Chpt 7 + 9.docx (1) PDF - Stars and Cosmology
Document Details
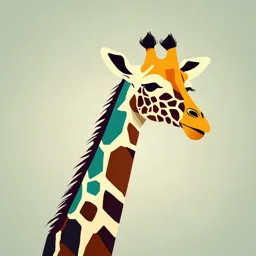
Uploaded by AdulatorySanJose9099
Tags
Summary
These notes cover fundamental concepts in astronomy and cosmology, exploring the life cycle of stars, the formation of stars from nebulae, the balance between gravity and radiation pressure in stars, the death of stars and the Big Bang theory, and basic concepts of celestial bodies.
Full Transcript
7.1 - Stars Accretion: Process in which particles of dust and rock slowly come together due to gravity to form a larger object. Binary Star System: Two stars that orbit a common centre of mass. Black Hole: Also known as a singularity, a collapsed star so massive that not even light escape from its g...
7.1 - Stars Accretion: Process in which particles of dust and rock slowly come together due to gravity to form a larger object. Binary Star System: Two stars that orbit a common centre of mass. Black Hole: Also known as a singularity, a collapsed star so massive that not even light escape from its gravitational field. Galaxy: A large group of stars attracted to one another by gravity. Gravitational Lensing: The bending of light rays due to the distortion of space caused by a massive object such as a black hole. Gravity: The force that causes all matter to collect together. Interstellar: Space between stars Light-Year (l.y.): The distance light travels in a year, approximately (9.5 × 1012) km. Milky Way: The galaxy in which the solar system is located. Nebula: A cloud of gas found in the empty space between stars; birthplace of new stars. Nuclear Fusion: Process where hydrogen gets converted into helium to produce light and heat. Parallax: A technique used to measure the distance to other stars. Parsec: An astronomical unit of length equal to 3.26 light-years. Protoplanetary Disk: Disk of gas surrounding a protostar that will form into planets. Protostar: A collapsing cloud of gas that will eventually become a star. Radiation Pressure: The force produced by radiation from pressure caused by heat. Stars: Discs of gas → C, H, He and Fe Stellar Parallax: The apparent change in the position of a star throughout the year due to the Earth’s motion around the Sun. Super-massive Black Hole: A black hole millions or billions of times the mass of our Sun found at the centre of a galaxy. Astronomy → Study of stars. Not all stars are like our Sun. Like people, stars can vary in age, size and appearance. Understanding the differences between stars will help us understand our universe. Gravity And The Universe: Gravity → Force of attraction experienced between any two objects in the universe. o It is the force that drives the universe. o Greater Mass = Greater Force but Greater Distance = Smaller Force. Gravity is an extremely weak force that depends on the masses of the two objects. These masses need to be incredibly large for the force to become significant. o The force of gravitational attraction between you and a person sitting next to you is less than 1 µN → One-millionth of a Newton. o Weight of a tennis ball is 0.5 N → You won’t feel the attraction. When one of the objects is as massive as a star, gravity becomes an extremely strong force. o Stars have gravity to keep gases coming towards its centre. o Because of gravity, stars can have different colours. It also creates immense pressures within the star that hydrogen atoms at the star’s centre become fused together to form atoms of helium → Nuclear fusion. Nuclear fusion produces the enormous amounts of heat and light that causes stars to glow. The structure of any star is determined by the balance between two opposing forces: o Inward force of gravity → Causes materials within to fall towards the centre o Outward force of gravity → Results from pressure caused by the heat radiated from nuclear fusion of stars (Radiation Pressure). Depending on its size, each star has a different balance point → Variety of stars. o Each star has a different balance point, depending on its size. This gives rise to a variety of different types of star → From average-sized stars like our Sun to massive red giants and blue supergiants. Stars: Large balls of hot gas. They look small because they are far away. Many are bigger and brighter than the sun. The heat of the star is made in the centre by nuclear fusion reactions. There are lots of different colours and sizes of stars. Creation Of Stars: Stars are made in giant clouds of dust and gas. Sometimes part of the cloud shrinks because of gravity. As it shrinks, it becomes hotter and when it is hot enough, nuclear reactions can start in the centre, and a star is born. Thermodynamic Equilibrium: The balance between gravity trying to make the star shrink and heat holding it up. Once nuclear fusion is producing heat in the centre of the new star, this heat stops the rest of the star from collapsing. The star then stays almost exactly the same for a long time (about 10 BY for a star like the sun). Colours Of The Star: Different stars are different colours, size and brightness. The bigger a star, the hotter and brighter it is. o Hot stars are blue. Smaller stars are less bright, cooler and red. Because they are so hot, the bigger stars actually have shorter lives than the small cool ones o Stars in the countryside are more visible than those in cities because there are less high-rise buildings and a low amount of pollution. Death Of A Star: Eventually, the hydrogen in the centre will run out. No new heat is made and gravity will take over and the centre of the star will shrink. This makes the very outside of the star 'float up' and cool down, making the star look much bigger and redder, a red giant star. As the centre collapses, it becomes very hot again, eventually getting hot enough to start a new kind of nuclear fusion with helium as fuel. The red giant shrinks and appears normal again. However, helium runs out quickly and the red giant forms again. End Of Sun-Like Star: No more nuclear fusion can take place so the centre of the star will then keep collapsing. A very dense object called a White Dwarf is formed as a result. o A white dwarf the size of a mobile phone would weigh as much as an elephant on the Earth. The outer parts of the star then drift off into space and cool down making a Planetary Nebula. o Planetary nebulae have nothing to do with planets, they just look a bit like them in small telescopes. For stars bigger than sun, their collapse cause a huge explosion called a Supernova. o A supernova can be brighter than an entire galaxy of 100,000,000,000 stars!! Supernova explosion leaves behind a ‘supernova remnant and a neutron star’, or even a Black Hole. The Overall Life Cycle Of Stars: Gravity is responsible for creating stars from nebulae (huge clouds of interstellar gas) 1. Stars are born in a nebula → a cloud in deep space made of dust and gases, mainly hydrogen. 2. The slow pull of gravitational forces acting over enormous periods of time causes the gas and dust to clump within the nebulae causing it to heat up and spin. o When a nebula has a high gravitational force, it pulls gases, particles, or even other stars towards itself. o This forms a large, dense clump known as a protostar. 3. When the protostar becomes hot enough, hydrogen atoms in the core (or centre) begin to fuse, producing helium and energy (nuclear fusion). o Causes the star to radiate heat and light to space and our Sun was born. o This phase of the star's life is called the main sequence. o This is the longest life stage of a star and lasts for billions of years. o This sequence is also where our sun is at the moment. When the hydrogen in the core of a main sequence star runs out, it undergoes a dramatic transformation and moves off the main sequence. There Are Two Types Of Life Cycles: a) Life cycle of a low-mass star (e.g. sun): a) ⮚ Nebula→ Protostar → Main Sequence Star →Red Giant → Planetary Nebula → White Dwarf → Black Dwarf ⮚ When hydrogen in the core of a medium-sized star runs out, the core shrinks and hydrogen fusion begin in the outer layers, which then expands the entire star, turning it into a Red Giant. Because the star expands, the energy is spread across a larger surface area, making it cooler, and causing it to shine more red → Towards the red part of the spectrum. As the hydrogen in the outer layers fuse, the helium produced sinks to the core of the star. This increases the gravitational force and temperature causing the helium to fuse into heavier metals (e.g. carbon). A red giant has enough helium fuel in its core to last for around 100 million years. o When a red giant runs out of helium fuel in the core, the core collapses further and the outer layers become a planetary nebula. o Over a ‘short’ period of 20 000 years, the planetary nebula will disperse to reveal the hot, dense core of the red giant called white dwarf. o The white dwarf then cools to form a black dwarf. b) Life cycle of a greater mass than sun: b) ⮚ Nebula → Protostar → Main Sequence Star → Red Supergiant → Supernova → Neutron Star Or Black Hole ⮚ Protostar And Nebula: o A protostar is the earliest stage of a star. ▪ It has a large amount of clouds of hydrogen, helium and dust. o A nebula is a cloud in space consisting of gas or dust/ dirt. ▪ Nebulas later form protostar before nucleosynthesis has set in. ▪ Nucleosynthesis: Creation of new atomic nuclei o It takes a hundred thousand years for nebula star to form or to reach a main sequence. o Before the last sequence, a star has large amount of clouds of hydrogen, helium and dust. o It is the nebula star that becomes hotter and denser, which is called as a protostar. The Distances Between Stars: The distances between stars are enormous. o The bigger a star is, the smaller is the distance between them and neighbouring stars. The closest star to us (other than our Sun) is Proxima Centauri → 4 x 1013 km Distances, such as these, mean that the kilometre is not a convenient unit of length. Astronomers often measure interstellar distances (the distances between stars) in: o Light-years (l.y.): ▪ Distance that light will travel in one year → 9.5 trillion (9.5 × 1012) kilometres. * 1 light year = 9.5 x 1012 km ▪ A light year is a unit of distance not a unit of time. * A light minute and