Cholinergic Antagonists PDF
Document Details
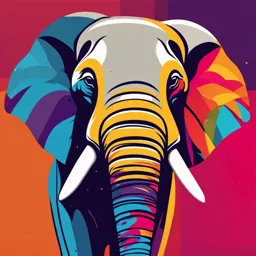
Uploaded by RadiantHyena
Tags
Summary
This document provides an overview of cholinergic antagonists, a category of medications. It examines their mechanisms and discusses their clinical uses. This resource covers topics about their effects, how they're used, and factors to consider.
Full Transcript
Pharmacology Autonomic Nervous System Cholinergic Antagonists Cholinergic Antagonists Content: Antimuscarinic Agents ………………………………………………………………....... 6 Ganglionic Blockers ……………………………………………………………………. 34 Neuromuscular Blocking Agents ……………………………………………………….... 38 Cholinergic Antagonists Overview: Choli...
Pharmacology Autonomic Nervous System Cholinergic Antagonists Cholinergic Antagonists Content: Antimuscarinic Agents ………………………………………………………………....... 6 Ganglionic Blockers ……………………………………………………………………. 34 Neuromuscular Blocking Agents ……………………………………………………….... 38 Cholinergic Antagonists Overview: Cholinergic antagonist is a general term for agents that bind to cholinoceptors (muscarinic or nicotinic) and prevent the effects of acetylcholine (ACh) and other cholinergic agonists. The most clinically useful of these agents are selective blockers of muscarinic receptors. They are commonly known as anticholinergic agents (a misnomer, as they antagonize only muscarinic receptors), antimuscarinic agents (more accurate terminology), or parasympatholytics. The effects of parasympathetic innervation are thus, interrupted by these agents, and the actions of sympathetic innervation are left unopposed. Cholinergic Antagonists Overview: A second group of drugs, the ganglionic blockers, shows a preference for nicotinic receptors of the sympathetic and parasympathetic ganglia. Clinically, they are the least important cholinergic antagonists. A third family of compounds, the neuromuscular blocking agents (mostly nicotinic antagonists), interfere with transmission of efferent impulses to skeletal muscles. These drugs are used as skeletal muscle relaxants in surgical anesthesia and as agents to facilitate intubation in surgical and critical care patients. Cholinergic Antagonists Figure 1 – Summary of selected cholinergic antagonists. Antimuscarinic Agents Antimuscarinic Agents Commonly known as anticholinergic drugs, these agents (for example, atropine and scopolamine) block muscarinic receptors (Figure 2), causing inhibition of muscarinic functions. In addition, these drugs block the few exceptional sympathetic neurons that are cholinergic, such as those innervating the salivary and sweat glands. Because they do not block nicotinic receptors, the anticholinergic drugs (more precisely, antimuscarinic drugs) have little or no action at skeletal neuromuscular junctions (NMJs) or autonomic ganglia. The anticholinergic drugs are beneficial in a variety of clinical situations. Note: A number of antihistamines and antidepressants (mainly tricyclic antidepressants) also have antimuscarinic activity. Antimuscarinic Agents Figure 2 – Sites of action of cholinergic antagonists. Antimuscarinic Agents 1. Atropine: Atropine is a tertiary amine extracted from belladonna alkaloid. It has a high affinity for muscarinic receptors and binds competitively to prevent ACh from binding (Figure 3). Atropine acts both centrally and peripherally. General actions last about 4 hours; however, effects of topical administration in the eye may persist for days. Neuroeffector organs have varying sensitivity to atropine. The greatest inhibitory effects are seen in bronchial tissue, salivary and sweat glands, and the heart. Antimuscarinic Agents 1. Atropine: Figure 3 – Competition of atropine and scopolamine with acetylcholine for the muscarinic receptor. Antimuscarinic Agents A. Atropine: 1. Actions: A. Eye: Atropine blocks muscarinic activity in the eye, resulting in mydriasis (dilation of the pupil), unresponsiveness to light, and cycloplegia (inability to focus for near vision). In patients with angle-closure glaucoma, intraocular pressure may rise dangerously. Antimuscarinic Agents A. Atropine: 1. Actions: B. Gastrointestinal (GI) Atropine (as the