell Irvine NUCLEAR WEAPONS Joseph M. Siracusa NUMBERS Peter M. Higgins OBJECTIVITYStephen Gaukroger THE OLD TESTAMENT Michael D. Coogan ORGANIZATIONS Mary Jo Hatch PAGANISM Owen Davies PARTICLE PHYSICS Frank Close PAUL E. P. Sanders PENTECOSTALISM William K. Kay THE PERIODIC TABLE Eric R. Scerri PHILOSOPHY Edward Craig PHILOSOPHY OF LAWRaymond Wacks PHILOSOPHY OF SCIENCE Samir Okasha PHOTOGRAPHY Steve Edwards PLAGUE Paul Slack PLANETS David A. Rothery PLANTS Timothy Walker PLATO Julia Annas POLITICAL PHILOSOPHY David Miller POLITICS Kenneth Minogue POSTCOLONIALISM Robert Young POSTMODERNISM Christopher Butler POSTSTRUCTURALISM Catherine Belsey PREHISTORY Chris Gosden PRESOCRATIC PHILOSOPHY Catherine Osborne PRIVACY Raymond Wacks PROBABILITY John Haigh PROGRESSIVISM Walter Nugent PROTESTANTISM Mark A. Noll PSYCHIATRY Tom Burns PSYCHOLOGY Gillian Butler and Freda McManus PURITANISM Francis J. Bremer THE QUAKERS Pink Dandelion QUANTUM THEORY John Polkinghorne RACISM Ali Rattansi RADIOACTIVITY Claudio Tuniz THE REAGAN REVOLUTION Gil Troy REALITY Jan Westerhoff THE REFORMATION Peter Marshall RELATIVITY Russell Stannard RELIGION IN AMERICA Timothy Beal THE RENAISSANCE Jerry Brotton RENAISSANCE ART Geraldine A. Johnson RISK Baruch Fischhoff and John Kadvany RIVERS Nick Middleton ROBOTICS Alan Winfield ROMAN BRITAIN Peter Salway THE ROMAN EMPIREChristopher Kelly THE ROMAN REPUBLIC David M. Gwynn ROMANTICISM Michael Ferber ROUSSEAU Robert Wokler RUSSELL A. C. Grayling RUSSIAN HISTORY Geoffrey Hosking RUSSIAN LITERATURE Catriona Kelly THE RUSSIAN REVOLUTION S. A. Smith SCHIZOPHRENIA Chris Frith and Eve Johnstone SCHOPENHAUER Christopher Janaway SCIENCE AND RELIGION Thomas Dixon SCIENCE FICTION David Seed THE SCIENTIFIC REVOLUTION Lawrence M. Principe SCOTLAND Rab Houston SEXUALITY Véronique Mottier SHAKESPEARE Germaine Greer SIKHISM Eleanor Nesbitt SLEEPSteven W. Lockley and Russell G. Foster SOCIAL AND CULTURAL ANTHROPOLOGY John Monaghan and Peter Just SOCIALISM Michael Newman SOCIOLOGY Steve Bruce SOCRATES C. C. W. Taylor THE SOVIET UNIONStephen Lovell THE SPANISH CIVIL WAR Helen Graham SPANISH LITERATURE Jo Labanyi SPINOZA Roger Scruton STARS Andrew King STATISTICS David J. Hand STEM CELLS Jonathan Slack STUART BRITAIN John Morrill SUPERCONDUCTIVITY Stephen Blundell TERRORISM Charles Townshend THEOLOGY David F. Ford THOMAS AQUINAS Fergus Kerr CIVIL ENGINEERINGthM">TOCQUEVILLE Harvey C. Mansfield TRAGEDY Adrian Poole TRUST Katherine Hawley THE TUDORS John Guy TWENTIETH-CENTURY BRITAIN Kenneth O. Morgan THE UNITED NATIONS Jussi M. Hanhimäki THE U.S. CONGRESS Donald A. Ritchie THE U.S. SUPREME COURT Linda Greenhouse UTOPIANISM Lyman Tower Sargent THE VIKINGS Julian Richards VIRUSES Dorothy H. Crawford WITCHCRAFT Malcolm Gaskill WITTGENSTEIN A. C. Grayling WORLD MUSIC Philip Bohlman THE WORLD TRADE ORGANIZATION Amrita Narlikar WRITING AND SCRIPT Andrew Robinson Available soon: GOVERNANCE Mark Bevir THE ORCHESTRA D. Kern Holoman WORK Stephen Fineman AMERICAN HISTORY Paul S. Boyer SPIRITUAL Engineering David Muir Wood Engineering Acknowledgements Early versions of the text of this book have been read by Margaret Abel, Hugh Balchin, Carol Collins, Julia Elton, Ted and Virginia Khan, Adrian Mathias, Helen Muir Wood, James Sutherland, and Sue Vardy. I am immensely grateful to all of them, but especially to Julia and James, for the thoughtful and detailed comments that they provided. Whether they will feel that I have adequately reacted to their suggestions I cannot tell – the supply of fresh water is one19seW responsibility for the final text rests with me. Civil Contents List of illustrations Introduction 1 Materials of civil engineering 2 Water and waste 3 ‘Directing the great sources of power in nature’ 4 Concept – technology – realization 5 Robustness 6 Civil engineering: looking forward Sources List of illustrations 1 Tower of Pisa 2 Todaiji temple, Nara 3 Corbelling ing in Publication Data Data available Johna 4 Striding arches, Moniaive 5 Blackfriars bridge 6 Arch bridge near Constantine 7 Chartres cathedral 8 Prague cathedral 9 Angkor Thom 10 Steel construction, NYC Kindly provided by Andrew Muir Wood 11 Buckling 12 Forth railway bridge 13 Structural action of Forth railway bridge 14 Sydney harbour bridge 15 Footbridge, Shirakawa 16 Nuclear power station, Torness 17 Footbridge, Aberfeldy 18 Airbus A380 Kindly provided by Andrew Muir Wood 19 Clywedog Dam 20 Taipei 101 21 Golden Gate bridge 22 Elements of civil engineering design process 23 Sydney Opera House 24 Bell Rock lighthouse Kindly provided by Andrew Muir Wood 25 Shinkansen 26 Millennium footbridge, London 27 tp://www.w3.or Introduction In 1828, the Royal Charter of the Institution of Civil Engineers, drafted by Thomas Tredgold, defined the purpose of the Institution: The general advancement of mechanical science, and more particularly for promoting the acquisition of that species of knowledge which constitutes the profession of a civil engineer; being the art of directing the great sources of power in nature for the use and convenience of man, as the means of production and of traffic in states, both for external and internal trade, as applied in the construction of roads, bridges, aqueducts, canals, river navigation, and docks, for internal intercourse and exchange; and in the construction of ports, harbours, moles, breakwaters, and light-houses, and in the art of navigation by artificial power, for the purposes of commerce; and in the construction and adaptation of machinery, and in the drainage of cities and towns. Setting off on a camping trip in the hills the associated needs are evident. You need shelter from the elements and from potential predators. You need a supply of fresh water, food, and energy for cooking, heating, and light. There is some advantage in thinking ahead about ‘waste disposal’ and possibly the need for medical supplies. Wandering the wilderness has its attraction but more frequently there will be some form of pathway in existence for you to follow. The pioneering people reaching the Americas or other unfamiliar lands had the same requirements. Interdependence encouraged the formation of settlements which became villages and then towns and cities. The benefits of scale and collaboration in provision of this infrastructure were obvious—common means of protection against predators (human or animal), common sources of clean water (no need for each household to have its own well), common strategies for disposal of waste (one man’s waste disposal can become another man’s pollution), common sources of energy, common networks of transport. The advantages of sharing skills and resources would have rapidly become apparent. All these elements of infrastructure come under Tredgold’s definition of ‘civil engineering’. The emergence of civil engineering The term civil engineering has been used, and its meaning has evolved for rather more than 200 years. Chambers Dictionary defines an engineer as ‘one who designs or makes, or puts to practical use, engines or machinery of any type, including electrical; one who designs or constructs public works, such as roads, railways, sewers, bridges, harbours, canals, etc; one who constructs or manages military fortifications, etc., or engines’. A civil engineer is ‘one who plans and builds railways, docks, etc. as opposed to a military engineer, or to a mechanical engineer, who makes machines etc.’ ‘Engineer’ comes from the Latin ingenium meaning skill, linked with the design and construction of clever devices (engines)—such as catapults—for inflicting damage in sources of power in nature which John in the military campaigns. The term civil engineering, introduced to distinguish those working with non-military engines, seems to be almost an oxymoron. But the term now relates to that branch of engineering which is concerned with the creation of the infrastructure of society: the civil implying this link with the citizen and with civilization. The techniques used for these civil projects have general application: civil engineers may well be working with the military on provision of buildings, roads, bridges, airfields—specifically un-civil engineering under the original meaning of the term. Of course, even before the term civil engineering was coined, civil engineers were in existence even if they were then described using other terms. The person (or persons) unknown who organized the construction of the Great Pyramids, some 5000 years ago, must be called an engineer. He (probably a man) certainly had acquired, by observation and experience, the skills necessary to translate a concept into reality using effective technology. The Greeks (Anthemius of Thrales and Isidorus of Miletus) put in charge of the design of the church of Hagia Sophia (in Constantinople – Byzantium or Istanbul) by the Roman Emperor Justinian in around AD 600 were, like Christopher Wren 1000 years later, expected both to solve the day-to-day problems of detailed construction and also to plan the overall shape and form: they were civil engineers. The Greek word μηχανη (mechani) means ‘a machine, or a clever trick’. Odysseus had the attribute πολνμηχανος (polimechanos): ‘one who knew many tricks and could cope with difficult situations’. This would be a good description of an engineer, but today a mechanic would be expected to have a narrower range of skills than an engineer. Anthemius and Isidoros would have been described as αρχιτκτονες (architektones): master builders. The potential for confusion of engineering and architecture is apparent. The inventiveness of cathedral builders such as Brunelleschi in devising special cranes for safely raising construction supplies would have qualified them for the description of engineer too. Words like mechanic, engineer, architect now resonate in a way which does not begin to think about their origins two or three millennia ago. Much mediaeval engineering was based on geometric rules based on prior experience of successful structures. Scientific understanding developed slowly as people such as Galileo sought explanations for phenomena and began to apply mathematical concepts to the analysis of structural elements. The Industrial Revolution which began in Britain in the early 18th century produced both a large number of ingenious steam-powered machines, which replaced man-power in the new factories, and also a new building material—cast iron—which had a strength greater than the timber that it replaced in many bridges and large buildings. With care in the manufacturing process, there was a predictability and narrow variation of the mechanical properties which made its use more straightforward. The cathedral builders (or engineers) organized themselves into a closed community, keeping the secrets of their experience within the community. The notion of engineering as a profession is a development from this but it occurred in different ways in different countries. The French, with an emphasisolution- neutral problem definition were their s on Cartesian analysis and mathematical representation, made rapid progress in what we now see as the various branches of engineering mechanics, with state encouragement. The