Chapter 9 Starr-Taggart Biology PDF
Document Details
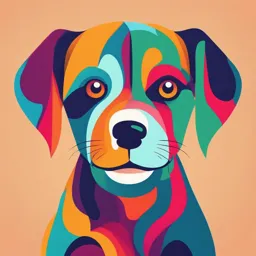
Uploaded by PurposefulForesight6010
Tags
Related
- 7. Transcription - From DNA to RNA PDF
- DMD5025/CHS5042 Nucleic Acids → Chromosomes → Genome PDF
- Lecture 24 Chapter 24 Genes & Chromosomes Chapter 25 DNA Metabolism Chapter 26 RNA Metabolism PDF
- Genetics/DNA/RNA PDF
- DNA/RNA & Genetics Study Guide PDF
- Molecular Biology Summary PDF - DNA, RNA, Genetics
Summary
This document provides an overview of the biological processes of DNA and RNA. It details processes such as transcription, which converts genetic information from DNA to RNA, and translation, where proteins are produced. The document discusses various types of molecules and their roles in these processes.
Full Transcript
9.1 The Aptly Acronymed RIPS Ricin is a naturally occurring protein that is highly toxic: A dose as small as a few grains of salt can kill an adult human, and there is no antidote. Ricin effectively deters beetles, birds, mammals, and other animals from eating seeds of the castor...
9.1 The Aptly Acronymed RIPS Ricin is a naturally occurring protein that is highly toxic: A dose as small as a few grains of salt can kill an adult human, and there is no antidote. Ricin effectively deters beetles, birds, mammals, and other animals from eating seeds of the castor-oil plant (Ricinus com- munis), which grows wild in tropical regions world- wide and is widely cultivated. Castor-oil seeds are the source of castor oil, an ingredient in plastics, cosmet- ics, paints, soaps, polishes, and many other items. After the oil is extracted from the seeds, the ricin is typically discarded along with the leftover seed pulp. The lethal effects of ricin were known as long ago as 1888, but using ricin as a weapon is now banned by most countries under the Geneva Protocol. However, controlling its production is impossible, because it takes no special skills or equipment to manufacture the toxin from easily obtained raw materials. Thus, ricin appears periodically in the news. For example, at the height of the Cold War, the Bulgarian writer Georgi Markov had defected to England and was working as a journalist for the BBC. As he made his way to a bus stop on a London street, an assassin used the tip of a modified umbrella to jam a small, ricin-laced ball into Markov's leg. Markov 'died in agony three days later. Plotted or thwarted terrorist activities involving ricin have made the news almost every year since then. Ricin is called a ribosome-inactivating protein (RIP) because it inactivates ribosomes, the organelles that assemble amino acids into proteins. RIPs occur in some bacteria, mushrooms, algae, and many plants (including food crops such as tomatoes, barley, and spinach). Many of these proteins are not particularly toxic because they do not cross intact cell membranes very well. Those that do, including ricin, have a domain that binds tightly to carbohydrates on plasma membranes (Figure 9.1). Binding causes the cell to take up the RIP by endocytosis. Once inside the cell, the second domain of the RIP-an enzyme-begins to inactivate ribosomes. One molecule of ricin can inacti- vate more than 1,000 ribosomes per minute. If enough ribosomes are affected, protein synthesis grinds to a halt. Proteins are critical to all life processes, so cells that cannot make them die very quickly. Someone who inhales ricin can die from low blood pressure and respiratory failure within a few days of exposure, but very few people actually encounter this toxin. Other RIPS are more prevalent. Shiga toxin, made by Shigella dysenteriae bacteria, causes dysentery. E. coli bacteria, including the strain O157:H7 (Section 4.1), make enterotoxin, an RIP that is the source of symptoms associated with food poisoning. A Ricin and castor-oil seeds. B Abrin and seeds of the rosary pea plant. C Cinnamomin and camphor tree seeds. D Enterotoxin and Escherichia coli. E Shiga toxin and Shigella dysenteriae. Figure 9.1 A few ribosome-inactivating proteins. The structure of RIPs are strikingly similar. One of their polypeptide chains (red) helps the molecule cross a cell's plasma membrane. The other chain (orange) destroys the cell's capacity for protein synthesis. 