active isomer, L-hyoscyamine) can be used as an antispasmodic to reduce activity of the GI tract. Atropine and scopolamine are probably the most potent antispasmodic drugs available. Although gastric motility is reduced, hydrochloric acid production is not significantly affected. Thus, atropine is not effective for the treatment of ulcers. Doses of atropine that reduce spasms also reduce saliva secretion, ocular accommodation, and urination. These effects decrease compliance with atropine. Antimuscarinic Agents A. Atropine: 1. Actions: C. Cardiovascular: Atropine produces divergent effects on the cardiovascular system, depending on the dose (Figure 4). At low doses, the predominant effect is a slight decrease in heart rate. This effect results from blockade of M1 receptors on the inhibitory prejunctional (or presynaptic) neurons, thus permitting increased ACh release. Higher doses of atropine cause a progressive increase in heart rate by blocking M2 receptors on the sinoatrial node. Antimuscarinic Agents A. Atropine: Figure 4 – Dose-dependent effects of atropine. Antimuscarinic Agents A. Atropine: 1. Actions: D. Secretions: Atropine blocks muscarinic receptors in the salivary glands, producing dryness of the mouth (xerostomia). The salivary glands are exquisitely sensitive to atropine. Sweat and lacrimal glands are similarly affected. Note: Inhibition of secretions of sweat glands can cause elevated body temperature, which can be dangerous in children and the elderly. Antimuscarinic Agents A. Atropine: 2. Therapeutic Uses: A. Ophthalmic: Topical atropine exerts both mydriatic and cycloplegic effects, and it permits the measurement of refractive errors without interference by the accommodative capacity of the eye. Shorter-acting antimuscarinics (cyclopentolate and tropicamide) have largely replaced atropine due to prolonged mydriasis observed with atropine (7 to 14 days vs. 6 to 24 hours with other agents). Note: Phenylephrine or similar αadrenergic drugs are preferred for pupillary dilation if cycloplegia is not required. Antimuscarinic Agents A. Atropine: 2. Therapeutic Uses: B. Antispasmodic: Atropine is used as an antispasmodic agent to relax the GI tract. C. Cardiovascular: Injectable atropine is used to treat bradycardia of varying etiologies. D. Antisecretory: Atropine is sometimes used as an antisecretory agent to block secretions in the respiratory tract prior to surgery. Note: Glycopyrrolate is also used for this indication. Antimuscarinic Agents A. Atropine: 2. Therapeutic Uses: E. Antidote for cholinergic agonists: Atropine is used for the treatment of organophosphate (insecticides, nerve gases) poisoning, of overdose of clinically used anticholinesterases such as physostigmine, and in some types of mushroom poisoning (certain mushrooms contain cholinergic substances that block cholinesterases). Massive doses of injectable atropine may be required over a long period to counteract the poisons. The ability of atropine to enter the central nervous system (CNS) is of particular importance in treating central toxic effects of anticholinesterases. Antimuscarinic Agents A. Atropine: 3. Pharmacokinetics: Atropine is readily absorbed, partially metabolized by the liver, and eliminated primarily in urine. It has a half-life of about 4 hours. Antimuscarinic Agents A. Atropine: 4. Adverse Effects: Depending on the dose, atropine may cause dry mouth, blurred vision, “sandy eyes,” tachycardia, urinary retention, and constipation. Effects on the CNS include restlessness, confusion, hallucinations, and delirium, which may progress to depression, collapse of the circulatory and respiratory systems, and death. Low doses of cholinesterase inhibitors, such as physostigmine, may be used to overcome atropine toxicity. Atropine may also induce troublesome urinary retention. The drug may be dangerous in children, because they are sensitive to its effects, particularly to rapid increases in body temperature. Antimuscarinic Agents B. Scopolamine: Scopolamine, another tertiary amine plant alkaloid, produces peripheral effects similar to those of atropine. However, scopolamine has greater action