British, with an emphasis on observation and practice, and less desire for central control, developed a more autonomous profession. The national routes to the formation of engineers differ widely in the balances of practice and theory or of training and education. In late 17th century France, the military engineer was given formal status by the creation of a Corps des Ingénieurs du Génie Militaire in 1690 by Maréchal Vauban. Vauban was famous for the design of fortifications, which usually consisted of masonry structures retaining compacted earth (within the remit of civil engineering today). In 1716, this was followed by the creation of the Corps des Ingénieurs des Ponts et Chaussées who were charged with the construction and maintenance of networks of communication (roads and bridges). The training of these latter engineers was formalized with the creation in 1747 of the École des Ponts et Chaussées, the first of the French Grandes Écoles: the concept of civil engineering as distinct from military engineering was already clear in France. The first director of the École des Ponts et Chaussées was Jean- Rodolphe Perronet who began his career with responsibility for the design of Paris sewers and roads – civil engineering infrastructure. In Britain, the Royal Society – founded in 1661 by Charles II – brought together individuals who shared a curiosity in observation of phenomena. Some took part in the search for scientific explanations of what they had seen. Christopher Wren and Robert Hooke were both members and worked together on the reconstruction of London after the fire of 1666. The boundary between what we might now see as science and engineering was rather fuzzy. We remember Wren as an architect and designer of buildings, Hooke more for his scientific insight (though Newton, who succeeded him as President of the Royal Society, did all he could to obliterate his memory). Both Wren and Hooke could equally be described as engineers. John Smeaton, born in 1724, was elected a fellow of the Royal Society in 1753, in recognition of his work on scientific instruments (which we would now categorize as mechanical engineering – he went on to study water and windmills). His ability to combine scientific interests with a skill in design resulted in his being recommended for the design of the Eddystone Lighthouse off the south-west coast of England. This success then developed into a major career in civil engineering, designing and supervising the construction of bridges, roads, and canals across England and Scotland. He was the first person to describe himself as a civil engineer, deliberately to distinguish himself from the military engineers, and was the natural person to create, in 1771, a Society of Engineers which met over dinner to share engineering problems and solutions. The membership of this rather informal group identified three classes of members: real engineers, actually employed as such; gentlemen of rank and fortune who have applied their minds to civil engineering; and various artists whose professions are useful to civil engineering: quite enlightened in its breadth. Smeaton’s Society of Engineers was the precursor of the Institution of Civil Engineers, which was founded in a coffee house on the Strand, in London, in January 1818 by three young engineers – Henry Palmer, James Jones, and Joshua Field. It did not have much influence as a professional engineering organization until Thomas Telford was elected sources of power in nature923 President in 1820. He was well known for his civil engineering prowess and had built a network of political and society contacts. He also knew most of those who were actively practising civil engineering in Britain. It was his drive which who were actively practising civil engineering in Britain. It was his drive which resulted in the award of the Royal Charter for the Institution in 1828, thus establishing the Institution as the visible professional body for engineers of all disciplines. (The Smeatonian Society of Civil Engineers remains in existence as an exclusive dining club and claims to be the oldest engineering society in the world.) The 19th century was a time of revolutions in Europe, a birth of new countries, and an upsurge of nationalism and self-confidence as industrialization and international commerce brought increased prosperity to nations and expansion to cities. It was inevitable that schisms should similarly develop in the newly established Institution of Civil Engineers. The Institution of Mechanical Engineers was created in 1847 (under the presidency of George Stephenson the railway engineer whose son and engineering partner Robert became President of the Institution of Civil Engineers in 1855). The (Royal) Institution of Naval Architects was founded in 1860 by (among others) John Scott Russell who had worked with Brunel on the Great Eastern (as partner or opponent, depending on which account you believe) and who was forced to resign his vice-presidency of the Institution of Civil Engineers because of his alleged involvement in some arms deals during the American Civil War. The Royal Aeronautical Society was founded in 1866. The Society of Telegraph Engineers (subsequently to become the Institution of Electrical Engineers) was founded in 1871. The Institution of Municipal Engineers was founded in 1874 with the encouragement of the Institution of Civil Engineers, with which they amalgamated in 1984. The Concrete Institute (subsequently the Institution of Structural Engineers) was founded in 1908. And so on … The sphere of influence of civil engineering was whittled away and although attempts have been made to merge some of these institutions in order to improve their national and international influence and to recognize the interdisciplinary nature of many of the most challenging engineering projects, the extent of consolidation in the UK has been somewhat limited. Some other countries have either avoided the schisms or have successfully reintegrated the profession. Today, the term ‘civil engineering’ distinguishes the engineering of the provision of infrastructure from these many other branches of engineering that have come into existence. It thus has a somewhat narrower scope now than it had in the 18th and early 19th centuries. There is a tendency to define it by exclusion: civil engineering is not mechanical engineering, not electrical engineering, not aeronautical engineering, not chemical engineering … But that is to lose sight of what is included. is to lose sight of what is included. In the 19th century, William Rankine was typical of those engineers/scientists who had a broad interest in phenomena and the theory to describe them. He is known today both for his developments in thermodynamics and the underpinning theory of heat engines, and for key theoretical analysis of the pressure exerted on earth retaining structures – enabling the design of walls supporting slopes or terraces, for example. Was he a civil engineer? Yes, of course. Was he a mechanical engineer? Well, yes, but the concept did not really exist until the middle of the century. Similarly Charles Coulomb is known by schoolchildren for his work on electric charge but is also well known to students of civil engineering for his theoretical work on the pressure of earth on retaining structures. Was he a civil engineer? His work on earth pressures was performed while he was in the French army in Martinique and designing defensive e supply of fresh water is onet experiencearthworks as part of the fortifications. So by strict definition he was not a civil engineer although the work for which he was responsible would today be seen as falling within the remit of a civil engineer. His work on electrostatics also makes him an electrical engineer. As a footnote to this discussion of the nature and definition of civil engineering, it is interesting to note that in some countries – for example, Norway and Sweden – the term civil engineer has retained something of its earlier broad meaning as an indicator of a level of educational attainment in an engineering field. One might be a civil engineer with qualification in electrical engineering or in information technology, or in chemical engineering – or in building engineering or in road and water construction. There is always room for diversity and confusion. Civil engineering today is seen as encompassing much of the infrastructure of modern society provided it does not move – roads, buildings, dams, tunnels, drains, airports (but not aeroplanes or air traffic control), railways (but not railway engines or signalling), power stations (but not turbines). The fuzzy definition of civil engineering as the engineering of infrastructure, the lubrication of society, should make us recognize that there are no precise boundaries and that any practising engineer is likely to have to communicate across whatever boundaries appear to have been created. This is a challenge for education to ensure that the languages of boundary disciplines are not so disparate that communication is prevented. The boundary with science is also fuzzy. Engineering is concerned with the solution of problems now, and cannot necessarily wait for the underlying science to catch up. But as scientific understanding of the bases for engineering decisions improves, so also does the confidence level attached to those decisions. All engineering is concerned with finding solutions to problems for which there is rarely a single answer. Presented with an appropriate ‘solution-neutral problem definition’ the engineer needs to find ways of applying existing or emergent technologies to the solution of the problem. There are a number of distinctive features which add excitement to the practice of civil engineering: civil engineering projects tend to be large, they tend to be visible, and they tend to be unique. At every stage through selection of structural form, choice of materials, to final appearance, decisions have to be made. There is a subjectivity, of course, but also an immense feeling of pride in having been closely involved with the decision making process which led to the construction of that bridge, that tunnel, that building. People are thus an important element of civil engineering projects. Though there may be possibilities for mass production of structural elements such as beams and columns or even sections of bridge decks, the structures into which they are incorporated will usually be quite different. The corollary is that, like the coke-can ring-pull, the project has got to work first time. The size and visibility mean that failures cannot be hidden. The uniqueness of most civil engineering projects means that, while theory and analysis certainly have their place, direct experience or experience transmitted by older engineers remains important: ‘good judgement comes from experience, experience comes from bad judgement’. The dramatic increase in the power and availability of computers over the past 50–60 years has opened up possibilities for (approximate) numerical solutions to engineering problems which could not have been contemplated previously. Those computer analyses