149 9.2 DNA, RNA, and Gene Expression Transcription converts information in a gene to RNA; transla- tion converts information in an mRNA to protein. Links to Proteins 3.6, Nucleic acids 3.8, Ribosomes 4.4, Enzymes 5.4, DNA sequence and replication 8.5 Converting a Gene to an RNA You learned in Chapter 8 that DNA carries genetic information. How does a cell convert that information into structural and functional components? Let's start with the nature of the information itself. DNA is like a book, an encyclopedia that contains all of the instructions for building a new individual. You already know the alphabet used to write the book: the four letters A, T, G, and C, for the four nucleotide bases adenine, thymine, guanine, and cytosine. Each strand of DNA consists of a chain of those four kinds of nucleotides. The linear order, or sequence, of the four bases in the strand is the genetic information. All of a cell's RNA and protein products are encoded by subsets of that DNA sequence called genes. base (guanine) 3 phosphate groups P P P CH2 A DNA nucleotide: guanine (G), or deoxyguanosine triphosphate 5' NH HC NH 2 3' 2 ' sugar (deoxyribos e) он A Guanine, one of the four nucleotides in DNA. The others (adenine, uracil, and cytosine) differ only in their component bases (blue). Three of the four bases in RNA nucleotides are identical to the bases in DNA nucleotides. 3 phosphate groups P-P P An RNA nucleotide: guanine (G), or guanosine triphosphate base (guanine) HC 5' -CH2 sugar (ribose) 3 ་ | OH OH NH NH2 B Compare the RNA nucleotide guanine. The only difference between the DNA and RNA versions of guanine (or adenine, or cytosine) is that RNA has a hydroxyl group at the 2' carbon of the sugar (shown in red). Figure 9.2 Comparing nucleotides of DNA and RNA. Converting the information encoded by a gene into a product starts with RNA synthesis, or transcription. By this process, enzymes use the nucleotide sequence of a gene as a template to synthesize a strand of RNA (ribonucleic acid): DNA transcription RNA RNA usually occurs in a single-stranded form that is structurally similar to a single strand of DNA. For example, both are chains of four kinds of nucleotides. Like a DNA nucleotide, an RNA nucleotide has three phosphate groups, a sugar, and one of four bases. However, DNA and RNA nucleotides are slightly dif- ferent (Figure 9.2). The two nucleic acids are named after their component sugars, ribose and deoxyribose, which differ in one functional group. Three of the bases (adenine, cytosine, and guanine) are the same in RNA and DNA nucleotides, but the fourth base in RNA is uracil, not thymine as it is in DNA (Figure 9.3). Despite these small differences in structure, DNA and RNA have very different functions. DNA's only role is to store a cell's heritable information. By con- trast, a cell transcribes several kinds of RNAs, and each kind has a different function. MicroRNAs are important in gene control, which is the subject of the next chapter. Three types of RNA have roles in protein synthesis. Ribosomal RNA (rRNA) is the main compo- nent of ribosomes, structures upon which polypeptide chains are built (Section 4.4). Transfer RNA (tRNA) delivers amino acids to ribosomes, one by one, in the order specified by a messenger RNA (mRNA). Converting mRNA to Protein Messenger RNA was named for its function as the "genetic messenger" between DNA and protein. A protein-building message is encoded within an mRNA's sequence by sets of three nucleotide bases, "genetic words" that follow one another along the length of the nucleotide strand. Like the words of a sentence, a series of these genetic words can form a meaningful parcel of information-in this case, the sequence of amino acids of a protein. By the process of translation, the protein-building information in an mRNA is decoded (translated) into a sequence of amino acids. The result is a polypeptide chain that twists and folds into a protein: mRNA translation → protein Sections 9.4 and 9.5 describe how rRNA and tRNA interact to translate the sequence of base triplets in an mRNA into the sequence of amino acids in a 150 UNIT || GENETICS adenine A NH2 HC guanine G HC N CH WH cytosine C NH2 HO HO thymine T CH3- HO NH NH 2 DNA deoxyribonucleic acid Nucleotide bases of DNA DNA has one function: It permanently stores a cell's genetic information, which is passed to offspring. Figure 9.3 DNA and RNA compared. sugar- phosphate backbone nucleotide base -base pair RNA ribonucleic acid adenine A NH2 RNAs have various functions. Some serve as disposable copies of DNA's genetic message; others are catalytic. Still