on the CNS (unlike atropine, CNS effects are observed at therapeutic doses) and a longer duration of action as compared to atropine. It has some special actions as indicated below. Antimuscarinic Agents B. Scopolamine: 1. Actions: Scopolamine is one of the most effective drugs available for motion sickness (Figure 5). It also has the unusual effect of blocking short-term memory. In contrast to atropine, scopolamine produces sedation, but at higher doses, it can produce excitement. Scopolamine may produce euphoria and is susceptible to abuse. Antimuscarinic Agents B. Scopolamine: Figure 5 – Scopolamine is an effective agent for motion sickness. Antimuscarinic Agents B. Scopolamine: 2. Therapeutic Uses: Scopolamine is used for the prevention of motion sickness and postoperative nausea and vomiting. For motion sickness, it is available as a topical patch that provides effects for up to 3 days. Note: As with all drugs used for motion sickness, it is much more effective prophylactically than for treating motion sickness once it occurs. 3. Pharmacokinetics and Adverse Effects: These aspects are similar to those of atropine, with the exception of longer half-life. Antimuscarinic Agents C. Aclidinium, Glycopyrrolate, Ipratropium, and Tiotropium: Ipratropium and tiotropium are quaternary derivatives of atropine, and glycopyrrolate and aclidinium are synthetic quaternary compounds. Ipratropium is classified as a short-acting muscarinic antagonist (SAMA), while glycopyrrolate, tiotropium, and aclidinium are classified as long-acting muscarinic antagonists (LAMAs) based on the duration of action. These agents are approved as bronchodilators for maintenance treatment of bronchospasm associated with chronic obstructive pulmonary disease (COPD). Antimuscarinic Agents C. Aclidinium, Glycopyrrolate, Ipratropium, and Tiotropium: Ipratropium and tiotropium are also used in the acute management of bronchospasm in asthma and chronic management of asthma, respectively. All of these agents are delivered via inhalation. Because of the positive charge, these drugs do not enter the systemic circulation or the CNS, restricting effects to the pulmonary system. Antimuscarinic Agents D. Tropicamide and Cyclopentolate: These agents are used as ophthalmic solutions for mydriasis and cycloplegia. Their duration of action is shorter than that of atropine. Tropicamide produces mydriasis for 6 hours and cyclopentolate for 24 hours. D. Benztropine and Trihexyphenidyl: Benztropine and trihexyphenidyl are useful as adjuncts with other antiparkinson agents to treat Parkinson disease and other types of parkinsonian syndromes, including antipsychotic-induced extrapyramidal symptoms. Antimuscarinic Agents F. Oxybutynin and other Antimuscarinic Agents for Overactive Bladder: Oxybutynin, darifenacin, fesoterodine, solifenacin, tolterodine, and trospium are synthetic atropine-like drugs with antimuscarinic actions. 1. Actions: By competitively blocking muscarinic (M3) receptors in the bladder, intravesical pressure is lowered, bladder capacity is increased, and the frequency of bladder contractions is reduced. Antimuscarinic actions at M3 receptors in the GI tract, salivary glands, CNS, and eye may cause adverse effects. Darifenacin and solifenacin are relatively more selective M3 muscarinic receptor antagonists; however, the other drugs are mainly nonselective muscarinic antagonists, and binding to other muscarinic receptor subtypes may contribute to adverse effects. Antimuscarinic Agents F. Oxybutynin and other Antimuscarinic Agents for Overactive Bladder: 2. Therapeutic Uses: These agents are used for management of overactive bladder and urinary incontinence. Oxybutynin is also used in patients with neurogenic bladder. Antimuscarinic Agents F. Oxybutynin and other Antimuscarinic Agents for Overactive Bladder: 3. Pharmacokinetics: All of the agents are available in oral dosage forms. Most agents have a long half-life, which allows once-daily administration. Note: Immediate-release oxybutynin and tolterodine must be dosed two or more times daily; however, extended-release formulations of these agents allow for once-daily