a light second are units related to light year. ▪ The distance to Proxima Centauri is 4.2 l.y. → 4 × 1013 km away. * This means that the light you see when you look at Proxima Centauri was emitted by the star nearly 650 years ago. ▪ Looking at stars is like looking backwards in time. o Parsec (pc): ▪ Distances that are even more further away are measured in parsec (pc). * One parsec = 3.26 light years ▪ Converting between light-years and parsecs * Light-years into Parsecs → Divide by 3.26 * To convert from parsecs to light-years, multiply by 3.26: Distance in light-years = distance in parsecs × 3.26 1) The star Betelgeuse is 650 light-years away. Calculate how many parsecs this represents: Distance in parsecs = 650 ÷ 3.26 = 199 pc 2) Beta Centauri is 107 parsecs away. Calculate how many light-years this represents: Distance in light years = 107 × 3.26 = 349 l.y 3) Calculate what the following distances would be in parsecs: a) Proxima Centauri, 4.2 l.y. away b) Antares, 10 p.c. away: Parallax: The parsec is based on a phenomenon known as parallax. Parallax causes you to see different views of the same object. Parallax can be used to measure the distance between the Sun and other stars. o As the Earth moves around the Sun, our changing point of view means that the positions of stars in the sky change very Even for the closest stars, this shift in position (known as stellar parallax) is tiny → Less than one-thousandth of 1 degree. It is only since the 19th century that astronomers have had instruments sensitive enough to reliably measure stellar parallax and calculate the distance to the nearest stars. Many stars are so far away that their stellar parallax is too small to be measured. o Astronomers need to use more indirect methods to measure the distances to these stars. Parallax In Science Experiments: Parallax error → Occurs due to incorrect positioning of the eyes while taking a reading on a measuring scale. Must read liquid measurements exactly at eye level, not slightly up nor down. You cannot look at a star ‘eye level’, so you measure the parallaxes and even it out. Tiny Angles: The closer a star is to our Sun, the bigger the parallax. The closest star to our Sun is Proxima Centauri, 4.2 light-years away. This corresponds to a parallax of about 0.0004 degrees → A very small angle to measure. Formation Of The Sun: Gravity creates stars from Nebulae. Most of the gas in Nebulae was hydrogen, with small amounts of helium and heavier elements such as carbon and iron. The slow pull of gravitational forces acting over enormous periods of time caused the cloud of gas to gather into a large clump known as a protostar. o 4.5 billion years ago the region of space now occupied by our solar system was filled with a featureless cloud of gas. This protostar would eventually become our Sun. Material that was too far away from the Sun to be drawn into it clumped together to form the planets. Galaxies: Although gravity is a relatively weak force, it can act over enormous distances, even the incredible distances between stars. Over billions of years, the gravitational attraction between ‘close’ stars have caused them to clump together into gigantic structures known as galaxies. The size of galaxies vary greatly but all galaxies contain an enormously large number of stars, usually between 10 million (107) and a hundred trillion (1014). Our own Sun is part of a galaxy known as the Milky Way. Beyond the Milky Way, even the closest galaxies are so far away that they show no observable parallax. o Alternative techniques need to be used to measure the distances to them. Black Holes: Most spectacular gravitational phenomena in the universe. The gravitational field of a black hole is so strong that not even light is fast enough to escape from it. This makes black holes hard to detect as they do not emit any visible light. However, it is possible to find black holes indirectly by the effect they have on other stars. o A black hole is easier to detect when it is part of a binary star system. * This occurs when two stars form close to one another and orbit a common centre of mass between them. o If one of these stars becomes a black hole, its enormous gravitational field will start to strip material from the other star. o As this material spirals into the black hole, it emits a distinctive high-energy X-ray signal that indicates the presence of the black hole. Process: Another way to detect a black hole is by a process called gravitational lensing. o According to Einstein’s theory of relativity, the gravitational field around a black hole is so strong that it can distort the shape of space itself. * This means that light from a distant star passing either side of the black hole can be bent back towards an observer on Earth. o Due to this lensing effect, the observer sees identical stars on either side of the black hole. o Scientists now believe that most galaxies have an enormous black hole at their centre. o These super-massive black holes may have masses equivalent to millions or billions of stars the size of our Sun. o Evidence suggests that the black hole at the centre of our Milky Way galaxy lies in the constellation of Sagittarius. Our Solar System: Terrestrial/Rocky Planets → Made of rocky particles: 1. Mercury - Closest to the sun; rotates around the sun in 88 days and on its own axis in 58 days; no atmosphere; has glaciers; called morning star (bright) 2. Venus - Size of earth; ‘earth’s twin’; no moons or rings; hot, thick atmosphere; brightest object in sky besides sun and moon; covered with craters, volcanoes and mountains. 3. Earth -Only planet to have life and liquid water; atmosphere composed of N(78%), O2 (21%) and other gases (1%). 4. Mars - ‘The Red Planet’; surface covered with iron oxide (proof of once free O molecules); volcanoes and huge dust storms; 2 moons (Phobos and Deimos) Gas Giants: 5. Jupiter - Largest planet in SS; 60+ moons, 5 visible from earth; strong magnetic field; giant red spot; rings have 3 parts (Halo ring, Main ring, Gossamer ring) 6. Saturn - 7 thin, flat rings made of ice, dust and rock particles; 31 moons (largest Titan); visible in the night sky; lightweight plante, spins fast; explored by Voyager. Ice Giants: 7. Uranus - Faint ring system; 27 known moons; covered with clouds; sits and spins on its side with N and S poles sticking out the sides. 8. Neptune - Discovered through Math; 7 known moons (largest is Triton); storm called the ‘Great Dark Spot’ appears as a dark oval (thought to be hole similar to the one in ozone of earth) Magellanic Clouds: 2 closest galaxies near the Milky Way → Large Magellanic Clouds (LMC) and Small Magellanic Clouds (SMC). o Easy to observe with the naked eye. Ferdinand Magellan was sent by the king of Spain to make the first circumnavigation (trip around the circumference) of the world. Killed in a battle by natives. Questions: 1) Name The Astronomer Who First Recorded Their Observations Of The Large Magellanic Cloud: Al-Sufi, Persian Astronomer In 964 CE 2) Identify The Century In Which The Magellanic Cloud Were First Observed By Europeans: 16th Century (1500s) 3) Recall A Story Of Indigenous Australians That Is Associated With These Clouds: Clouds Are An Old Man and woman or two ‘lawmen’ whose fire sparks into the sky. 7.2 - Colour And Magnitude Absolute Magnitude: A measure of how bright a star would appear if it was 10 parsecs from Earth. Apparent Magnitude: A measure of the brightness of a star as it appears to an observer on Earth. Blue Supergiants: Stars that are ten or more times more massive than the Sun. Black Dwarf: Cold dark remains of a white dwarf. Density: Mass per unit volume of a material. Electromagnetic Spectrum: Different types of electromagnetic radiation ranging from radio waves to gamma rays. Gamma Rays: Very high-energy electromagnetic rays. Isotope: Atoms with the same number of protons but different numbers of neutrons. Magnitude: A measure of the brightness of a star. Main Sequence: A group of stars lying on a line running from the top left to the bottom right of the H–R diagram. Neutrino: An almost mass-less, neutral particle released during some nuclear reactions. Neutron Star: Remnant of a supernova, consisting entirely of neutrons. Plasma: State of matter consisting of positively charged ions and free electrons. Positron: A positively charged electron. Planetary Nebula: A cloud of gas produced when a red giant runs out of fuel. Red Giant: A star produced when the core of a Sun-sized star runs of hydrogen. Spectral Class: A classification system for stars based on their colour. Spectrometer: A device that splits light into a spectrum to show its component wavelengths. Supernova: A giant explosion that occurs when a star many times larger than our Sun runs out of nuclear fuel. White Dwarf: Hot, dense star that is the remains of a red giant. The universe is not a static, unchanging place. Much like a living organism, each star has a history → The star is ‘born’ and eventually it will ‘die’. Astronomers can uncover a star’s history by carefully observing the light it produces. o This gives us clues to its size and temperature, how it formed and even what will happen to it at the end of its life. Brightness: Stars differ from one another in brightness. Astronomers refer to the brightness of a star as its magnitude. A star’s apparent magnitude is a measure of how bright it will appear to an observer on Earth. Brightest stars are given the lowest magnitudes, while dimmer stars are given higher magnitudes. o The very brightest stars are given negative magnitudes. o Brighter star = Lower magnitude o Darker/dimmer star = Higher magnitude. * Alpha Centauri (the brighter of the two Pointers to the Southern Cross) has an apparent magnitude of –0.27. o Under ideal conditions, the human eye can see stars down to a magnitude of 6.5 → Limit Apparent magnitude is measured on a logarithmic scale. A change of one unit on this scale changes the brightness of the star by a factor of about 2.5. o Two of the brightest stars in the constellation Orion are Betelgeuse and Bellatrix. * Betelgeuse is the bright red star that makes the right shoulder of the hunter in the constellation → Bellatrix is the hunter’s left shoulder. * Betelgeuse → Apparent magnitude