require as input the output of to resist thest their scientific studies of material response: science and engineering proceed hand in hand. Decisions formerly made on the basis of experience (‘would a structure built with these proportions be likely to stand up?’) can now be supported by analysis. However, it is too easy to be taken in by the first colourful outpourings of a computer program without confirming, by simple back-of-the-envelope calculation, drawing on experience or simplified modelling, that the answers are at least of the correct order of magnitude. Architecture and civil engineering Inevitably many of the example projects that will be described here will be structures – for example, buildings and bridges. These are among the more structures – for example, buildings and bridges. These are among the more visible creations of civil engineers, but we tend to associate architects rather than engineers with such structures – though originally an architect was simply a ‘master builder’ (or engineer). The distinctive separation of the professions has occurred gradually over the past few centuries. Sir Christopher Wren is remembered as an architect of the second half of the 17th century, yet in his activities in designing and supervising the construction of St Paul’s Cathedral after the Great Fire of London he was as much an engineer (in our 21st century interpretation of the term) as an architect. The distinction did not then exist in Britain. Projects such as the creation of channels to supply water to growing conurbations required understanding of the mechanics of pumps and mills and steam engines. The emphasis of the projects fell firmly onto engineers (to handle all the engines) and surveyors, and the opportunity for involvement of architects (as we understand the term) was limited. In early 18th century France, the Corps du Génie was concerned with the (civil) engineering of defence fortifications for withstanding the siege of towns. The Corps des Ponts et Chaussées was responsible for the (civil) engineering of roads, canals, water supply. A third institution, the Bâtiments du Roi, was linked with aesthetics of engineered buildings as required by the king. In America, the Corps of Engineers, though primarily concerned with matters of defence and fortification in time of war, manoeuvred itself into a powerful position, reclaiming land from mudflats on the Potomac river and developing the new capital city of Washington DC. The introduction of iron as a construction material after the Industrial Revolution produced a breed of fabricator/contractor, developing skills onto a larger scale from the work of the pre-existing blacksmith. In dealings with architects they had a clear notion of the capabilities and limitations of their material. Gustave Eiffel was primarily an enterprising contractor but had sufficient numeracy and design skills to be thought of now as an engineer and designer. The rapidly increasing extent of scientific knowledge could be applied to the analysis of structural elements and systems (and the ground). Enlightened programmes of education tried to maintain a breadth which kept engineering and architecture together. However, increasing investment in roads and railways and building (and other elements of infrastructure) led to increasing demands for specialist engineering designers. Andrew Saint suggests that a ‘broadbrush way of describing the change in relations between architects and engineers over the past two centuries might be to say that they used to work on different projects but have similar skills, whereas they now work on the same projects but have different skills,’ and also, ‘Architecture lacks the linearity of engineering, that quality of conConcept – technology – realizationthMsistent serviceability and of the creative skill subordinating itself to the practical end at issue … It is architecture’s freedom from the manipulations of utility that makes it a true art’. Many of the attempts to separate engineering and architecture seem to be based on rhetoric rather than on the actual process of creating structures. ‘Once we enter into what happens when a structure is actually assembled in any age, we find designing and making, architecture and engineering, art and science muddled up together so constantly and utterly that a once-and-for-all process of dissociation in an age of reason or enhanced technology appears implausible.’ Ars sine scientia nihil est and scientia sine ars nihil est: partnership and recognition and appreciation of complementary skills are in the interests of all construction professionals. US President Harry Truman said in 1948, ‘You can achieve anything so long as you don’t mind who takes the credit.’ Pride in a quality product should outweigh personal recognition. However, it is galling when the credit is given to a quite different professional group. The media often credit scientists with having done something which is clearly the result of engineering input, and architects (we may feel) receive more than their share of credit for civil and structural engineering projects. Most projects are in fact partnerships between a number of pUBLIC "-//W3C/ Chapter 1 Materials of civil engineering In searching for economical and efficient solutions to the problems that have been posed, engineers seek effective exploitation of the mechanical properties of the materials that are being used: properties such as the stretchiness or stiffness (the change in dimensions as the material is subjected to changes in load) and the strength (the limit to the amount of load that can be supported). Through experience and understanding, engineers have become more adept at exploiting these properties in order to produce increasingly daring structures. Effective exploitation implies that civil engineers are able either to control these properties to suit the application or to understand the nature of the properties of the materials with which they are presented. For example, the steel and concrete that we see in structures above the ground can be designed to have chosen stiffness and strength. These are manufactured materials which may have to be transported a long way to reach a construction site. The costs of transportation may be regarded as excessive, and locally sourced wood and rock may be available instead – their strength and stiffness are as you find them. They will be expected to ensek. Rock is just one constituent of the more general range of materials that make up the ground. The softer materials are designated as soils though the boundary between weak rocks and hard soils is not well defined. Soil All civil engineering constructions sit upon or sit in the ground. Tunnels pass through the ground; foundations for buildings and bridges are excavated from the ground; aeroplanes land on the ground; motor cars and railway trains drive on roads or rails laid on the ground; and ships berth against structures which are attached to the ground. So the behaviour of the soil or other materials that make up the ground in its natural state is rather important to engineers. However, although it can be guessed from exploratory probings and from knowledge of the local geological history, the exact nature of the ground can never be discovered before construction begins. By contrast, road embankments are formed of carefully prepared soils; and water-retaining dams may also be constructed from selected soils and rocks – these can be seen as ‘designer soils’. Today tourists flock to Pisa (Figure 1) not because of the fine Romanesque duomo but because of the unfortunate incident of the belfry (which has resulted in the area around the duomo being called the campo dei miracoli) – they come to see the leaning tower. Like the duomo, the tower is an example of Romanesque construction with semicircular arches round the lower storey and semicircular arcades of arches around each storey of the tower. The few window openings on the staircase which spirals round inside the wall are small. The bells are hung within the top storey. What went wrong? Soils are formed of mineral particles packed together with surrounding voids – the particles can never pack perfectly. The mineral particles come from the erosion of rock: sands are formed of particles of quartz or feldspar formed by mechanical erosion; clay minerals are silicates and aluminates resulting from weathering of parent rocks under conditions of high temperature and humidity. It may be surprising that granite, which is naturally a very strong structural material, weathers to form the clay mineral kaolinite, used for making porcelain. Porcelain, after it has been formed and baked at high temperature, is once again very strong and brittle. 1. The tower of Pisa would not attract tourists if the foundation conditions had been more uniform. Recent removal of small quantities of clay from the foundation has stabilized the tilt Clay particles are small – officially smaller than 2 microns (2 millionths of a metre) in size – but soils can contain a very wide range of particle sizes depending on the source material, the manner of the weathering process, and the nature of the transportation process to the present location. Fine particles can be carried by wind; coarser particles can be carried by water; ice in the form of glaciers slowly moving down hillsides can transport huge boulders which may be subsequently discovered when the ground conditions at a site chosen for construction are being explored. The voids around the soil particles are filled with either air or water or a mixture of the two. In northern climes the ground is saturated with water for much of the time. For deformation of the soil to occur, any change in volume must be accompanied by movement of water through and out of the voids. Clay supply of fresh water is oneanMsen particles are small, the surrounding voids are small, and movement of water through these voids is slow – the permeability is said to be low. If a new load, such as a bridge deck or a tall building, is to be constructed, the ground will want to react to the new loads. A clayey soil will be unable to react instantly because of the low permeability and, as a result, there will be delayed deformations as the water is squeezed out of the clay ground and the clay slowly consolidates. The consolidation of a thick clay layer may take centuries to approach completion. The site chosen for the construction of the belfry at Pisa, begun in 1173, provided a rather poor foundation. The irregular layering of two different clays gave rise to a variable time-dependent deformation under the foundation of the tower with the result that it started to tilt only a few years after construction had begun. The 12th and 13th centuries were unsettled times in Italy with the different city states of Pisa, Genoa, Lucca, and Florence all vying for dominance. Battles demanded money and people, and so the construction of the belfry took second place. When the masons under the direction of Giovanni di Simone resumed construction in 1272 they introduced a curve in the vertical alignment of the tower so that the courses of stone were once again being laid