others have roles in gene control. HO CH guanine G NH HC NH 2 cytosine C NH2 HO HO 0 uracil U =0 HO NH HO Nucleotide bases of RNA protein. Transcription and translation are steps in gene expression, the process by which genetic information encoded by a gene is converted into a structural or functional part of a cell or a body: transcription DNA mRNA translation protein gene DNA sequence that encodes an RNA or protein product. gene expression Process by which the information in a gene becomes converted to an RNA or protein product. messenger RNA (mRNA) A type of RNA that carries a protein-building message. ribosomal RNA (rRNA) A type of RNA that becomes part of ribosomes. transcription Process by which an RNA is assembled from nucleotides using the base sequence of a gene as a template. transfer RNA (tRNA) A type of RNA that delivers amino acids to a ribosome during translation. translation Process by which a polypeptide chain is assembled from amino acids in the order specified by an mRNA. A cell's DNA sequence contains all of the informa- tion it needs to make the molecules of life. Each gene encodes an RNA, and different types of RNAs interact to assemble proteins from amino acids (Section 3.6). Some of those proteins are enzymes that assemble lip- ids and complex carbohydrates from simple building blocks (Section 3.3), replicate DNA (Section 8.5), and make RNA (as you will see in the next section). Take-Home Message What is the nature of genetic information carried by DNA? » Genetic information occurs in genes, which are DNA sequences that encode instructions for building RNA or protein products. >> A cell transcribes the nucleotide sequence of a gene into RNA. » Although RNA is structurally similar to a single strand of DNA, the two types of molecules differ functionally. » A messenger RNA (mRNA) carries a protein-building code in its nucleotide sequence. rRNAs and tRNAs interact to translate that code into a protein. 9.3 Transcription: DNA to RNA RNA polymerase links RNA nucleotides into a chain, in the order dictated by the base sequence of a gene. A new RNA strand is complementary in sequence to the DNA strand from which it was transcribed. Links to Base pairing 8.4, DNA replication 8.5 Remember that DNA replication begins with one DNA double helix and ends with two DNA double helices (Section 8.5). The two double helices are identical to the parent molecule because base-pairing rules are RNA polymerase gene region promoter sequence in DNA The enzyme RNA polymerase binds to a promoter in the DNA. The binding positions the polymerase near a gene. Only the DNA strand complementary to the gene sequence will be translated into RNA. RNA DNA winding up DNA unwinding 2 The polymerase begins to move along the gene and unwind the DNA. As it does, it links RNA nucleotides in the order speci- fied by the base sequence of the complementary (noncoding) DNA strand. The DNA winds up again after the polymerase passes. The structure of the "opened" DNA at the transcription site is called a transcription bubble, after its appearance. 3' 5' followed during DNA replication. A nucleotide can be added to a growing strand of DNA only if it base-pairs with the corresponding nucleotide of the parent strand: G pairs with C, and A pairs with T (Section 8.4). Base- pairing rules also govern RNA synthesis during tran- scription. An RNA strand is structurally so similar to a DNA strand that the two can base-pair if their nucleo- tide sequences are complementary. In such hybrid mol- ecules, G pairs with C; A pairs with U (uracil). During transcription, a strand of DNA acts as a template upon which a strand of RNA-a transcript- is assembled from RNA nucleotides (Figure 9.4). A nucleotide can be added to a growing RNA only if it is complementary to the corresponding nucleotide of the parent DNA strand. Thus, each new RNA is comple- mentary in sequence to the DNA strand that served as its template. As in DNA replication, each nucleotide provides the energy for its own attachment to the end of a growing strand. Transcription is similar to DNA replication in that one strand of a nucleic acid serves as a template for synthesis of another. However, in contrast with DNA replication, only part of one DNA strand (not the whole molecule) serves as the template. The enzyme RNA polymerase, not DNA polymerase, adds nucleotides to the end of a growing transcript. Also, transcription produces