of 0.6 * Bellatrix → Apparent magnitude of 1.6. * Betelgeuse’s apparent magnitude is one unit lower than that of Bellatrix, so it appears about two and a half times brighter than Bellatrix. There are two main factors that determine a star’s apparent magnitude: o The amount of light the star emits o The distance between the star and Earth → The greater the distance between the star and Earth, the dimmer the star will appear. In order to study stars, it is important to eliminate the effect of distance on our measurements. Absolute Magnitude: Measure of a star’s actual brightness. This measures how bright a star would appear to us if it was a distance of 10 parsecs from Earth. Using absolute magnitudes allows the brightness of stars from different parts of the galaxy to be meaningfully compared. o Betelgeuse → 200 pc from Earth, has an absolute magnitude of –5.14. o Bellatrix → 75 pc from Earth, has an apparent magnitude of –2.72 o If Betelgeuse was 10 pc away, it would be 200 pc brighter than is currently. And if Bellatrix and Betelgeuse were equi-distant from Earth, Betelgeuse would still be brighter. Colour: The colour of a star is due to its temperature and the elements it contains. Each star emits light at a range of different wavelengths. Some of this light is in the visible part of the electromagnetic spectrum, while some of it will be in the invisible infrared or ultraviolet range. Your eyes collect the visible light from stars and your brain performs a complex averaging process that results in you perceiving the star as a particular colour. Rather than rely on the human eye and the brain to interpret the colour, scientists analyse the light from a star by using filters. A star’s colour can be precisely measured by comparing the magnitude of the star when viewed through coloured filters. A star’s spectrum is mainly determined by its surface temperature. o Cooler stars emit most of their energy in the infrared and visible red parts of the spectrum and therefore appear red to your eyes. o Very hot stars emit a lot of energy in the visible violet and ultraviolet part of the spectrum and appear blue. o Stars with temperatures in between these extremes emit light across a range of wavelengths and can appear orange, yellow or white. Spectrometer: This is a device that splits light into a spectrum to reveal its component colours. Used to analyse starlight and determine what chemical elements are present in a star from distinctive lines that appear in its spectrum. Particular elements emit colours of particular wavelengths. These can be measured precisely to determine the elements in the star. When studying the spectra from stars, scientists also see dark lines showing missing colours. Fraunhofer lines: Fraunhofer - Dark lines (of missing colours) due to light interacting with atoms in outer layer of stars. The light energy is absorbed by electrons in atoms of all the elements in the outer gas layers. These electrons absorb light energy of particular wavelengths. The absorption occurs at exactly the same wavelength that the element would emit when it is extremely hot. Using the spectra from different stars, scientists have created a classification system called spectral class. Spectral class indicates the elements present in the star, the temperature and colour of a star. o Our Sun → Surface temperature of just under 6000°C so it is classified as a G-type star. o It has a yellow colour. Seeing the colour of stars: The colours you see in the stars seem much clearer than those you see when you look at the night sky. o The colour receptors in your eyes require a lot of light and do not work well at night. o Our eyes have difficulty in detecting the colour of small points of light. The long exposure time used in taking images makes many small stars appear as small discs instead of points of light. o This makes their colour easier to see. Nuclear fusion: Data from spectral analysis indicates that ¾ of material in a typical star is hydrogen. Most of the remaining quarter consists of helium, small amounts of oxygen and other heavy elements. The enormous gravitational forces within a star can heat the material at its centre to a temperature of almost 15 million degrees Celsius. Hydrogen is the simplest element in the periodic table, consisting usually of a single proton and an electron. At the enormous temperatures inside a star, the electrons have too much energy to stay bound to the protons, so the material takes the form of plasma. o Plasma is a state of matter consisting of a ‘soup’ of positively charged ions and free electrons. Protons are positively charged and so they strongly repel each other. However, in the centre of a star, the massive gravitational force is enough to bring individual protons close enough to fuse together into a new nucleus. During fusion, one of the protons is converted into a neutron and two tiny particles are released → A small positively charged particle (positron) and a tiny, neutral particle (neutrino). o A positron is the antimatter particle for an electron. * It is identical to an electron except that it has a positive charge. o The positron does not stay in existence for long. o As it is positively charged, it will be attracted to any electrons in the plasma. o When a positron collides with an electron, the two particles annihilate (destroy) each other and become two high-energy gamma rays. o As these gamma rays make their way out from the core of the star, their wavelengths increase and they are stretched into the heat, light and UV radiation that’s observed from Earth. The new nucleus formed in this fusion reaction consists of a proton and a neutron. o This is still the nucleus of a hydrogen atom, but it is an isotope of H known as deuterium. o The deuterium can undergo further fusion reactions. o The overall result of all these reactions is that hydrogen is converted into helium, neutrinos and energy. Neutrinos: Extremely difficult to detect → No mass, no charge, not affected by electric or magnetic fields. It is suspected that trillions of neutrinos produced by the Sun pass through your body each second without having any effect on you at all. The Life Cycle Of Stars: In the early part of the 20th century, two astronomers, Ejnar Hertzsprung of Denmark and Henry Norris Russell of the United States, independently came up with the idea of plotting stars on a diagram. Absolute magnitude (brightness) was placed on one axis and spectral l class (colour) on the other. When they did this, they noticed that the stars fell into a number of clearly defined groups. This type of diagram became known as the Hertzsprung–Russell or H–R diagram. Hertzsprung—Russell diagrams: The H–R diagram revolutionised astronomy because it showed that there was a relationship between the brightness and temperature of stars. H–R diagrams were also interpreted as showing that stars were changing from one ‘type’ to another. These changes became known as the ‘life cycle’ of a star. Using an H–R diagram is a bit like going into a forest and seeing all the trees at different stages of growth and concluding that the stages represent different life stages of the species. o You can’t actually see a tree at one stage grow into the other, but it is clear that they must. Main Sequence: On the H–R diagram, most stars fall on a broad line running from the top left-hand corner to the bottom right-hand corner. o This line is known as the main sequence. In a main sequence star, gravity and radiation pressure are in equilibrium → They balance each other out, giving the star a constant radius and brightness. This equilibrium can last for millions or even billions of years until the hydrogen in the core of the star starts to run out. For main sequence stars, there is a simple relationship between the mass of the star and temperature and brightness → The heavier the star, the hotter and brighter it will be. This is because more mass results in a greater gravitational force. The greater gravity from the large mass causes the core of the star to be more tightly compressed and therefore nuclear fusion occurs more rapidly. Hydrogen is converted into helium more quickly and produces more heat and light. This also means that more massive stars burn up their fuel more quickly. o Our Sun is a medium-sized star on the main sequence. o At a temperature of 6000°C, our Sun’s nuclear fuel will last for about 10 billion years. o In comparison, a star ten times as massive as the Sun will be 10 000 times brighter, have a temperature of 22 000°C and burn out in just 20 million years. On an H–R diagram, the stars in the top left-hand corner are brighter, hotter and larger. Stars in the bottom right-hand corner are dim, cool and small. Typical stars in the main sequence start their lives at the bottom right-hand corner of the main sequence and move towards the top left of the H–R diagram. When the hydrogen in the core of a main sequence star runs out, it undergoes a dramatic transformation and moves off the main sequence. Where it goes next on the diagram depends on its mass. Red Giants: When the hydrogen in the core of a medium-sized star runs out, fusion stops and the outward radiation pressure also stops. Gravity causes the star to collapse inwards and the outer layers of the star to start to fuse. Heat from this fusion produces radiation pressure, which causes these outer layers to expand and cool. The star becomes a red giant with a small dense core and a large, relatively cool outer atmosphere. Fusion in the outer layers of a red giant occurs at a lower temperature than in a main sequence star. A red giant produces more light in the red part of the spectrum, giving the star its distinctive red colour. As the hydrogen in the outer layers of the red giant fuses, the helium produced sinks into the core of the star. As more and more matter is added to the core, its gravitational force and temperature increase until helium atoms start to fuse into heavier elements e.g. carbon. Typically, a red giant that has formed from a medium-sized star has enough helium fuel such as beryllium and helium fuel in its core to last for around 100 million years. When this runs out, the star collapses further and the outer layers escape to become a cloud of gas known as