horizontally. This stratagem proved inadequate and the tower continued, slowly, to tilt further. Construction stopped again in 1284 and the belfry was only completed in 1372. The top storey very obviously makes another valiant attempt to establish a properly vertical line. A leaning tower may be a valuable draw for tourists but as the tilt increases, the tower becomes progressively less stable. The tilted loading tries to encourage additional tilt of the foundation. There are many leaning towers in Italy: the skyline of Venice contains an impressive collection. There were once more. The brick-built campanile of San Marco in Venice collapsed in 1902 and was rebuilt. In 1989 the civic tower in Pavia collapsed without warning, killing three people. It was leaning but not dramatically: failure may have occurred because of variable movements in the cement mortar in which its bricks were set or because of slow, time-dependent changes in the properties of the bricks themselves rather than because of foundation problems. But the failure of one leaning tower naturally raised concerns about the safety of another one. naturally raised concerns about the safety of another one. In 1990, careful monitoring of the Pisa campanile showed signs that the tilt of the tower was accelerating and there were signs of distress in stonework on the down-tilt side. Of the possible options, the only realistic one was to make some intervention in order to safeguard the tower in its current tilted position. Tampering with the foundation of a structure which is on the brink of collapse carries the risk that it will in fact trigger that collapse. This daring decision was taken by an international committee of geotechnical engineers (civil engineers with a particular interest in the behaviour of the ground). Modest safety measures were installed in the form of lead weights on the up-tilt side of the foundation together with cables which could have provided some slight restraint if the tilt had started to accelerate. Then small chunks of clay were extracted from under the up-tilt side of the foundation until the tower had rotated backwards to the inclination which it had had in about 1838. Its lifetime was thus successfully extended. Timber At Pisa and other cathedrals timber was used to construct temporary access platforms and supporting structures to enable the masonry building to be completed. Timber provides the internal structure for the sources of power in natureH experience conventional roof that is seen from outside the duomo. Provided there are trees around, timber has the obvious advantage over stone of being easier to work with. It obviously has the disadvantage of being combustible. Few timber buildings remain from the Middle Ages or earlier and, where they do, there is the constant risk of destruction. The Great Fire of London of 1666 started in a baker’s shop and destroyed the old St Paul’s Cathedral and some 13,000 houses. Nearly an entire block of the city of Trondheim, Norway, was destroyed in December 2002 by a fire which started in the kitchen of a restaurant. Also, timber has a tendency to rot if it becomes permanently damp and is eaten by bugs of various sorts and has a tendency to warp as it seasons and takes up or loses moisture. On the other hand, trees grow in a way which is structurally efficient. The root systems respond to the external loadings applied by the wind in order to anchor a tree to the ground, whereas the branches are sufficiently flexible to move under wind and other environmental loadings. The cross section of a tree reduces from the roots upwards and outwards as the forces required to sustain or resist the movements of the more distant parts of the tree themselves become smaller. Wood can support compression loads very effectively and can support tensile loads, provided they are applied parallel to the grain (along the branches). Inspection of the wounds left by a branch or a trunk splitting will reveal the very fibrous nature of the growth and hint at the way in which these fibres might be strong individually but become readily delaminated if the applied loads try to pull them apart. The shape of a tree – a trunk with a single branch – can be deliberately exploited to provide a single curved cruck for a house or barn. 2. The great south gate (Nandaimon) of the Todaiji temple complex in Nara, Japan is typical of the use of ‘trabeated’ timber construction – beams and columns The great south gate of the Todaiji temple complex in Nara, Japan, (Figure 2) was built in the 12th century at about the same time as many of the great Gothic cathedrals of Europe. The decoration is elaborate and serves to conceal some of the structural interconnections, with horizontal tie beams slotted through the main supporting vertical posts (a trabeated style of building). The detail of the substructures devised to permit the eaves of the roof to overhang as far as possible and to enable a high roof ridge, apparently two storeys up, to be formed over a single storey internal space shows great ingenuity. This was the product of cultural exchanges between Buddhists in Japan and China led by the priest Shunjobo Chogen who brought Chinese craftsmen to work alongside Japanese carpenters. Japan is, of course, a country of frequent earthquakes: timber structures such as these old temple buildings have enough flexibility in their joints to be able to withstand significant horizontal shaking without damage. Stone ‘Hardwick Hall – more glass than wall’ was built by Robert Smythson for Bess of Hardwick in the 16th century, on a hilltop, as a deliberate statement of wealth and power. The individual panes of glass were small and irregular, the source of that dappled charm of old buildings, but the internal result was of great light. The Romanesque cathedrals such as Pisa give a feeling of solidity and security. The columns in the nav6">A Very Short Introductioncolour rather than light since the enormous windows are usually filled with magnificent mediaeval stained glass). Rock (or stone) is a good construction material. Evidently there are different types of rock with different strengths and different abilities to resist the decay that is encouraged by sunshine, moisture, and frost, but rocks are generally strong, dimensionally stable materials: they do not shrink or twist with time. We might measure the strength of a type of rock in terms of the height of a column of that rock that will just cause the lowest layer of the rock to crush: on such a scale sandstone would have a strength of about 2 kilometres, good limestone about 4 kilometres. A solid pyramid 150 m high uses quite a small proportion of this available strength. If the rock can be easily cut then it can readily be used for the carving of shapes and figures (but is also more likely to weather and decay). The builders of the Egyptian pyramids had the task of creating a massive rock structure around a small burial chamber. The small hole created local stress concentrations which they could relieve by leaning slabs of stronger stone against each other to form an internal pitched roof. Or alternatively they could create an internal protection by placing blocks of stone one on top of the other on each side of the void, projecting out (corbelling – see Figure 3) over the void gradually further and further until the void was closed by a stepped roof – much as one would if trying to create a bridge with children’s bricks to span a gap wider than the longest brick. 3. The builders of the pyramids in Egypt around 2600 BC used corbelling to bridge over internal burial chambers The Romans developed the semicircular arch as a structural form. An arch formed of a series of shaped voussoirs (carefully prepared wedge-shaped stones) is extremely strong once it is complete, provided it is able to push sideways against unyielding abutments (Figure 4). During construction the incomplete arch has to be supported using appropriate centring, usually made of wood (Figure 5). A little mortar on the radial joints between the voussoirs can provide some extra strength, but is not essential if the stones are carefully prepared. The centring is typically removed by pulling out carefully placed timber wedges. There is usually a little inevitable movement of the masonry as it takes up the load. 4. An arch converts vertical loads to a horizontal abutment thrust. This is one of Andy Goldsworthy’s ‘striding arches’ near Moniaive A Very Short IntroductionWhen the centring for the Pont de Neuilly over the Seine just west of Paris was going to be removed in 1772, the engineer, Perronet, made sure that the centring for each of the five spans was removed simultaneously with a great splash in order to distract attention from the movement of the bridge which the crowds of spectators (including Louis XV) might otherwise have found a little disturbing. If the abutments move outwards a little, as they usually will, then the arch will spread a little and cracks open between some of the voussoirs – see Figure 6. This is not necessarily a problem provided the loads applied to the arch (which might form the main load carrying element of a bridge or aqueduct) can find a route through the contact points of the voussoirs in whatever slightly rotated positions they now find themselves. Careful study of masonry structures, old and new, will usually reveal some modest (harmless) cracking from which the mechanism of displacement of the masonry can be deduced. 5. Until the arch is complete it requires temporary support in the form of timber ‘centring’, shown here for Blackfriars Bridge under construction across the Thames (it was opened in 1769) A continuous barrel vault over a space such as a hall, a wine cellar, or the nave of a church, is just a series of arches in a row, all pushing sideways uniformly and with solid supporting side walls resisting this push. However, if these side walls consist mostly of space (windows) then some other means of supporting the vertical load (the weight of the vault and the roof) and withstanding the sideways push has to be devised. So these forces have to be concentrated into individual columns between the windows. With everything being done to emphasize height, the columns supporting the vault to the nave of a church have to be slender. There is no difficulty in the columns carrying the vertical load from the vault and roof. Even in a cathedral like Chartres, near Paris, where the vault is 37 metres above the floor of the nave, the margin of safety appears to be large compared with the strength of the rock, which is of the order of a few kilometres. As we look up into the vault we can see the ribs channelling the loads into the columns. Unlike a bridge, there are no earth abutments at the top of the columns so a stone abutment-substitute has to be created. The horizontal push from the vault, and the loading from winds, can be absorbed by using elegant flying buttresses (Figure 7), which carry the load over the side aisles to lower external buttresses. Pinnacles on top of these buttresses push the load more effectively into the masonry. 