a single strand of RNA; DNA replication produces two DNA double helices. Transcription begins when an RNA polymerase and regulatory proteins attach to a specific binding site in the DNA called a promoter. Binding positions the polymerase at a transcription start site close to the gene. Typically, only one of the two DNA strands in a double helix encodes a gene. The strand that is complementary to the gene sequence (the noncod- 5' U C C A C GA G A G 3 Zooming in on the transcription bubble, we can see that RNA polymerase covalently bonds successive nucleotides into an RNA strand. The new RNA is complementary to the noncoding strand of DNA, so it is an RNA copy of the gene. Figure 9.4 Animated Transcription. By this process, a strand of RNA is assembled from nucleo- tides according to a template: a gene region in DNA. Figure It Out: After the guanine, what is the next nucleotide that will be added to this growing strand of RNA? (9) en 150 LINIT II GENETICS C direction of transcription RNA transcripts Figure 9.5 Typically, many RNA polymerases simultaneously transcribe the same gene, producing a structure often called a "Christmas tree" after its shape. Here, three genes next to one another on the same chromosome are being transcribed. Figure It Out: Are the polymerases transcribing this DNA molecule moving from left to right or from right to left? DNA molecule ing strand) is the one that serves as the template for transcription. The polymerase starts moving along the DNA, in the 3' to 5' direction over the gene region As it moves, the polymerase unwinds the double helix just a bit so it can "read" the base sequence of the noncoding DNA strand. The polymerase joins free RNA nucleotides in the order dictated by that DNA sequence. As in DNA replication, the synthesis is directional: An RNA polymerase adds nucleotides only to the 3' end of the growing strand of RNA. When the polymerase reaches the end of the gene region, the DNA and the new RNA are released. RNA polymerase follows base-pairing rules, so the new RNA strand is complementary in base sequence to the DNA strand from which it was transcribed 3. It is an RNA copy of a gene, the same way that a paper transcript of a conversation format. Typically, many carries the same information in a differ- ent polymerases transcribe a particular gene region at the same time, so many new RNA strands can be produced very quickly (Figure 9.5). Post-Transcriptional Modifications In eukaryotes, transcription takes place in the nucleus. Eukaryotes also modify their RNA inside the nucleus, then ship it to the cytoplasm. Just as a dressmaker may snip off loose threads or add bows to a dress before it leaves the shop, so do eukaryotic cells tailor their RNA before it leaves the nucleus. For example, most eukaryotic genes contain inter- vening sequences called introns. Introns are removed alternative splicing RNA processing event in which some exons are removed or joined in alternate combinations. exon Nucleotide sequence that is not spliced out of RNA during processing. intron Nucleotide sequence that intervenes between exons and is excised during RNA processing. promoter In DNA, a sequence to which RNA polymerase binds. RNA polymerase Enzyme that carries out transcription. in chunks from a newly transcribed RNA before it leaves the nucleus. Sequences that stay in the RNA are exons (Figure 9.6). Exons can be rearranged and spliced together in different combinations. By such alternative splicing, one gene can encode different proteins. New transcripts that will become mRNAs are fur- ther tailored after splicing. A modified guanine "cap" gets attached to the 5' end of each. Later, the cap will help the mRNA bind to a ribosome. A tail of 50 to 300 adenines is also added to the 3′ end of a new mRNA; hence the name, poly-A tail. The tail is a signal that allows an mRNA to be exported from the nucleus. As you will see in Chapter 10, an mRNA's poly-A tail also helps regulate the timing and speed of its translation. DNA gene promoter exon intron exon intron exon transcription exon intron exon intron exon new transcript RNA processing exon exon exon finished RNA 5' 3' сар poly-A tail Figure 9.6 Animated Post-transcriptional modification of RNA. Introns are removed and exons spliced together. Messenger RNAs also get a poly-A tail and modified guanine "cap." Take-Home Message How is RNA assembled? >> In transcription, RNA polymerase