a planetary nebula. o Almost half of the mass of the original star is lost into this planetary nebula. The remaining core of the red giant is extremely hot and emits ultraviolet light. o This causes the planetary nebula to glow in spectacular patterns. Over a ‘short’ period of 20 000 years, a planetary nebula will disperse to reveal the hot, dense sphere of carbon and hydrogen that is the remains of the red giant (its core). This fades to become a very dense star called a white dwarf. White dwarfs are so dense that if our Sun became a white dwarf, its mass could be packed into a sphere only slightly larger than Earth. Although a white dwarf is very hot, it is much dimmer than the red giant from which it forms. o The lower brightness now places it off the main sequence and this means that the white dwarf drops to the bottom of the H–R diagram. Nuclear fusion has ceased in a white dwarf, so the star will eventually fade to become a cold, dark ball of inert matter known as a black dwarf. Since white dwarfs are very small and have a relatively small surface area, they radiate heat very slowly. o It would take hundreds of billions of years for white dwarf to turn into a black dwarf. Scientists currently believe that the universe is less than 20 billion years old, so black dwarfs exist only in the imaginations of astronomers. Supergiants: Stars that are ten or more times as massive as our Sun follow quite a different life-cycle from the stars on the main sequence. These massive stars start at the top left-hand corner of the H–R diagram and are known as blue supergiants. Being much larger than main sequence stars, they have much higher gravitational forces and consume their hydrogen much more quickly → In millions rather than billions of years. o When the hydrogen runs out and helium fusion begins, the star maintains its brightness but it slowly cools down. o On the H–R diagram, this is seen as movement from left to right. Once all the helium has been fused into carbon and oxygen, the temperature in these massive stars is hot enough to cause these atoms to fuse into heavier and heavier elements. Eventually though, even this fuel will have to run out and then the results are nothing short of spectacular—a supernova occurs. Supernova—‘Big, New Star’: In Latin, the word nova means ‘new’. This term was originally used by the earliest astronomers because it seemed that a ‘new’ star was being born. A nova would appear, burn brightly for a few weeks or months and then fade dramatically. If a nova was particularly spectacular, it came to be known as ‘supernova’. Scientists now understand that a nova or supernova is not a new star at all. The star that exploded into the supernova was always there, it may just have been too dim for astronomers to notice it. Supernova/e: Once all the material in the core of a supergiant has been fused into iron, it cannot easily fuse any further. Creating elements that are heavier than iron absorbs more energy than it releases. If the core of a star stops producing energy, there is no force to act against gravity and all of the material in the outer layers collapses inwards at incredible speeds. When this material reaches the solid core, it rebounds in a massive explosion called a supernova. The star will become over 100 million times brighter than it was originally, outshining the rest of the stars in its galaxy combined. In 1987, astronomers were fortunate enough to capture this process occurring in a star in. When a star explodes this violently (and becomes much brighter), much of its mass is blown into space. Supernovas are mostly in the form of neutrons, which collide with other atomic nuclei as they are flung outwards. Many of the neutrons are captured by these nuclei to form heavy elements such as gold and silver. What happens to the material left behind by this explosion depends on the size of the star. Big Star (Massive Star): o Core collapses more violently into a black hole after a supernova. Small Star (Less Massive Star): o Forms neutron stars after undergoing supernova of low energy. Neutron Stars → Small Stars: If the amount of material left behind by a supernova is between 1.4 and 3 times the mass of our Sun, then gravitational forces are strong enough to cause the structure of the atoms within it to break down. Electrons and protons combine to form neutrons. The resulting neutron star has an enormous density since its entire mass can be compressed into a sphere about 10–15 km across. Black Holes → Big Stars: For supernova remnants that are more than three times the mass of our Sun, the process of collapse after a supernova does not end with the formation of a neutron star. The immense gravitational forces cause the star to shrink even further into what scientists refer to as a singularity or black hole. The initial size of a star is critical in determining its life cycle and the type of star it will eventually become. 7.3 - Cosmology Big Bang: Theory that the universe began with an enormous explosion of energy. Blue-Shift: The compression of light waves due to the motion of stars towards Earth; blue-shift makes light appear bluer than it should. Cosmic Microwave Background Radiation: The afterglow of the Big Bang; low-energy radiation that fills the universe. Cosmology: The study of the history and structure of the entire universe. Doppler Effect: The expansion or compression of waves due to the motion of the object making the waves. (Cosmological) Red-Shift: The stretching of light waves due to the motion of stars away from Earth; red-shift makes light appear redder than it should. Steady State Theory (Aka Infinite Universe Theory): Now discounted (disregarded) theory that the universe has always existed in the form that it is in today. Many cultures and religions provide their own answers to the questions such as ‘Where did the universe come from?’ and ‘How will the universe end?’ The answers given by scientists must match observable evidence such as the composition and structure of the universe around us. Galaxies: Our modern understanding of the structure of the universe began with the Ancient Greek philosopher Democritus. In the 4th century BCE, he suggested that the Milky Way could be made up of many distant stars. This idea was rejected by other philosophers of his time, who believed the Milky Way was an atmospheric phenomenon. o Democritus’ theory was not confirmed until the 17th century. This was when Galileo Galilei (1564–1642) looked through his newly invented telescope at the Milky Way and saw its individual stars. Astronomers now know that the Milky Way is just one of billions of galaxies in the observable universe. There are 3 types of galaxies → Elliptical, Spiral (and barred spiral) and Irregular Galaxies. This has caused scientists to change their estimate of the size of the universe. The Milky Way is estimated to contain between 200 and 400 billion stars and be about 100 000 light-years across. The Canis Major dwarf galaxy is the closest galaxy to the Milky Way. o It is 25 000 light-years away from us. Only four galaxies are closer than 2 million light-years away. Most galaxies are many millions of light-years away. Recent estimates put the number of observable galaxies at around 500 billion. This makes the universe unimaginably large. Measuring Distances To Galaxies: The distances to other galaxies are far too big to be measured by parallax methods. One of the best techniques for measuring the distance to another galaxy uses a special type of star known as a Cepheid variable. A Cepheid is a type of star that has variable brightness—over a certain period of time, it changes from bright to dark and back to bright again. * The period of this variation is directly related to the absolute magnitude of the star. o To measure the distance to another galaxy, an astronomer must first identify a Cepheid variable inside the galaxy. o Then, by measuring the period of variation of the Cepheid, the absolute magnitude of the star can be determined. o By comparing this to the star’s apparent magnitude, the distance to the star (and the galaxy that contains it) can be calculated. Steady State Theory: Fifty years ago, the most popular cosmological model was the steady state or infinite universe theory. This theory suggested that the universe is infinite in extent and has always existed in roughly the same form as observed today. This theory matches the fact that galaxies seem to be spread relatively evenly across the sky. This theory was expressed in its most complete form in 1948 by English astrophysicist Sir Fred Hoyle (1915–2001). Big Bang Theory: The first evidence to undermine the steady state model was American astronomer Edwin Hubble’s discovery that the universe is expanding Hubble used Cepheid variables to measure the distance to a number of galaxies. He then carefully observed the spectrum of light from these galaxies and discovered that, in almost every case, it was distorted in a manner known as cosmological red-shift, or just red-shift. Red-shift is similar to a phenomenon known as the Doppler effect. The Doppler Effect: The Doppler effect happens because waves produced by a moving source are either lengthened or shortened due to the motion of the source. You may notice the Doppler effect when something fast and noisy passes by. o For example, the siren of the ambulance sounds as if it has a much higher pitch as it approaches you than when it is has passed you. o You hear the same effect when an aircraft flies overhead or a mosquito buzzes past your ear. Red-Shift: A phenomenon similar to the Doppler effect can be observed with light emitted from stars and galaxies. Light from stars moving towards us will be compressed, making the light appear bluer than it should → Blue-shift. Red-shift is what happens when light from stars moves away from us →light shifts towards the red end of the spectrum, making it appear redder. Hubble’s observation was that the light from almost all galaxies was red-shifted. Hubble’s measurements of this red-shifted light led him to the following conclusions: o Almost all galaxies in the universe are moving away from the Milky Way galaxy. o The further away a galaxy is, the more its light is red-shifted. * This means that the more distant