6. Many arches are able to carry loads even when cracks open between rotated voussoirs, as in this bridge near Constantine (Algeria) For most Gothic buildings the general scheme or concept would be defined by the dean and chapter of the cathedral but they, having no expertise in construction, would expect the master mason (engineer) with his team of masons and labourers to devise the structural (and decorative) detail and to work out how to build it. Such teams were peripatetic, moving from one construction site to the next as money ran out or became available. They would learn new ideas for the next project and there would, e6">A Very Short Introduction 7. The arched vault of a Gothic cathedral such as Chartres requires support in the form of flying buttresses which carry the lateral loads (and wind load) over the aisles Timber temporary structures enabled the masons to gain access to the working levels of the church and to provide support centring for the stone of the vaults and arches as they were being assembled. It would not have been practicable to erect scaffolding from the floor to the vault, and very difficult to arrange the safe removal of the centring to allow the vaults to take up their loads. Large churches are riddled with corner stairs and passages hidden in the larger columns and walls, giving access to temporary working platforms. The centring would have been strutted from projections and holes deliberately left in the walls below. Cranes (engines) driven by man-powered wheels (like large versions of hamster wheels, putting the energy to useful purpose) were used to lift blocks of stone. While the builders of the Gothic cathedrals were becoming ever more daring (Figure 8) with their exploration of the possibilities of the stone arch, the Khmer builders of the temples of Angkor in Cambodia (Figure 9) were still, in the 13th century after five or six centuries of building, sticking firmly to a trabeated form of construction using horizontal lintels and vertical supporting posts. They had not been told about the arch and could only bridge spaces with corbelled structures as used over the inner chambers within the pyramids several thousand years earlier. 8. The later Gothic flying buttresses at Prague reflect the increasing confidence and daring of the masons in their use of stone as a construction material 9. The temple builders in Angkor Thom, Cambodia, were still using corbelling to create archways in AD 1200 The knowledge possessed by the European masons (engineers) was kept within the group as far as possible: they had to train their successors but had also to give the impression that their largely empirical knowledge, based on geometrical rules whose prior success had been observed, was too complex for the layman, or amateur, or ecclesiastical client to grasp. Where failures occurred they were usually geometrical:solution-neutral problem definitioni10 extremely slender and tall columns became susceptible to instability through buckling if there was some slight change in alignment as a result of foundation settlement. The emergence of theoretical ideas which might support their designs (or might not) was regarded as a threat in somehow removing this aura of secrecy and skill and potentially opening their profession to anybody of mathematical education. Iron and steel Iron has been used for several millennia for elements such as bars and chain links which might be used in conjunction with other structural materials, links which might be used in conjunction with other structural materials, particularly stone. Stone is very strong when compressed, or pushed, but not so strong in tension: when it is pulled cracks may open up. The provision of iron links between adjacent stone blocks can help to provide some tensile strength. The concept was straightforward but the technology was challenging. The Industrial Revolution, in the 18th century, resulted in the development of techniques for the reliable production of iron (and later steel), which were then put to use in the creation of more exciting structures than could be produced with masonry alone. Cast iron can be formed into many different shapes and is resistant to rust but is brittle – when it breaks it loses all its strength very suddenly. Wrought iron, a mixture of iron with a low proportion of carbon, is more ductile – it can be stretched without losing all its strength – and can be beaten or rolled (wrought) into simple shapes. Steel is a mixture of iron with a higher proportion of carbon than wrought iron and with other elements (such as manganese or titanium or chromium) which provide particular mechanical benefits. Mild steel has a remarkable ductility – a tolerance of being stretched – which results from its chemical composition and which allows it to be rolled into sheets or extruded into chosen shapes without losing its strength and stiffness. There are limits on the ratio of the quantities of carbon and other elements to that of the iron itself in order to maintain these desirable properties for the mixture. Its development was thus associated with both the provision of close control of the chemical content in the blast furnaces (then the Bessemer converter, and latterly electric arc furnaces) and the construction of powerful rolling mills. 10. Site safety rules would today frown upon the antics of these workmen assembling the steel frame of a skyscraper in New York. However, the I cross-section of the beams and columns is apparent. The web carries the transverse load and the flanges resist bending – and improve resistance to buckling There are many iconic photographs of the high-riggers on skyscrapers in New York, showing a disregard for safety precautions that would not be accepted today (Figure 10). If you look at a steel-framed building while it is being constructed you will see that most of the steel elements, vertical or horizontal, have an H section consisting of two parallel plates (flanges) connected by another plate (web). Steel is very strong and stiff in tension or pulling: steel wire and steel cables are obviously very well suited for hanging loads. If you push down on a long thin strip it will not remain straight but will buckle sideways, undergoing geometrical failure. If you are lucky, it will return to its original shape when you stop squashing it. This is the geometrical failure towards for many years as. There which the increasingly slender and tall stone columns of Gothic cathedrals were heading. The buckling of a thin steel section might seem to indicate that the material has a low stiffness when it is compressed. This geometric loss of stiffness can be improved in two ways. If we hold the centre of the thin strip to stop it moving sideways and then push down we will find that the load at which the buckling instability occurs is about four times larger than for the unrestrained strip (Figure 11). The mode of buckling now has two bows, one each side of the central restraint. So, shortening the unsupported length is one way of improving the ability of a structural member to support compression. The other way is to make it harder for the structural member to bow. A thin plate on its own has very little bending resistance. If the plate is incorporated into an H section then the bending becomes more difficult. However, even an H section steel girder will be much stronger when being pulled than when being compressed. In compression, buckling will eventually occur. The propensity to buckle thus depends on the cross-section of the girder and also on its length. The design of the river crossing of the Firth of Forth (Figure 12), heading north from Edinburgh, was subjected to great scrutiny and conservatism of design because of the failure, in 1879, of the railway bridge across the River Tay – the next estuary to the north. The designer for the Forth Bridge Company, which was providing a vital link between sections of the railway network of the North British Railway Company, was Sir Benjamin Baker with Sir John Fowler. The result was the iconic structure, completed in 1890, somehow typifying Victorian engineering, and it is still in regular use for all railway traffic heading from Edinburgh to Dundee and Aberdeen. Such icons are not necessarily things of beauty but this one shows very well how it carries the loads (Figure 13), with strongly braced three-dimensional frames sitting over each pier separated by lighter structural sections which balance between the strong frames. It was the first major structure in Britain to be constructed entirely from steel (the contemporary Eiffel Tower in Paris was constructed of wrought iron). A similar structural arrangement was used for the Canadian National Railway bridge across the St Lawrence river, upstream of Quebec, completed in 1919. 11. (Left) A thin strip, loaded axially, develops a geometrical failure called buckling. (Right) If the strip is prevented from moving sideways at its midpoint then the load it can carry before it buckles is increased fourfold 12. The Forth Railway Bridge, near Edinburgh, was designed to resist much higher wind loading than the Tay bridge, some 50 km further north, which collapsed in a storm in 1879 13. The topmost members of the main truss of the Forth Railway Bridge (dotted) act in tension (pulling); the vertical and lower members of the truss (solid) act in compression Forth railway bridge Masonry (stone) arches have been recognized for more than two millennia as providing a strong way of bridging a gap in order to support loads of people or traffic. The arch converts the vertical forces provided by the steady or varying loads into horizontal forces at the abutments, each side of the gap. The abutments need to be sufficiently strong to be able to withstand this horizontal push. The shape of the arch has to be chosen to ensure that the masonry is in compression all round the arch. Masonry is heavy and may not be readily available locally. Steel provides a much lighter structural material. An arch can be formed out of a steel lattice with the cross-bracing ensuring that the unrestrained lengths of the compression elements are short enough to eliminate the possibility of buckling. The Sydney Harbour Bridge (Figure 14), which was completed in 1932 with a main span of 503 m, is just such a steel arch bridge, but with the deck running through the arch at half height. It is very similar in appearance and structural form to the less well known Hell Gate Bridge in New York, a railway bridge 310 m long, designed by Gustav Lindenthal and completed in 1916. The deck is partly hung from and partly supported on (towards the ends of the main span) the steel arch which is terminated with a masonry tower at each end which serves the same structural purpose as a pinnacle over the outer wall of a Gothic cathedral, where it receives the force from a flying buttress. Its weight helps to push the resultant abutment force from the arch more directly downwards into the foundation. Aesthetically the towers give a reassuring sense of solidity to the bridge. The upper steel members, which also seem to form an arch, do not actually reach the masonry towers. They provide stiffening and lateral support to the arch itself in order to prevent it from buckling. 