uses the nucleotide sequence of a gene region in a chromosome as a template to assemble a strand of RNA. » The new strand of RNA is a copy of the gene from which it was transcribed. Post-transcriptional modification of RNA occurs in the nucleus of eukaryotes. CHAPTER 9 FROM DNA TO PROTEIN 153 9.4 RNA and the Genetic Code Base triplets in an mRNA encode a protein-building message. Ribosomal RNA and transfer RNA translate that message into a polypeptide chain. Links to Polypeptides 3.6, Ribosomes 4.4, Catalysis 5.4 DNA stores heritable information about proteins, but making those proteins requires messenger RNA (mRNA), transfer RNA (tRNA), and ribosomal RNA (rRNA). The three types of RNA interact to translate DNA's information into a protein. AUGULAUG not first base mRNA-The Messenger An mRNA is essentially a disposable copy of a gene. Its job is to carry DNA's protein-building informa- tion to the other two types of RNA for translation. second base third base C A G U บบบ UCU UAU UGU phe tyr cys UUC UCC UAC UGC ser UUA UCA UAA stop UGA stop leu UUG UCG UAG stop UGG trp G F E E D F E E D K E E P F E E D That protein-building information consists of a linear sequence of genetic "words" spelled with an alphabet of the four bases A, C, G, and U. Each of the genetic "words" carried by an mRNA is three bases long, and each is a code-a codon-for a particular amino acid. There are four possible bases in each of the three posi- tions of a codon, so there are a total of sixty-four (43) mRNA codons. Collectively, the sixty-four codons con- stitute the genetic code (Figure 9.7). The sequence of the three nucleotides in a base triplet determines which amino acid the codon specifies. For instance, the codon AUG codes for the amino acid methionine (met), and UGG codes for tryptophan (trp). One codon follows the next along the length of an mRNA, so the order of codons in an mRNA deter- mines the order of amino acids in the polypeptide that will be translated from it. Thus, the base sequence of a gene is transcribed into the base sequence of an mRNA, which is in turn translated into an amino acid sequence (Figure 9.8). Twenty naturally occurring amino acids are encoded by the sixty-four codons in the genetic code. Some amino acids are specified by more than one codon. For instance, GAA and GAG both code for glu- tamic acid. Other codons signal the beginning and end of a protein-coding sequence. For example, the first AUG in an mRNA is the signal to start translation in most species. AUG also happens to be the codon for methionine, so methionine is always the first amino acid in new polypeptides of such organisms. UAA, UAG, and UGA do not specify an amino acid. They CUU CCU CAU CGU his CUC CCC CAC CGC leu pro arg CUA CCA CAA CGA A gin ala alanine (A) leu leucine (L) CUG CCG CAG CGG G arg arginine (R) lys lysine (K) asn asparagine (N) met methionine (M) A AUU ACU AAU AGU asp aspartic acid (D) phe phenylalanine (F) asn ser cys cysteine (C) pro proline (P) AUC ile ACC AAC AGC thr glu glutamic acid (E) ser serine (S) AUA ACA AAA AGA gln glutamine (Q) thr threonine (T) A lys arg gly glycine (G) trp tryptophan (W) -AUG met ACG AAG AGG G his histidine (H) tyr tyrosine (Y) ile isoleucine (1) val valine (V) G GUU GCU GAU GGU asp GUC GCC GAC GGC val ala gly GUA GCA GAA GGA A glu GUG GCG GAG GGG G Figure 9.7 The genetic code. Each codon in mRNA is a set of three nucleotide bases. In the large chart, the left column lists a codon's first base, the top row lists the second, and the right col- umn lists the third. Sixty-one of the triplets encode amino acids; the remaining three are signals that stop translation. The amino acid names that correspond to abbreviations in the chart are listed above. Figure It Out: Which codons specify the amino acid lysine (lys)? pue JmSu 154 UNIT || GENETICS ATGACTCAG a gene region in DNA transcription codon codon codon mRNA AUGU A CUCAG UG translation methionine-tyrosine (met ) (tyr) serine (ser) amino acid sequence Figure 9.8 Example of the correspondence between DNA, RNA, and proteins. A DNA strand is transcribed into mRNA, and the codons of the mRNA specify a chain of amino acids. large subunit small subunit intact ribosome Figure 9.9 Animated Ribosome structure. An intact ribosome consists of a large and a small subunit. Protein components are shown in green. are signals that stop translation, so they are called stop codons. A stop codon marks the end of