galaxies are moving away from the Milky Way faster than the closer galaxies are. Taken together, these observations suggested that the universe is expanding. This conclusion has important implications: o If the universe is expanding, then it is reasonable to assume that at some point all the matter in the universe was condensed into one point. o This represents the birth of the universe, the moment of an enormous explosion of energy now known as the ‘Big Bang’. Stretching Space: Cosmological red-shift is not caused by the Doppler effect since light waves do not travel through a medium as sound waves do through air. The expansion of the universe should not be visualised as stars expanding out into empty space. Instead, it is the space between stars that expands. It is the expansion or stretching of space itself that causes electromagnetic radiation to be stretched and become red-shifted. Cosmic Microwave Background Radiation: In 1965, two American astronomers, Arno Penzias (1933–) and Robert Wilson (1927–2002), were trying to study radio signals from the Milky Way. They kept finding an annoying background signal coming from all directions in the sky that interfered with interfered with their measurements. By chance, they called a cosmologist, Bob Dicke (1916–1997), who realised that this background signal was the ‘afterglow’ of the Big Bang. o It was radiation emitted approximately 400 000 years after the Big Bang. This afterglow is now known as the cosmic microwave background radiation. It is consistent with predictions that radiation from the early universe should have been red-shifted into the microwave part of the spectrum by the expansion of the universe since the Big Bang. It was this discovery, together with Hubble’s demonstration of the expansion of the universe, that convinced most scientists to accept the Big Bang model. A Brief History Of The Universe: Once the rate of expansion of the universe is accurately measured, it is possible to extrapolate back through time to the Big Bang. Astronomers currently estimate that the Big Bang occurred just under 14 billion years ago. They have also been able to suggest a rough outline of the history of the universe. Einstein’s Big Mistake: Einstein was working on his theory of general relativity, he realised that it suggested that the universe was expanding. He disliked the idea of an expanding universe so much that he added a ‘cosmological constant’ to his theory to ensure that it matched the steady state model. Einstein later described this as the ‘biggest blunder’ (mistake) of his life. Recreating The Big Bang One of the challenges faced by cosmologists is that many of the objects and processes they study are very different from those found in a standard laboratory. The conditions in the centre of a star or in the early moments of the Big Bang involve extremes of pressure and temperatures that are hard to physically recreate on Earth. Large Hadron Collider or LHC consists of a ring-shaped tunnel 27 km long buried beneath the border between France and Switzerland. LATC Questions: 1. State the location of the LHC: It’s buried beneath the border between France and Switzerland. 2. Describe the purpose of the LHC: To help scientists understand the big bang and discover a single model that will unite quantum mechanics and general relativity. 3. Describe how the LHC attempts to reproduce the conditions of the Big Bang: The LHC accelerates subatomic particles to very high speeds and makes them collide with each other. 4. Explain how particles are accelerated and made to travel around the LHC: Particles are accelerated using magnetic fields. 9.1 - Crime Scene Arson: Deliberate lighting of a fire. Ballistics: The study of guns and ammunition Circumstantial Evidence: Evidence that points to a suspect but doesn’t prove they are guilty. Core Body Temperature: 37°C for a healthy human. Corpse: Dead body. DNA: Deoxyribonucleic acid. Crime Scene Unit (CSU): Specialists who collect and bag evidence. Fibres: Strands of material such as hair, wool or polyester. Fingerprints: Patterns of ridges and grooves on the fingers and toes. Fluoresce: Shining due to special light (e.g. UV). Homicide: Murder or manslaughter of another human. Impressions: Track marks. Positive Impression: An exact image, such as the pattern of a shoe or a tyre Negative Impression: Formed by material gathering in recesses such as the grooves in a tyre/ shoe. Non-Porous: Doesn’t absorb liquids such as body oils. Pathology: Study of disease and cause of death. Physical Evidence: Large pieces of evidence. Porous: Absorbs body fluid such as oils. Tool Mark: Characteristic mark left by a tool. Toxicology: Study of the effects of poisons and chemicals on the body. Trace Evidence: Microscopic evidence such as fibres or DNA. Forensic Science: Meaning: Applying scientific methods and processes to solving crimes. Forensic science may prove the evidence, perpetrator or connection to a crime through the: o Examination of physical evidence. o Interpretation of data. o Administration of tests. o Clear and concise repairing o Truthful testimony of a forensic scientist. Crime Scene: Crime scenes are isolated to prevent the contamination of the site. Crime ranges from shoplifting, theft and forgery to assault and murder. All these crimes leave clues that point to those most likely to have committed the crime. The analysis of these clues is the work of forensic scientists. The information that they obtain from these clues is then used against suspects in court. o Sometimes clues are used to defend suspects instead. Forensic Scientists: Include forensic professionals working in law enforcement government, or private forensic laboratories who are responsible for dealing with any number of specific First On The Scene: The first authorities to arrive at a crime scene will usually be the police. They will: o Quickly cordon off (rope off ) the crime scene. o Call for back-up from other police, detectives, crime scene units, ambulance and fire brigade o Start interviewing eyewitnesses (people who were there). Crime Scene Unit (CSU): a team of specialist police, photographers and forensic scientists trained in collecting and analysing evidence. Does not deal with less serious crimes (e.g. burglary) but concentrates on more complex and serious crimes such as homicide (where another human is killed), terrorist attacks, arson (when a fire is started deliberately) or sexual assault. Every piece of evidence from a crime scene is a clue, and the CSU thoroughly searches the area to ensure that nothing is missed. Every piece of evidence is then marked and photographed while in position. Three different ways/methods the CSU use to ensure that the crime scene is thoroughly searched: zone Crime scene is then cleared and everything is sent away to be analysed by forensic scientists specialised in particular fields, such as pathology (the study of disease and cause of death), ballistics (the study of guns and bullets), toxicology (the study of the effects of poisons and chemicals on the body), fingerprints, fibres, chemistry and dentistry. The materials found at a crime scene can be classified broadly as either physical evidence or trace evidence. Ballistics: Study of guns and bullets. Ballistic evidence helps explain what: o Type of firearm was used. o Number of bullets. o Caliber and bullet. o Where the shooting was located. o Whether a weapon was fired. o If the firearm was used in previous crime scene/s. Physical Evidence: Anything that is reasonably large and easy to see. It includes: o Dead bodies. o Tool marks. o Fingerprints. o Weapons. o Tracks. o Blood spatters. o Damaged furniture. o Bullets and bullet holes. Corpses: Dead body. The identity of a corpse will need to be determined, so the CSU records or collects anything on or around the body that might help in its identification. Includes personal items such as wallets or purses, which might contain driver’s licence, Medicare card and credit cards. Mobile phones are likely to provide a list of friends and family, and computers, smartphones and tablets can provide the email and the social media accounts of their user. If the crime scene is a home or apartment, then family photos and telephone, gas and electricity bills all provide further information about the person who lived there. Evidence Of Time Of Death: A series of changes in a corpse begins immediately after death and can be used to determine how long a person has been dead. This information is vital for investigators since it will confirm the day (and possibly the hour) the crime was committed. This then allows police to narrow their range of suspects of who committed the murder. One obvious change is body temperature. o A healthy living human has a core body temperature of 37°C. After death, this falls at a rate of about 0.8°C every hour until it reaches room temperature — the exact rate of cooling depends on the victim’s clothing and the surrounding temperature. o This means that a body usually cools completely within one day. Other changes that can be used to determine the time of death are the: o Extent of rigor mortis (stiffening of muscles) o Colour of the skin ▪ Skin takes on a greenish appearance within two days of death because bacteria are starting to decompose the body. o Extent of decomposition o Type and life-cycle stage of insects present on and around the body Tool Marks And Track Impressions Tool Marks: Every type of tool leaves its own characteristic impression or tool mark. o The jemmy bar being used will leave scratches, dents and cuts in the window frame and sill. Different marks would have been left by a screwdriver, hammer, axe, knife or chisel. o The marks on a window frame allow investigators to determine whether a screwdriver or a jemmy bar was used to lever open a window. Tool marks depend on: o The type of tool being used o Whether the material being marked is hard (such as wood or bone) or soft (such as fabric, paper, skin, fat or muscle) o The sharpness of the individual tool. ▪ A sharp saw will produce a fine, crisp cut in wood, while a blunt one will rip the wood and produce a ragged edge. o Faults in the tool, such as missing teeth or heavy wear patterns. These are photographed and compared with