14. The deck of the Sydney Harbour Bridge is carried on a steel arch with plenty of vertical and diagonal bracing to ensure that the arch will not buckle Concrete Concrete is a sort of artificial rock (conglomerate) which can be cast to almost any chosen shape and size. The Romans discovered that mixing pozzolana – a volcanic ash product found in the region around Vesuvius – with lime and water produced a reaction which, when complete, left a very strong rock-like material. The dome of the Pantheon in Rome was built in around AD 126 using this early concrete. A large quantity of massed concrete around the lower levels of the dome was used to absorb the horizontal thrusts. The Pantheon is intended to be admired from within (the coffered cut-outs from the underside of the dome help to reduce its weight) rather than from without. It remained the dome with the largest span in the world until the dome of Santa Maria del Fiore in Florence was completed. For some applications, all that is required is the mass of the concrete, and its necessary mechanical properties are equivalent to those of an artificial rock. For example, with appropriate ground conditions, concrete gravity dams provide a straightforward means of blocking a valley and retaining a reservoir. The Colorado River runs from the Rockies in Colorado and Wyoming through Utah, Arizona, and Nevad { text-align: justify; margin-top: 1em; margin-bottom: 1em; text-indent: 0.01em; margin-left: as. There a to the Mexican border and the Gulf of California. Its name comes from the colour of the sediments that it carries down to the sea. But for river water to reach the sea (in another country) seems a waste when it could be used to support growing city populations. The Hoover Dam (originally called the Boulder Dam, from the canyon that it closes) approved by Congress in 1928, together with the 400 km aqueduct to Los Angeles, was completed in 1935, providing a major source of construction employment during the Depression years. Congressional approval was notionally dependent on the completion of an agreement between at least six of the seven states which had an interest in the Colorado water. But the agreed water distribution was based on flow rates which were greatly overoptimistic. The dam retains a water depth of about 180 m and retains a reservoir which is some 180 km long with a volume of about 35 cubic kilometres. It regulates the flow in the downstream river to allow abstraction for the aqueduct but also relies on this flow through its turbines to generate 2.1 GW of electricity, of which a sizeable proportion is required to power the five pumping stations that take the water over the hills to the Californian coast. A dam is just a big plug in a valley. This plug can be made in many different ways. Childhood experience of damming streams shows that compacted earth will restrict the flow and allow a (modest) lake to form. Including some stones or small rocks helps to give some extra solidity. But water is quite good at finding chinks – through or under or round the sides of the quite good at finding chinks – through or under or round the sides of the dam. Even if these holes are blocked, when the lake fills to the top of the dam the overflowing water will erode weaker elements of the downstream face. Few of those youthful constructions survive for long. The same problems face the designer of a real dam. Initially, small dams across the valley divert the river into pipes or tunnels so that the subsequent serious construction work can proceed in the dry. At the Hoover Dam parts of these diversion tunnels were incorporated into the eventual spillway system allowing controlled flow of water into the downstream river and also a safe means of discharge, without erosion of the dam, when the reservoir is full. The pressure exerted by 180 m of water is high and has somehow to be transmitted to the base and sides of the gorge in which the dam is to be built. An arch is an effective way of transmitting vertical loads from bridge traffic to the horizontally resistant abutments. An arch dam performs the same mechanical process but turned through 90 degrees. The Hoover Dam is a combined gravity arch concrete dam. The convex arched upstream section in plan takes the water pressure to the sides of the gorge. The descriptor gravity refers to the sheer bulk of the dam sitting on the floor of the gorge and resisting downstream movement. The dam is 200 m thick at its base and 14 m thick at the crest, which is 380 m long, and it has a volume of 2.5 million cubic metres. As concrete sets, the chemical reactions that turn a sloppy mixture of cement and water and stones into a rock-like solid produce a lot of heat. If a large volume of concrete is poured without any special precautions then, as it cools down, having solidified, it will shrink and crack. The Hoover Dam was built as a series of separate concrete columns of limited dimension through which pipes carrying cooling water were passed in order to control the temperature rise. Evidently the junctions between the blocks needed to be sealed and the cooling pipes to be filled, and the rock beneath and beside the dam to be rendered impermeable. Grout is a fine mixture of cement, water, and sand which can be pumped into gaps and fissures. Holes drilled in the valley floor were used to inject a grout curtainsolution-neutral problem definitioni10 in order to seal the rock to a depth sufficient to limit any leakage from the full reservoir. Similarly, having understood the nature of the geological structures present in the sides of the valley, these rocks were grouted both to prevent flow of water and also to strengthen them to resist the abutment forces from the dam. The importance of understanding the geology of a site for the construction of a dam was emphasized by the failure in 1959 of the Malpasset Dam in south-east France. This was a double curvature mass concrete arch dam – curved both horizontally (like Hoover) but also vertically. It was the thinnest arch dam ever built – 7 m thick at the base and 1.5 m thick at the crest – and about 60 m high and 190 m between abutments. Completion in 1954 was followed by a series of dry years (and a problem with a recalcitrant landowner) and it was not until the extremely heavy rains in late 1959 that the reservoir filled very rapidly. The dam failed on the evening of 2 December killing 423 people downstream. Protracted investigations revealed weak seams in the rock of the left abutment which had displaced slightly under the rapidly rising water load. This displacement was enough to destroy the elegant structure. Reinforced and prestressed concrete Concrete is mixed as a heavy fluid with no strength until it starts to set. Embedding bars of a material such as steel, which is strong in tension, in the fluid concrete gives some tensile strength. Reinforced concrete is used today for huge amounts of construction throughout the world. When the amount of steel present in the concrete is substantial, additives are used to encourage the fresh concrete to flow through intricate spaces and form a good bond with the steel. For the steel to start to resist tensile loads it has to stretch a little; if the concrete around the steel also stretches it may crack. The concrete has little reliable tensile strength and is intended to protect the steel. The concrete can be used more efficiently if the steel reinforcement, in the form of cables or rods, is tensioned, either before the concrete has set or after the concrete has set but before it starts to carry its eventual live loads. The concrete is forced into compression by the stretched steel. The working loads that the concrete has to support would have been expected to generate tension in the concrete. As a result of the prestressing, these loads are carried by reducing this initial compression of the concrete. Such prestressed concrete gives amazing possibilities for very slender and daring structures (Figure 15). The analysis of such structures provides its own challenges: the concrete must be able to withstand the tension in the steel, whether or not the full working loads are being applied. For an arch bridge made from prestressed concrete, the prestress from the steel cables tries to lift up the concrete and reduce the span whereas the traffic loads on the bridge are trying to push it down and increase the span. The location and amount of the prestress has to be chosen to provide the optimum use of the available strength under all possible load combinations. The pressure vessels used to contain the central reactor of a nuclear power station provide a typical example of the application of prestressed concrete. 15. Prestressed concrete provides possibilities for extremely slender structures such as this ribbon footbridge at Shirakawa in Japan A Very Short IntroductionAll nuclear power generation so far relies on the principle of nuclear fission: when bombarded with neutrons, uranium 235 decays into other elements (such as krypton and barium) with the release of several neutrons. These neutrons are available to bombard other uranium atoms to encourage further degradation, each time with the release of energy. At the centre of a nuclear power station is a reactor in which this process occurs in a controlled fashion. Control is provided by a combination of nuclear moderator and control rods made of material which is ‘poisonous’ to neutrons and thus can absorb them or slow them down so that their thermal energy can be extracted. Graphite is a good moderator, and the cores of nuclear reactors are often formed of carefully engineered graphite blocks. The coolant fluid used to extract heat from the core can also have moderating properties. The nuclear power station at Torness in south-east Scotland (Figure 16), using advanced gas-cooled reactors, was among the last of this type to be built in the UK at the end of a programme of nuclear investment which began in the 1950s. It has a generating capacity of 1.36 GW and came on power in 1988 after an 8 year construction period. Its operating life is presently destined to end in 2023. The two reactors use carbon dioxide at 40 times atmospheric pressure as the coolant of the radioactive core. Water is pumped through a heat exchanger in the reactor where it absorbs heat from the circulating carbon dioxide and is turned to steam at a temperature similar to that used in typical coal-fired power stations. The steam expands through the turbines and in generating power loses pressure and partially condenses to water. The condensation process is completed in a cooling loop, and the resulting liquid is ready to start the cycle once more. The condensation process requires cooling water, and typically power stations are located by a convenient river or other source of water – Torness is on the coast. There are many civil engineering contributions required in the several elements of the power station – and certainly many interactions with specialists from other branches of engineering. The electricity generation side of a nuclear power station is subject to exactly the same design constraints as any other power station. Pipework leading the steam and water through the plant has to be able to cope with severe temperature variations, rotating machinery requires foundations which not only have to be precisely aligned but also have to be able to tolerate the high frequency vibrations arising from the rotations. Residual small out-of- balance forces, transmitted to the foundation continuously over long periods, could degrade the stiffness of the ground. Every system has its resonant frequency at which applied cyclic loads will tend to be amplified, possibly uncontrollably, unless prevented by the damping properties of the foundation materials. Even if the rotating machinery is being operated well away from any resonant frequency under normal conditions, there will be start-up periods in which the frequency sweeps up from stationery, zero frequency, and so an undesirable resonance may be triggered on the way – just as there is often a slightly alarming stage as a washing machine moves into the spin stage. 