dosing. Oxybutynin is also available in a transdermal patch and topical gel formulation. These drugs are hepatically metabolized by the cytochrome P450 system (primarily CYP 3A4 and 2D6), with the exception of trospium, which is thought to undergo ester hydrolysis. Antimuscarinic Agents F. Oxybutynin and other Antimuscarinic Agents for Overactive Bladder: 4. Adverse Effects: Side effects include dry mouth, constipation, and blurred vision, which limit tolerability of these agents. Extended-release formulations and the transdermal patch have a lower incidence of adverse effects and may be better tolerated. Trospium is a quaternary compound that minimally crosses the blood–brain barrier and has fewer CNS effects than do other agents, making it a preferred choice in treating overactive bladder in patients with dementia. Important characteristics of the muscarinic antagonists are summarized in Figures 6 and 7. Antimuscarinic Agents Figure 6 – Adverse effects commonly observed with muscarinic antagonists. Antimuscarinic Agents Figure 7 – Summary of cholinergic antagonists. *Contraindicated in angle-closure glaucoma. GI = gastrointestinal; COPD = chronic obstructive pulmonary disease. Ganglionic Blockers Ganglionic Blockers Ganglionic blockers specifically act on the nicotinic receptors of both parasympathetic and sympathetic autonomic ganglia. Some also block the ion channels of the autonomic ganglia. These drugs show no selectivity toward the parasympathetic or sympathetic ganglia and are not effective as neuromuscular antagonists. Thus, these drugs block the entire output of the autonomic nervous system at the nicotinic receptor. Except for nicotine, the other drugs mentioned in this category are nondepolarizing, competitive antagonists. The responses of the nondepolarizing blockers are complex and mostly unpredictable. Therefore, ganglionic blockade is rarely used therapeutically, but often serves as a tool in experimental pharmacology. Ganglionic Blockers A. Nicotine: A component of cigarette smoke, nicotine is a poison with many undesirable actions. It is without therapeutic benefit and is deleterious to health. Depending on the dose, nicotine depolarizes autonomic ganglia, resulting first in stimulation and then in paralysis of all ganglia. The stimulatory effects are complex and result from increased release of neurotransmitters (Figure 8), due to effects on both sympathetic and parasympathetic ganglia. Ganglionic Blockers Figure 8 – Neurochemical effects of nicotine. GABA = γ-aminobutyric acid. Neuromuscular Blocking Agents Neuromuscular Blocking Agents These drugs block cholinergic transmission between motor nerve endings and the nicotinic receptors on skeletal muscle. They possess some chemical similarities to ACh and act either as antagonists (nondepolarizing) or as agonists (depolarizing) at the receptors on the endplate of the NMJ. Neuromuscular blockers (NMBs) are clinically useful to facilitate rapid intubation when needed due to respiratory failure (rapid sequence intubation). Neuromuscular Blocking Agents During surgery, they are used to facilitate endotracheal intubation and provide complete muscle relaxation at lower anesthetic doses. This increases the safety of anesthesia by allowing patients to recover quickly and completely. NMBs should not substitute for inadequate anesthesia. NMBs are also used in the intensive care unit (ICU) as adjuvant therapy to facilitate intubation and mechanical ventilation in critically ill patients. The first known NMB was curare, which Amazon hunters used to paralyze prey. Neuromuscular Blocking Agents A. Nondepolarizing (Competitive) Blockers : The development of tubocurarine followed, but it has been replaced by agents with fewer adverse effects, such as cisatracurium, mivacurium, pancuronium, rocuronium, and vecuronium. 