tools collected from the suspect or the suspect’s residence. Track Impressions: Footprints and impressions from shoes and tyres are often left behind at a crime scene. Leave 2 types of impressions: o Positive Impression: ▪ Image that is exactly the same as the pattern on the shoe or tyre. ▪ Left on hard surfaces by feet, shoes and tyres that are dirty or soaked with water, oil or blood. o Negative impression: ▪ Formed if the suspect crossed damp sand, mud, snow, grease or congealing (thickening) blood. ▪ This is because the material only gathers in gaps in the tread of the shoes or tyres. ▪ Will have wet plaster poured into them to form a mould that can be taken back to the laboratory for analysis. All impressions are photographed next to a ruler to indicate their actual size. Example of track impression being used: o -The make and style of shoe that a criminal wore can be determined from its prints. o -Even more information comes from cuts, stones, scuffing and wear on sole. Collecting Fingerprints: In the grooves of your fingerprints are glands that secrete (release) sweat, oils and amino acids. A little of these secretions is left behind as an image of the fingerprint whenever something is touched or picked up. Non-porous materials (e.g. metal and glass) do not absorb sweat, oil or amino acids and so clear fingerprints are most likely to be found on: o Metallic objects such as doorknobs, forks, saucepans, knives and guns. o Glass and ceramics such as drinking glasses, windows, mirrors, bathroom tiles, cups, mugs and plates. o Plastics that make up light switches, car dashboards, steering wheels and broom and brush handles. o Painted surfaces such as cars and front doors and their surrounds. o Polished or varnished surfaces of furniture and stair railings fingerprints are revealed by brushing special types of powder onto the surface so that it sticks to the oils left behind. ▪ Black carbon powder is dusted onto light-coloured and clear surfaces and white aluminium powder is brushed onto dark surfaces to reveal fingerprint on non-porous materials. Porous materials (e.g. fabrics and raw timber) absorb body fluids, making it difficult to find fingerprints on them. However, fingerprints can still be revealed by brushing them with chemicals that react with the secretions of the fingerprint o Ninhydrin is a chemical that reacts with amino acids, turning the fingerprint a bluish purple. Light can also help to reveal fingerprints. Superglue reacts with the oils that make up fingerprints and superglue vapour is sometimes blown onto prints to make them stickier and more visible. Sealed objects such as fridges and cars can be filled with superglue vapour, revealing all the prints inside them. Blood Spatters: Drops of blood tell investigators a lot about what happened at a crime scene. Blood spatters change shape and size depending on the height and direction from which they fall. Blood that has been wiped up or washed from surfaces or clothing can be revealed using ultraviolet (UV) light. Blood spatters change shape depending on the direction they hit a surface. Trace evidence: Microscopic materials found at the crime scene. Incredibly useful to investigators since the criminal cannot see it and so cannot collect it or wipe it away. Fibres: Strands of natural materials such as hair, fur, fluff, wool and cotton, and synthetics such as nylon and polyester. Wherever you go, a few hairs are likely to fall from your head and body and a few threads are likely to drop from your clothes. These get trapped in the carpet, on furniture and on other people’s clothes. A violent struggle will cause even more fibres to drop. Investigators can collect these fibres by picking them up with sticky tape or by using a specialised vacuum cleaner. A microscope is then used to compare fibres with those from other crime scenes and those of the victim and suspects. Fibres can be identified from their characteristic surfaces. Fibres do not prove that a particular person was at the crime scene. Many people have the same shirt, jeans or dress and the same colour and type of hair. For this reason, fibres are only circumstantial evidence— they point to a suspect but cannot be used as proof that the person was there. Seeds: Microscopic grains of pollen and seeds found at a crime scene can be compared with plants nearby and in the areas in which the suspects live. This helps investigators to piece together what happened. o A body that has been shifted might have seeds from another area on it. DNA: Wherever you go, you leave a little bit of yourself behind. It might be a few cells of skin, a smear of body oil, sweat, a strand of hair, a few flakes of dandruff or saliva. o The saliva on a cigarette stub will contain the DNA of whoever smoked it from the saliva. DNA (deoxyribonucleic acid) is a chemical present in the nucleus of body cells. Everyone has different DNA, making DNA the ‘ultimate fingerprint’. Some of the DNA found at a crime scene will come from the victim, some may come from people known to be innocent and some will probably come from the suspect. This proves that the suspect was there at some time. 9.2 - Victims And Suspects Alpha Rays: Form of nuclear radiation. Anthropometry: Study of body size and proportions. Arches: A form of fingerprint in the shape of arches. Autopsy: Systematic dissection of a body. Autoradiogram: Identifying pattern that represents DNA patterning. Biometric Facial Recognition: Process in which a face is scanned and computer-matched with stored images of faces. CCTV: Closed circuit TV. Dioxins: Type of poison. Cold Case: A case that has been put on hold because of a lack of evidence. Composite: Form of fingerprint that shows two or more forms of fingerprint. Coroner: Judge who oversees inquiries into the death of people in disastrous or suspicious circumstances. Diatoms: Microscopic organisms found in water, each with a different shape. Gel Electrophoresis: Process in which DNA is cut then analysed to produce an autoradiogram. Identikit: Form of identification in which facial features are slotted together. Loops: A form of fingerprint in the shape of loops. Mitochondrial DNA (mtDNA): Small amount of DNA found in mitochondria, which are inherited only from the mother. Pathologist: Doctor who specialises in the study and diagnosis of diseases. Polonium-210: Radioactive material, used once as a poison. Reconciliation: Comparison of information from autopsy with data obtained about the likely victim. Retroactive Interference: Changes in the memory of an eyewitness after looking at other images. Ricin: A type of poison. Whorls: A form of fingerprint in the shape of whorls. Identifying Victims: Any corpse found at a crime scene needs to be identified. o Bodies found after natural disasters such as bushfires, floods and earthquakes, and accidents from cars, boats and aircraft. A corpse will be sent from a crime scene to the morgue or coroner’s office where identification will take place. Identification involves three stages: o Collection of ante-mortem data o Autopsy or post-mortem examination o Reconciliation. Ante-Mortem Data: Ante-mortem means ‘before death’ so any information relevant to the person before they died is ante-mortem data. This data includes the person’s sex, age, ethnicity, height, medical and dental records, fingerprints, recent photos, tattoos, piercings, scars and samples of their DNA. A corpse is easy to identify if an ID card, a driver’s licence, credit cards or a Medicare card are found on or near it. Photos of the person can be matched with the body and card numbers can be checked against home addresses. A mobile phone will hold phone numbers of friends and relatives who can be called. They can then view the body and confirm its identity. Even without these forms of identification, police can be reasonably certain about who the person was. o A body found in a burnt-out house is most likely to be someone who lived there. o The body in the driver’s seat of a badly damaged car was probably the registered owner or someone closely related to them. ▪ Hire cars are even easier to track since the driver’s name and address will have been left at the hire-car company. Autopsy: If there is doubt about the identity of a corpse or the way they died, then the coroner will instruct that an autopsy be performed. An autopsy is a systematic dissection of a corpse. The main steps in an autopsy: The coroner is a judge who is in charge of inquiries into the death of people in accidents or suspicious circumstances. An autopsy is performed by a pathologist, a doctor who specialises in the study and diagnosis of diseases. If the body is relatively intact, then the pathologist: o Takes fingerprints and extracts DNA. o Inspects the body for identifying marks such as birthmarks, tattoos or scars. o Takes samples of clothing and jewellery or takes photos of them. o Determines whether injuries happened before death (ante-mortem), at death (peri-mortem) or after death (post-mortem). o Determines which injuries or disease led to death. From the information collected at the autopsy, the pathologist can construct a timeline of how and when certain injuries happened. o Any cut made before death will clot and partially or completely heal. o Its edges might be red and swollen and there may be pus, a sign of infection. There would be little or no evidence of fresh blood. If a cut was inflicted as the person died or just before (e.g. during an accident or assault) then there would be no chance for clotting, swelling, healing or infection to take place. o However, there would be clear signs of bleeding. ▪ The heart stops on death so any cut made after death would produce no blood. o In the same way, bruises made before death will spread and show signs of repair, changing colour as they heal. o Bruises happening at death have less chance to spread and no chance of changing colour. ▪ Bruises do not form at all after death. Reconciliation: Comparing information collected in the autopsy with with any ante-mortem data that has been collected. Reconciliation compares: o Fingerprints with those on police files or those found around the home or apartment of the person thought to be the victim. o Identifying marks with those seen on the likely