16. The grey cuboids of the power station at Torness, Scotland, give no clue of the prestressed concrete nuclear reactors and power generation plant to be found inside The coolant under high pressure in the nuclear reactor deliberately st their has to be retained in a pressure vessel: complete integrity of the containment vessel is important. Torness has a central gas-tight liner formed of 13 mm thick carbon steel and around this a prestressed concrete pressure vessel 5.5 m thick which acts as a biological shield. A vessel under internal pressure is trying to pull itself apart in all directions. The concrete in the pressure vessel must be kept under compression by embedding a dense array of steel wires which are pretensioned before the internal pressure is applied. The stretching of the wires is equilibrated by compression of the concrete. All the internal pressure now does is to reduce this compression. Plastic The use of plastic or composite materials (other than reinforced concrete) in conventional civil engineering structures has not developed very rapidly. Such plastic composites tend to be more expensive than their metallic equivalents so, unless weight is of particular concern (as in aircraft structures), there is no natural economic driver for their use. Carbon fibre sheets have been used to repair or strengthen existing structures to improve their resistance to earthquakes, where their light weight makes them easier to handle and position. Construction companies having been found to be reluctant to blaze a trail in the use of an unfamiliar material, the all-plastic footbridge at Aberfeldy, Scotland (Figure 17) was erected by students (without any need for high capacity lifting ‘engines’). To link the Airbus A380 (Figure 18) and civil engineering may seem fanciful. However, an aircraft is a large structure, and the structural design is subject to the same laws of equilibrium and material behaviour as any structure which is destined never to leave the ground. The aerodynamic loads on the wings are of course an essential part of the loading for an aircraft; for most land-based structures the wind loading will be a lesser component of loading. The A380 is an enormous structure, some 25 m high, 73 m long and with a wingspan of about 80 m; the area of the wings is about 850 m2; and the aircraft can carry some 853 passengers, on two floors, if fitted out only for economy class. For comparison, St Paul’s Cathedral in London is 73 m wide at the transept; and the top of the inner dome, visible from inside the cathedral, is about 65 m above the floor of the nave. 17. Civil engineering contractors have hesitated to tackle new construction materials. The footbridge at Aberfeldy was built entirely from plastic by Dundee University students under the direction of Bill Harvey 18. Any aeroplane is subject to the same laws of structural mechanics as conventional ground-based structures. The Airbus A380 has dimensions which are similar to those of a large building. Use of plastics in aircraft structures provides strength with reduced weight The structure of the A380 makes much use of plastic – composite – materials in its quest for lighter, stronger structures. Such composite materials obtain their desirable strength and stiffness properties from fibres of glass or carbon which are carefully aligned – exactly like the steel in reinforced concrete – in such a way as to best resist the stresses develop6">A Very Short Introduction But the Airbus A380 is also a wonderful example of the elaborate management and organization required for any complex engineering project. The aircraft are ultimately fitted out in Hamburg having been previously assembled in Toulouse. The rear sections of the fuselage come from Hamburg; the wings are manufactured in the UK; some of the front sections of the fuselage are manufactured in St Nazaire, France; the belly and tail of the plane come from Cadiz in southern Spain. These enormous parts are transported from their various origins by sea to Bordeaux on the Atlantic coast of France and then to Toulouse first by canal and then in a road convoy along carefully chosen routes, avoiding tight corners and narrow carriageways but ensuring adequate strength of the road surface. The Airbus A380 is thus typical of many modern projects in its need for the successful integration of a wide range of engineering skills and disciplines. Road materials ‘Before the Roman came to Rye or out to Severn strode, the rolling English drunkard made the rolling English road.’ But after the Romans had left, the English reverted, and created lanes and roads that wound round the natural features of the landscape, skirted fields, linked farms with mills and with market towns – straight lines were the coincidental exception rather than the norm. The Romans had very clear notions concerning road layout – there was a wide paved carriageway with clear areas each side of the carriageway to remove the possibility of cover for ambush. These roads were clearly provided for military purposes. The concept of formal road building also disappeared with the departure of the Romans. The hope was that human and animal feet and cart wheels would compact the ground sufficiently to give a modest stability. But come the rains, and the track would once again become a mire. The farmer taking produce to and from market with a beast of burden could pick his way across the terrain, but armies with their chariots and military equipment (engines) needed something more serious to aid their rapid deployment. (One might imagine that elephants could be used to compact a layer of soil being placed for a road. Unfortunately, an elephant having once traversed a certain placed for a road. Unfortunately, an elephant having once traversed a certain route will remember where it has been and always place its feet in the same spots: pachydermic compaction of an area of soil is not effective.) Road design makes use of designer soils: materials from the ground are modified in order to give particular mechanical properties. There are also special materials that are used for construction of the road pavement (the surface on which the traffic runs). The principles of design of roads have not changed much over two millennia, even if the loading provided by the juggernaut lorries of today considerably exceeds that provided by Roman chariots and soldiers. The materials available for pavement construction have been improved, and the machinery available for placing and compacting those materials has developed – particularly in scale. The Via Appia which leads from Rome to Brindisi on the Adriatic coast, a distance of 360 Roman miles (about 530 km), built over a period of about 60 years around 300 BC, still exists. Traffic requires a strong running surface, resistant to wear. This layer needs some underlying support to prevent it spreading, and an underlying drainage layer to draw rainwater into lateral ditches to prevent the formation of a morass in wet weather. The Via Appia6">A Very Short Introduction Adaptations were needed if the road had to cross swampy ground. The Romans developed a technique using osiers – willow branches – spread across the soft ground both to help distribute the load and also to tie together the road base material in order to discourage it from flowing out sideways. Today road designers use synthetic reinforcing materials – so-called geogrids or geomembranes – to achieve the same result. When the features of good road construction were rediscovered in the 18th century by, among others, Thomas Telford (1757–1834) in Britain, efforts were concentrated on finding ways of producing a tightly packed running surface. John McAdam (1756–1836) became obsessed with the size of stones to be used. He specified a maximum size of 20 mm for the stones: a road-building labourer could check whether a rock had been adequately broken by seeing whether the resulting stones could be fitted into his mouth. This size was chosen to be significantly smaller than the typical wheel rim thickness (100 mm). McAdam reckoned that the severity of Telford’s camber was not necessary to guarantee sufficient runoff of rainwater, so his designs were more economical. The material which we see so often on modern road surfaces, and known as tarmac(adam) or asphalt, preserving his name at least in part, was introduced in the early 20th century. Binding together the surface layers of stones with the early 20th century. Binding together the surface layers of stones with bitumen or tar gave the running surface a better strength. Tar is a viscous material which deforms with time under load; ruts may form, particularly in hot weather. Special treatments can be used for the asphalt to reduce the surface noise made by tyres; porous asphalt can encourage drainage. On the other hand, a running surface that is more resistant to traffic loading can be provided with a concrete slab reinforced with a crisscross steel mesh to maintain its integrity between deliberately inserted construction joints, so that any cracking resulting from seasonal thermal contraction occurs at locations chosen by the engineer rather than randomly across the concrete slab. The initial costs of concrete road surfaces are higher than the asphalt alternatives but the full-life costs may be lower. The eventual process of replacement of a concrete road surface may be more complex and disruptive to traffic flow than the repair of an asphalt surface and the driving experience, registering each construction joint in turn, may be less comfortable: there are various factors which might influence the choice. While an aeroplane is not strictly a product of civil engineering, much of the infrastructure of an airport certainly is. There are buildings of greater or lesser complexity and architectural pretension, there are transport connections both between parts of the airport and between the airport and the outside world, and there are the runways. A runway pavement provides a foundation for a structure (the aeroplane) which can be placed anywhere within its area and which will generate dynamic impact loading as well. A runway is essentially just