the protein- coding sequence in an mRNA. The genetic code is highly conserved, which means that most organisms use the same code and probably always have. Bacteria, archaea, and some protists have a few codons that differ from the typical code, as do mitochondria and chloroplasts—a clue that led to a theory of how these two organelles evolved (we return to this topic in Section 19.5). rRNA and tRNA-The Translators Ribosomes and tRNAs interact to translate the genetic code carried by an mRNA into a polypeptide. Both are abundant in cytoplasm. A ribosome has one large and one small subunit. Each subunit consists mainly of rRNA, with some associated structural proteins (Figure 9.9). During trans- lation, a large and a small ribosomal subunit converge as an intact ribosome on an mRNA. A tRNA has two attachment sites. The first is an anticodon, a triplet of nucleotides that base-pairs with an mRNA codon (Figure 9.10). The other attachment site binds to an amino acid--the one specified by the codon. Transfer RNAs with different anticodons carry different amino acids. You will see in the next section how those tRNAs deliver amino acids, one after the next, to a ribosome during translation of an mRNA. anticodon Set of three nucleotides in a tRNA; base-pairs with mRNA codon. codon In mRNA, a nucleotide base triplet that codes for an amino acid or stop signal during translation. genetic code Complete set of sixty-four mRNA codons. ACC anticodon D o g g trp amino acid attachment site A Icon and model of the tRNA that carries the amino acid tryptophan. Each tRNA's antico- don is complementary to an mRNA codon. Each also carries the amino acid specified by that codon. Pink balls are magnesium ions. Figure 9.10 tRNA structure. B During translation, tRNAs dock at an intact ribosome (only the small subunit is shown, in tan). The anticodons of two tRNAs have lined up with complementary codons of an mRNA (red). Ribosomal RNA is one example of RNA with enzymatic activity: The rRNA of a ribosome, not the protein, catalyzes the formation of a peptide bond between amino acids. As the amino acids are deliv- ered, the ribosome joins them via peptide bonds into a new polypeptide (Section 3.6). Thus, the order of codons in an mRNA-DNA's protein-building mes- sage becomes translated into a new protein. Take-Home Message What roles do mRNA, tRNA, and rRNA play during translation? » mRNA carries protein-building information. The bases in mRNA are "read" in sets of three during protein synthesis. Most of these base triplets (codons) code for amino acids. The genetic code consists of all sixty-four codons. >> Ribosomes, which consist of two subunits of rRNA and proteins, assemble amino acids into polypeptide chains. » A tRNA has an anticodon complementary to an mRNA codon, and it has a binding site for the amino acid specified by that codon. Transfer RNAs deliver amino acids to ribosomes. 9.5 Translation: RNA to Protein start codon (AUG) initiator tRNA · first amino acid-met of polypeptide peptide bond- Box Book UA CCA C met val met val met val leu D o n g rnet val leu Ribosome subunits and an initiator tRNA converge on an mRNA. A second tRNA binds to the second codon. 2 A peptide bond forms between the first two amino acids. 3 The first tRNA is released and the ribo- some moves to the next codon. A third tRNA binds to the third codon: A peptide bond forms between the second and third amino acids. 5 The second tRNA is released and the ribo- some moves to the next codon. A fourth tRNA binds the fourth codon. Translation converts the information carried by an mRNA into a new polypeptide chain. The order of codons in an mRNA determines the order of amino acids in the polypeptide chain translated from it. Links to Peptide bonds 3.6, Energy in metabolism 5.6 Translation, the second part of protein synthesis, pro- ceeds in three stages: initiation, elongation, and ter- mination. It occurs in the cytoplasm, which has many free amino acids, tRNAs, and ribosomal subunits. In eukaryotes, the initiation stage begins when an mRNA leaves the nucleus and a small ribosomal subunit binds to it (Figures 9.11 and 9.12A). Next, the anticodon of a special tRNA called an initiator base-pairs with the first AUG codon of the mRNA. Then, a large ribo- somal subunit joins the small subunit 0. In the elongation stage, the ribosome assembles a polypeptide chain as it moves along the mRNA. The initiator tRNA carries