victim in family photos or on the database of missing persons. o Clothing and jewellery with those worn by the likely victim when last seen alive. o DNA with the DNA collected from the clothing, toothbrush or hairbrush of the likely victim. ▪ If the person has been missing for a long time, then comparison might need to be with their direct relatives, who will have similar DNA. o DNA with Guthrie cards. ▪ These cards store small samples of blood taken 48 to 72 hours after babies are born. ▪ Most people born in Australia since 1970 have a Guthrie card and so there is a sample of their blood and DNA on file. Identifying Skeletons: DNA deteriorates with time, especially when exposed to intense heat. There might even be no tissue left on the skeleton to analyse on corpses that were not discovered for a long time. Investigators then compare the medical and dental records of the likely victim with the injuries and dental work found on the skeleton. o Badly broken bones commonly have pins, plates and screws inserted in them to help them repair. ▪ Finding these in a skeleton can confirm its identity, as can replacement hips or knees. o Teeth grow until about the age of 20, so their development can be used to determine the age of someone in that age group. o Jewellery, belt buckles and buttons left on the skeleton will also give an idea of the victim’s sex and age. o Styles change and so this will suggest roughly when the person died. If none of this if possible, then the age and sex of the corpse can be determined from the size and density of large bones such as the pelvis, skull and femur (the main bone of the leg). Cause Of Death: A forensic pathologist must determine whether death was natural or caused by someone or something else. Each cause of death such as shooting, stabbing and drowning leaves its tell-tale signs. Shooting: Bullets may still be in a body or may have exited and lodged in furniture or walls nearby. This allows the path of the bullet to be determined. o -Shotguns shower a victim with multiple pellets. ▪ A single wound suggests that the shotgun was close while a scattering of wounds suggests that the victim was shot from a distance. o If there are burn marks from the exploding gunpowder, then this would indicate that the gun was shot from close range. Wounds: Knives leave their own tool marks on the body. o A wound produced by a straight-edged knife is different from that caused by a knife with a serrated edge. The depth of the wound gives an idea of the force used in the attack, and its angle suggests the direction of the attack. ▪ Sometimes the angle will even suggest that it was not an attack at all but a horrible accident. Where the wounds are located also provides information about the attacker. o Wounds on the right side of the chest suggest that the attacker was left-handed. o Wounds on the right of the back suggest the attacker was right-handed. Bruises indicate that a blunt object was used as the weapon. o Skin bruises badly if punched, kicked or hit. o The skin won’t be pierced but it might split and bones underneath are likely to fracture. Drowning: Water will be in the lungs and stomach of a victim who has drowned. This water will contain diatoms → Small single-celled organisms that are partly made of silica, a very hard material that forms a variety of shapes. Each creek, lake or dam has different-shaped diatoms and so their shape can pinpoint the location of a drowning and whether the body was shifted after death. Diatoms come in an amazing variety of shapes. The characteristic shapes of diatoms can point to where a drowning occurred. Identifying suspects Photographs: Photographs are excellent for proving identity and are used in passports, driver’s licences and student identification cards. They are not as useful for identifying suspects for several reasons: o The only photos police have will be of those who have already been found guilty of previous crimes. ▪ First offenders won’t yet have their photos on file. o An eyewitness needs to sift through hundreds of photographs ▪ This exposure can lead to retroactive interference, a process in which eyewitnesses involuntarily merge images in their memory to form a ‘suspect' who looks nothing like the person who committed the crime. Identikit: Sketched portraits have long been used as a method of identification. At first these sketches were drawn by professional artists from descriptions from eyewitnesses. However, the quality of these portraits depended as much on the ability of the artist as it did on the accuracy of the eyewitness account. Identikit became widely used after 1959. It used sets of pre-drawn facial features (such as eyes, mouth and chin shape) that slotted together to form a portrait. Identikit had many advantages over artist-drawn portraits. It: o Allowed a realistic image to be constructed at any police station o Did not depend on the ability or availability of an artist o Allowed various combinations of features to be tried to trigger the memory of the eyewitness. Today, police use computerised versions of Identikit. The computer-composite images are fast, can be digitally enhanced to make them even more realistic and can be three-dimensional. Some are incredibly accurate. Computer software allows realistic images to be produced by dragging across different facial features from its database of images. o Australia was the first country to produce a coloured computerised version of Identikit called FACE allowed police to take laptop computers to the crime scene and immediately identify criminals. o FACE helped to identify the three men who had set off explosives in the 2002 terrorist bombing in Bali. Proving The Suspect Was There: For a prosecution to be successful, police need to be able to prove that a suspect was at the scene of a crime. This can be done in a number of ways, including: o Electronic tracking o DNA. o Fingerprints Electronic Tracking: Security cameras and CCTV (closed circuit TV) are now common in shopping centres, banks, train stations and airports, outside nightclubs and even in city streets and lanes. Video from these can prove that a suspect was at the scene at the time of the crime and what they were carrying. Biometric facial recognition technologies that allow a CCTV to scan a crowd and identify known offenders are now being trialled. Most of these techniques compare the positions of points on the face with those of known criminals. Biometric facial recognition involves scanning features and comparing them with those on a computer data base. How it is done: o A person’s face has been scanned and converted into a ‘net’ that the computer software can compare. o Fingerprint scanning is another form of biometric identification. When switched on, your mobile phone or smartphone can enable your phone company to locate you to within 10 to 50 metres. This allows police to track suspects (and victims) and gives an easy way of proving that the suspect was close to the scene at the time the crime happened. Photos or video of unlawful behaviour sometimes ends up on social networking sites. Once there, it can be used as evidence in court. o In 2009, police used a video posted on Facebook to convict a 20-year-old Victorian man of hoon (dangerous) driving. o His $21 000 Ford Falcon utility was confiscated, his licence was disqualified for 18 months and he was fined $2000. Fingerprints: No two sets of fingerprints from different people have ever been found to be the same. This makes fingerprints an excellent method of identifying suspects or proving that the suspect was at the scene of a crime. The patterns that fingerprints form can be classified as loops, whorls, arches or composites. Before computers, fingerprints were unlikely to be stored outside the state or country of the suspect. Also, manual comparison of fingerprints was difficult since it is slow and prone to mistakes. For these reasons, some criminals were able to move about undetected, committing crimes as they went. Computers have overcome most of these problems by storing, sharing, comparing and matching fingerprints across different states and countries. DNA Analysis: DNA can be extracted from even the tiniest samples of flesh, blood, hair, saliva or semen left at crime scene. o A knife used in an attack will contain the DNA of the victim in the blood on its blade and the DNA of their attacker in sweat and oils on its handle. Everyone's DNA is different and so finding a suspect's DNA at a crime scene is proof that they were there at some time. However, it does not suggest when they were there or for what reason. o Your genetic code is determined by the sequence of nitrogen-rich bases adenine (A), thymine (T), guanine (G), ad cytosine (C) along the DNA strand. o These bases form complementary base pairs A-T or G-C. o You have a unique genetic code so the order of bases and base pairs along your DNA is unique too. o Hence, a person can be identified by the order of these base pairs. This order can be determined from a sequence of bars called an autoradiogram. If the order of bars in 2 auto-radiograms is identical and that both samples came from the same person. An autoradiogram is produced by chopping up DNA an then separating its fragments → Process is called Gel Electrophoresis. The role of fast computers: Normally about 400 base pairs need to be compared to match a person’s DNA to a sample at a crime scene. Manual matching of the autoradiogram of a suspect against those on police files would be near-impossible due to the multitude of black bars on an autoradiogram and the number of files that would need to be checked. The development of fast computers has made this comparison quick and accurate. Computers allow the autoradiogram of DNA found at a crime scene to be compared with those on police databases from people convicted of other crimes, DNA from other crime scenes, from corpses and from missing-person files. Twins: It is even more difficult to match DNA if the suspect is an identical twin Identical twins initially have the same DNA as the fertilised egg split and formed them, giving them near-identical DNA. As identical twins develop, small differences in their DNA occur due to copying errors (mutations) as the DNA replicates. To find these