1. Mechanism of Action: A. At Low Dose: NMBs competitively block ACh at the nicotinic receptors (Figure 9). They compete with ACh at the receptor without stimulating it, thus preventing depolarization of the muscle cell membrane and inhibiting muscular contraction. Neuromuscular Blocking Agents Figure 9 – Mechanism of action of competitive neuromuscular blocking drugs. Neuromuscular Blocking Agents A. Nondepolarizing (Competitive) Blockers : 1. Mechanism of Action: A. At Low Dose: Their competitive action can be overcome by administration of cholinesterase inhibitors, such as neostigmine and edrophonium, which increase the concentration of ACh in the NMJ. Clinicians employ this strategy to shorten the duration of neuromuscular blockade. In addition, at low doses the muscle responds to direct electrical stimulation from a peripheral nerve stimulator to varying degrees, allowing for monitoring of the extent of neuromuscular blockade. Neuromuscular Blocking Agents A. Nondepolarizing (Competitive) Blockers : 1. Mechanism of Action: B. At High Dose: At Nondepolarizing agents can block the ion channels of the motor end plate. This leads to further weakening of neuromuscular transmission, reducing the ability of cholinesterase inhibitors to reverse the actions of the nondepolarizing blockers. With complete blockade, the muscle does not respond to direct electrical stimulation. Neuromuscular Blocking Agents A. Nondepolarizing (Competitive) Blockers : 2. Actions: Muscles have differing sensitivity to blockade by competitive agents. Small, rapidly contracting muscles of the face and eye are most susceptible and are paralyzed first, followed by the fingers, limbs, neck, and trunk muscles. Next, the intercostal muscles are affected and, lastly, the diaphragm. The muscles recover in the reverse manner. Note: Sugammadex is a selective relaxant-binding agent that terminates the action of both rocuronium and vecuronium and can be used to speed recovery. Neuromuscular Blocking Agents A. Nondepolarizing (Competitive) Blockers : 3. Pharmacokinetics: All NMBs are injected intravenously or occasionally intramuscularly. These agents possess two or more quaternary amines in their bulky ring structure that prevent absorption from the gut. They penetrate membranes very poorly and do not enter cells or cross the blood– brain barrier. Drug action is terminated in a variety of ways (Figure 10). Pancuronium is excreted unchanged in urine. Neuromuscular Blocking Agents Figure 10 – Pharmacokinetics of the neuromuscular blocking drugs. Cisatracurium undergoes organ-independent elimination. Mivacurium and succinylcholine are metabolized by plasma cholinesterase. Neuromuscular Blocking Agents A. Nondepolarizing (Competitive) Blockers : 3. Pharmacokinetics: Cisatracurium undergoes organ-independent metabolism (via Hofmann elimination) to laudanosine, which is further metabolized and renally excreted. The amino steroid drugs vecuronium and rocuronium are deacetylated in the liver and excreted unchanged in bile. Mivacurium is eliminated by plasma cholinesterase. The choice of agent depends on the desired onset and duration of muscle relaxation and the route of elimination. Characteristics of the neuromuscular-blocking drugs are shown in Figure 11. Neuromuscular Blocking Agents Figure 11 – Characteristics of neuromuscular blocking drugs. Neuromuscular Blocking Agents A. Nondepolarizing (Competitive) Blockers : 4. Adverse Effects: In general, these agents are safe with minimal side effects. Neuromuscular Blocking Agents A. Nondepolarizing (Competitive) Blockers : 5. Drug Interactions: A. Cholinesterase Inhibitors: Drugs such as neostigmine, physostigmine, pyridostigmine, and edrophonium can overcome the action of nondepolarizing NMBs. However, with increased dosage, cholinesterase inhibitors can cause a depolarizing block due to elevated ACh concentrations at the end plate membrane. If the NMB has entered the ion channel (is bound to the receptor), cholinesterase inhibitors are not as effective in overcoming blockade. Neuromuscular Blocking Agents A. Nondepolarizing (Competitive) Blockers : 5. Drug Interactions: B. Halogenated Hydrocarbon Anesthetics: Drugs such as desflurane act to enhance neuromuscular blockade by exerting a stabilizing action at the NMJ. These agents sensitize the NMJ to the effects of NMBs. C. Aminoglycoside Antibiotics: Drugs such as gentamicin and tobramycin inhibit ACh release from cholinergic