a short road and is subject to the same design considerations. Just as for heavy lorries, the load of an aeroplane is spread over a number of tyres in order to keep the individual loads to a satisfactory minimum. The runway spreads the load from these tyres into the underlying ground without significant deformation, temporary or permanent, of the runway surface. As with roads, runways may be surfaced with asphaltic or concrete materials. In choosing a material the lik { text-align: justify; margin-top: 1em; margin-bottom: 1em; text-indent: 0.01em; margin-left: as. There ely need for, and frequency of, maintenance and repairwrite in newsp Chapter 2 Water and waste Water is not a construction material but it is of huge importance to mankind, both in the damage that it can do when uncontrolled and in the dependence that we have on its abundant provision. Water and waste illustrate the intricate interdependencies of civilized life. One man’s effluent is another man’s water supply. It is much easier to be casual about outputs until the effect on others becomes overwhelming. A supply of fresh water is one of the essential elements of infrastructure which we often take for granted in towns or cities. Dwellings in remote rural areas may have their own wells or springs which are at the mercy of the weather. Carrying our own water when trekking into remote areas makes us all the more appreciative of the reliable tap back home. Child mortality in the developing world is dramatically reduced when the quality of the water available for drinking and cooking is improved. Failure of waste disposal systems following natural disasters – either directly destroyed or implicitly absent in temporary camps for displaced persons – leads to cholera and typhoid epidemics through contamination. Flying over the western United States or central and western Australia, it is very obvious that the desert is nature’s preference for much of the terrain. Settlements exist only under sufferance and these are certainly dependent on a supply of water from somewhere else, either below or distant from the site. Pumping water from deep wells or boreholes intercepting permeable aquifers uses old water which has probably spent many thousands of years percolating through the ground in order to reach the aquifer (the geological layer in which the water is stored and through which th leaning tower10 carefule water is able to flow). It is evidently quite easy for the rate of pumping to exceed the rate of replenishment, so that the water supply becomes a diminishing resource. Reliance on pumped water to supply an expanding urban area can be precarious. Water collected from rainfall in surface reservoirs or abstracted from rivers can be seen as young water: there is a more evident relationship between quantities of water that are available and demanded. Rivers add their own problems to the water supply. Water abstracted upstream of a town will no doubt be discharged as waste water to be used as input for other a town will no doubt be discharged as waste water to be used as input for other towns further downstream. River water may be used many times before it is eventually allowed to find freedom in the sea. Water Water has been such an essential supporting component of civilization over the millennia that its availability has often governed the location of castles and villages. Equally, the provision of water and handling of waste for towns and cities has often required impressive civil engineering. The island of Samos in the Aegean has a fortified town with the same name on its southern coast. Any fortified castle or town needs a supply of water (and food) in order to be able to survive a prolonged siege. A plentiful spring, separated from the town by the limestone Mount Ampelos (228 m high), provided a possible source. Transporting the water by contour channel would have been vulnerable to attack. The alternative, to tunnel through the mountain for a distance of about 1 km, is described by Herodotus, writing about 60 years after its completion in around 525 BC, as one of the greatest engineering feats of the Greek world. He actually names its designer/engineer (he uses the term architect, ‘master builder’) as Eupalinos. Civil engineers are required not only to design their works but also put them in the intended locations. Skills of surveying are today greatly assisted by precision optical instruments, laser levels and global positioning systems feeding on satellites that are constantly orbiting the earth. Such modern devices may seem a bit like cheating to those surveyors who had to toil up mountains with heavy theodolites, hoping for conditions clear enough to be able to see their colleagues on adjacent peaks, all the time creating invisible triangles across the countryside which would be brought down to earth at a baselength which had been very precisely measured using chains, or rods of metal or glass. The precision that was achieved by such techniques in the 18th century was remarkable. Eupalinos had no serious optical instruments, but he must have used some system of sighting beacons to establish the line of the tunnel under the mountain, and some sort of levelling device to establish the relative levels of the two entrances to the tunnel, constructed simultaneously from both ends. In the event the two tunnel headings met with a separation of 1 m vertically and 6 m horizontally. The water supply was carried in an earthenware channel at the bottom of a slot about 80 cm thick cut from the floor of the tunnel to depths up to 8 m, to ensure the necessary gradient of about 0.6 per cent providing a flow of some 1200 cubic metres per day, sufficient to supply a town of 20,000 inhabitants together with a fleet of ships, an army, and the construction workers brought in for the tunnel itself and other works of fortification. The channel itself was roofed over and the slot backfilled with rock debris. The spring at the north end and the supply reservoir at the city end are linked into the tunnel by buried conduits. The tunnel, 1.8 m × 1.8 m in section, doubled as a subterranean escape route from the fortified town – like the modern Elements of civil engineering design processearthquakeum tunnel in Kuala Lumpur which doubles as a highway and a flood relief channel. Travelling today on the Via Appia, or on other roads heading for Rome, you can see extensive remains of aqueducts conveying water to the city built between 312 BC and AD 226. There are other more spectacular remains of Roman water supply networks. The Pont du Gard, in southern France, supplied some 40,000 cubic metres of water per day to the city of Nîmes from a spring some 50 km away: the water took more than a day for its journey from spring to city. The Romans used lead or earthenware pipes when necessary to cross rivers by forming inverted siphons, but they preferred to use open channels as far as possible. The surveying feats in setting out the necessary low gradients for the flow over tens of kilometres were impressive. Minerals of value, such as gold, are found where the geology is appropriate but not necessarily where there is water available for human or industrial consumption. The gold rush in Western Australia was driven by the same human weakness for the yellow metal as that in North America. The discovery of gold at Kalgoorlie in the 1890s, led ultimately to the creation of one of the largest open-pit mines in the world, but also triggered a need for water. Charles Yelverton O’Connor was appointed as chief engineer for Western Australia in 1892 and, having built the harbour at Fremantle, turned his attention to the problem of water supply for Kalgoorlie. His solution was to build a mass concrete dam in the hills just to the east of Perth and then construct a 0.75 m diameter pipeline for some 500 km through which to pump the water, with a series of eight pumping stations. The project took about five years to complete and water started flowing through the pipeline in 1903. Sadly, the constant sceptical criticism of the project and false accusations of corrupt practices to which he was subjected led O’Connor to commit suicide in 1902 by riding his horse into the sea. He is now regarded as a local hero. Waste A good supply of fresh water is one essential element of civilized infrastructure; some control of the waste water from houses and industries is another. The two are, of course, not completely independent since one of the desirable requirements of a source of fresh water is that it should not have been contaminated with waste before it reaches its destination of consumption: hence the preference for long aqueducts or pipelines starting from natural springs, rather than taking water from rivers which were probably already contaminated by upstream conurbations. It is curious how often in history this lesson has had to be relearnt. At Mohenjodaro on the Indus River in what is now Pakistan from 2500–1500 BC there was an extensive network of drainage channels beneath the streets, lined with fired bricks and leading to soakaways beyond the urban area. The Minoans in Crete provided a similarly elaborate flushed drainage system beneath the palace at Knossos at around the same period. However, the Greeks did not spot the advantage of such a drainage scheme, and waste was thrown in the streets in a way that continued in many European cities into the 18th century. The inhabitants of Rome did rather better. Large underground channels were constructed to drain low-lying areas of the city and channel rainwater from the streets. Lesser drains carried waste from public latrines, flushed by water from the public bath-houses, efficiently using grey water for this purpose, a concept that has been adopted in the past century in areas where water is in short supply (and which could rationally be adopted more generally). However, such municipal engineering for the public sources of power in nature4 experiencebenefit did not extend far outside the Imperial City. Visitors to the ruins of the town of Pompeii (240 km from Rome), destroyed by the eruption of Vesuvius in AD 79, will recall the paved main streets with raised pavements and occasional stepping stones. The spacing of the stones allowed for the spacing of the chariot wheels; while the gaps between and the height of the kerbs provided space for rainwater to flow and in the process clear some of the animal and human waste and other detritus from the streets. Pompeii did have a water supply to the public baths and street-corner fountains but did not have any organized subterranean system of sewage collection and disposal. Some houses had latrines, usually located in diminutive kitchens so that cooking waste could join everything else in the cesspit. London embankment sewer In the 19th century, the Industrial Revolution had resulted in a huge expansion of the city of London, but its estuarine location meant that gradients were shallow and there were no mountain torrents to arrive fresh from the springs, either to provide a supply of pure water or to provide a means of clearing the streets. From the early 17th century water had been brought by a New River fr