differences, billions of base pairs need to be compared. This would clearly be impossible without fast computers. Identical twins do not have identical fingerprints. However, their fingerprints do share more similarities than found between non-twin brothers and sisters. Cold Cases: The quick comparison that computers bring also allows police to reopen cold cases. These are cases that stalled long ago because a lack of evidence. Before the mid 1980s, the technology for testing and comparing DNA did not exist. Hence, DNA could not be used in forensics. Usually, DNA is extracted from the nuclei of cells found in body tissue collected from a crime scene. However, this nuclear DNA deteriorates quickly, sometimes leaving none for analysis years later. When this has happened, DNA can be extracted from cell mitochondria instead. This mitochondrial DNA (mtDNA) is passed on only from the mother, in contrast to nuclear DNA which is inherited equally from both parents. mtDNA is less likely to deteriorate than nuclear DNA, allowing it to be extracted and matched regardless of the age or condition of the sample. DNA analysis has provided evidence to successfully convict criminals many years after their crime or proven some people to be innocent of the crimes they were jailed for, allowing them to be released, pardoned and financially compensated. Types Of Poisons Used For Murder Dioxins: Poisonous chemicals that are present in tiny quantities in cigarette smoke, plastics and some industrial wastes. Assassins used dioxins in an attempt to kill Victor Yushchenko while he was campaigning to become President of the Ukraine. Ricin: Poison extracted from castor beans that is about 200 times more deadly than cyanide. Its symptoms are similar to natural diseases. o -Vary depending on whether it has been eaten, injected or breathed in. There is no test that will confirm its presence and there is no known antidote (cure). Questions: 1. Define the term assassination: Arranged political murders. 2. Name the rays that polonium-210 emits: Alpha rays 3. Explain why Alexander Litvinenko’s exposure to polonium-210 could not have been an accident: Polonium emits alpha rays, a type of nuclear radiation that is easily blocked. If exposure was accidental, then his clothes and skin would have stopped it entering his body. 4. The initial symptoms of ricin, dioxin and polonium poisoning can look like simple food poisoning. Propose what advantages this gives an assassin: The early symptoms of these poisons are often similar to food poisoning, which can make it difficult to detect the true cause. This allows the assassin to remain undetected for longer, potentially making it more difficult to trace the crime back to them. 5. There are extremely strict controls on the release of dioxins from industry into the environment. Propose a reason why: Dioxins are highly toxic. Can cause serious health problems in humans → Cancer, birth defects, and damage to the immune system. Incredibly difficult to remove from the environment once released → Any accidental release could have long-lasting on animals and plants. 9.3 - Fakes And Extortions Binary Code: The way a computer stores information: Uses 0 and 1. Chromatography: The use of solvents to separate colours of inks and other materials. Counterfeit: Fake (usually refers to a banknote). Extortion: Threats and blackmail. Identity Fraud: Pretending to be someone else. Intaglio: Raised printing. Polymer Film: Plastic used to make banknotes. Criminals don’t always rob banks to get money. o Some produce fake banknotes that can pass for the real thing. o Some fake their own identity to obtain money by pretending to be someone else or to pass through security at international borders. o Others try and get money by threats. Forensics provides ways of detecting what and who is fake and who is making threats. False Identities: Knowing the true identity of a person is vital whenever they: o Cross an international border o Claim money from the government (e.g. Centrelink), institutions (e.g. banks, insurance companies) or individuals (e.g. inheritance from a will). o Gain access to high-security institutions (e.g. bank vaults, military installations and biochemical laboratories). o Gain access to sensitive files (e.g. those held by hospitals, state and federal police, espionage or spy organisations e.g. ASIO or ASIS). Identity fraud takes place when someone pretends to be someone else. It costs Australia billions of dollars each year and so increasingly complex methods are being used to stop it. Documents Proving Identity: Passports, driver’s licences and credit cards are easy ways of proving your identity. All these documents have features to ensure they are secure and cannot be used by anyone but their ‘owners’: o Passports once had photos pasted into them but this proved too easy to change or replace. Modern passports (and driver’s licences) contain scanned images that are difficult to alter. o Passports now have an electronic chip in their centre page that contains the name, sex, nationality, date, birth and a digital version of the passport-holder’s photograph. ▪ This chip is activated when scanned and its details can be checked against the passport’s front page. ▪ These ‘chipped’ passports are known as ePassports and Australia was one of the first countries in the world to introduce them The ‘chip’ symbol in Figure 9.3.1 but indicates that the document is an ePassport. o Modern credit and identity cards usually include features such as holograms that make them difficult to reproduce. ▪ Credit cards also include magnetic strips and chips that contain the customer’s details and a personal identification number (PIN). Signatures are easily forged but only the true owner of the card should know its PIN. Biometric Facial Recognition: Most people identify you by your face. Biometric facial recognition software allows a computer to do this too. It measures the position of your facial features and compares them with those of photographs on file. International airports around Australia and many overseas have special self-service gates (Smartgates) that photograph travellers and identify them with the digital photo on the chip in their ePassports. Fingerprint Scans: Most countries have increased their border security since the terrorist attacks of 11 September 2001 (aka ‘9/11’). o All visitors entering and leaving the USA via its international airports have their fingerprints scanned. o These are then checked against files of known terrorists. Scanned fingerprints and whole handprints are also increasingly being used to sign in and out of work and as a ‘key’ to access computers, secure facilities or bank accounts. Access is only gained if the match is positive. Iris And Retina Identification: The iris is the coloured ring of muscle in the eye that controls the size of the pupil. Its patterns and colouring are incredibly complex. Each person has unique iris patterns and each eye has a different pattern. For these reasons, the iris provides a far better method of identification than a fingerprint. It is also impossible to fake — the iris contains 266 identifiable features and constantly moves, whereas a fake eye remains static. The retina is at the rear of the eye and its pattern of blood vessels is even better than the iris as proof of identity. Although more difficult to obtain, retina scans are more accurate than iris scan. Forgery: Counterfeit (fake) banknotes are relatively rare because real notes include special features that make them difficult to reproduce. Special features are: Australia was the first to use polymer film (plastic) notes. They are harder to counterfeit and last four or five times longer than paper ones — while a paper $5 note had an average life of about 6 months, a plastic one lasts more than 3 years. Organised crime groups are usually the only ones with sufficient money to purchase the technology, materials and skills needed to produce realistic counterfeit banknotes. Realistic counterfeit banknotes are difficult to detect by shop assistants and bank tellers unless they know what to look for. It might be the feel of the paper used, its colours or its serial number. o Real banknotes all carry different serial numbers, whereas counterfeits usually have the same serial number. Unfortunately, these differences may only become apparent after many fake notes have already been passed off as real. Fake Documents And Extortion: Criminals sometimes use fake signatures and documents to gain access to inheritances and insurance policies and to commit business fraud. They sometimes also use extortion (threats and blackmail) to get what they want. Forensics can help to determine: o If a signature or document is real. o Who wrote a threatening letter or email or who posted offending material on the internet. Electronic Documents: Everything you do on a computer, laptop or tablet is recorded on its hard drive in binary code, using the numbers 0 and 1. When you delete a file, all you delete is the way you access it. The original coding and information is still there. o This means that every document, email, Twitter or Facebook entry will still be there, to be retrieved if and when needed by forensics investigators. Handwriting: Everyone’s handwriting has characteristic features that can be used to identify who wrote or signed a document. Some of these feature: Handwriting changes with a person’s age, but its basic features do not. o You can alter your handwriting but the way you construct each letter tends to remain the same. ▪ Different ways to construct the letter E: Papers And Inks: Papers differ in their texture, absorbencies, the presence of watermarks and weight (often referred to as grams per square metre or GSM). All these characteristics affect the way an ink spreads and is absorbed and the way each individual letter appears. Inks differ too. o Different black inks contain different combinations of colours: some black inks contain blue while others have brown in them. Chromatography uses a solvent such as water or alcohol to dissolve and separate an ink into its component pigments. In this way, the ink used to forge a signature or letter or to write or print a threat can be matched with a suspect’s pen or printer.