nerves by competing with calcium ions. They synergize with competitive blockers, enhancing neuromuscular blockade. D. Calcium Channel Blockers: These agents may increase the neuromuscular blockade of competitive blockers. Neuromuscular Blocking Agents B. Depolarizing Agents: Depolarizing blocking agents work by depolarizing the plasma membrane of the muscle fiber, similar to the action of ACh. However, these agents are more resistant to degradation by acetylcholinesterase (AChE) and can more persistently depolarize the muscle fibers. Succinylcholine is the only depolarizing muscle relaxant in use today. Neuromuscular Blocking Agents B. Depolarizing Agents: 1. Mechanism of Action: Succinylcholine attaches to the nicotinic receptor and acts like ACh to depolarize the junction (Figure 12). Unlike ACh, which is instantly destroyed by AChE, the depolarizing agent persists at high concentrations in the synaptic cleft, remaining attached to the receptor for a longer time and providing sustained depolarization of the muscle cell. Note: The duration of action is dependent on diffusion from the motor end plate and hydrolysis by plasma cholinesterase (also called butyrylcholinesterase or pseudocholinesterase). Genetic variants in which plasma cholinesterase levels are low or absent lead to prolonged neuromuscular paralysis Neuromuscular Blocking Agents B. Depolarizing Agents: Figure 12 – Mechanism of action of depolarizing neuromuscular blocking drugs. Neuromuscular Blocking Agents B. Depolarizing Agents: 1. Mechanism of Action: The depolarizing agent first causes opening of the sodium channel associated with nicotinic receptors, which results in depolarization of the receptor (phase I). This leads to a transient twitching of the muscle (fasciculations). Continued binding of the depolarizing agent renders the receptor incapable of transmitting further impulses. With time, continuous depolarization gives way to gradual repolarization as the sodium channel closes or is blocked. This causes a resistance to depolarization (phase II) and flaccid paralysis. Neuromuscular Blocking Agents B. Depolarizing Agents: 2. Actions: As with the competitive blockers, the respiratory muscles are paralyzed last. Succinylcholine initially produces brief muscle fasciculations that cause muscle soreness. This may be prevented by administering a small dose of nondepolarizing NMB prior to succinylcholine. Normally, the duration of action of succinylcholine is extremely short, due to rapid hydrolysis by plasma cholinesterase. However, succinylcholine that reaches the NMJ is not metabolized, allowing the agent to bind to nicotinic receptors, and redistribution to plasma is necessary for metabolism. Neuromuscular Blocking Agents B. Depolarizing Agents: 3. Therapeutic Uses: Because of its rapid onset of action, succinylcholine is useful when rapid endotracheal intubation is required. It is also used during electroconvulsive shock treatment. 4. Pharmacokinetics: Succinylcholine is injected intravenously. Its brief duration of action results from redistribution and rapid hydrolysis by plasma cholinesterase. Drug effects rapidly disappear upon discontinuation. Neuromuscular Blocking Agents B. Depolarizing Agents: 5. Adverse Effects: A. Hyperthermia: Succinylcholine can potentially induce malignant hyperthermia in susceptible patients. B. Apnea: Administration of succinylcholine to a patient who is deficient in plasma cholinesterase or has an atypical form of the enzyme can lead to prolonged apnea due to paralysis of the diaphragm. The rapid release of potassium may also contribute to prolonged apnea in patients with electrolyte imbalances. In patients with electrolyte imbalances receiving digoxin or diuretics (such as heart failure patients) succinylcholine should be used cautiously or not at all. Neuromuscular Blocking Agents B. Depolarizing Agents: 5. Adverse Effects: C. Hyperkalemia: Succinylcholine increases potassium release from intracellular stores. This may be particularly dangerous in burn patients and patients with massive tissue damage in which potassium has been rapidly lost or in patients with renal failure.