the amino acid methionine, so the first amino acid of the new polypeptide chain is methionine. Another tRNA brings the second amino acid to the complex as its anticodon base-pairs with the second codon in the mRNA. The ribosome cata- lyzes formation of a peptide bond between the first two amino acids The first tRNA is released and the ribosome moves to the next codon. Another tRNA brings the third amino acid to the complex as its anticodon base-pairs with the third codon of the mRNA 3. A peptide bond forms between the second and third amino acids The second tRNA is released and the ribosome moves to the next codon. Another tRNA brings the fourth amino acid to the complex as its anticodon base-pairs with the fourth codon of the mRNA S A peptide bond forms between the third and fourth amino acids 6. The new polypeptide chain continues to elongate as the ribosome catalyzes peptide bonds between amino acids delivered by successive tRNAs. Termination occurs when the ribosome reaches a stop codon in the mRNA. The mRNA and the poly- peptide detach from the ribosome, and the ribosomal subunits separate from each other. Translation is now complete. The new polypeptide will either join the pool of proteins in the cytoplasm, or enter rough ER of the endomembrane system (Section 4.7). *19999999A peptide bond forms met val leu gly between the third and fourth amino acids. The process repeats until the ribosome encounters a stop codon in the mRNA. Figure 9.11 Animated Translation. Translation initiates when ribo- somal subunits and an initiator tRNA converge on an mRNA. tRNAs deliver amino acids in the order dictated by successive codons in the mRNA. The ribosome links the amino acids together as it moves along the mRNA, so a polypeptide forms and elongates. Translation terminates when the ribosome reaches a stop codon. 156 UNIT II GENETICS Transcription mRNA polypeptide ribosome subunits Convergence of RNAs tRNA Translation polysomes A In eukaryotes, RNA transcribed in the nucleus moves into the cytoplasm through nuclear pores. Translation occurs in the cytoplasm. Ribosomes simultanously translating the same mRNA are called polysomes. Figure 9.12 Animated Overview of translation. In cells that are making a lot of protein, many ribo- somes may simultaneously translate the same mRNA, in which case they are called polysomes (Figure 9.12B). In bacteria and archaea, transcription and translation both occur in the cytoplasm, and these processes are closely linked in time and space. Translation begins before transcription ends, so a transcription "Christmas tree" is often decorated with polysome "balls." Given that many polypeptides can be translated from one mRNA, why would any cell also make many copies of an mRNA? Compared with DNA, RNA is not very stable. An mRNA may last only a few minutes before it is disassembled by enzymes in cytoplasm. The fast turnover allows cells to adjust their protein synthesis quickly in response to changing needs. mRNA polysomes newly forming polypeptide B Polypeptides elongat- ing from polysomes on an mRNA. Translation is energy intensive. That energy is pro- vided mainly in the form of phosphate-group transfers from the RNA nucleotide GTP (shown in Figure 9.2B) to molecules involved in the process. Take-Home Message How is mRNA translated into protein? » Translation is an energy-requiring process that converts protein-building information carried by an mRNA into a polypeptide. >> During initiation, an mRNA, an initiator tRNA, and two ribosome subunits join. » During elongation, amino acids are delivered to the complex by tRNAs in the order dictated by successive mRNA codons. As they arrive, the ribosome joins each to the end of the polypeptide chain. » Termination occurs when the ribosome reaches a stop codon in the mRNA. The mRNA and the polypeptide are released, and the ribosome disassembles. CHAPTER 9 FROM DNA TO PROTEIN 157 9.6 Mutated Genes and Their Protein Products A Hemoglobin, an oxygen-binding protein in red blood cells. This pro- tein consists of four polypeptides: two alpha globins (blue) and two beta globins (green). Each globin has a pocket that cradles a heme (red). Oxygen molecules bind to the iron atom at the center of each heme. 16 17 18 19 20 21 22 23 24 25 26 27 28 29 30 G GA CTCC C C C pro- CA GAGGAGA AG glu -glu lys GA CU ser B Part of the DNA (blue), mRNA (brown), and amino acid sequence (green) of human beta globin. Numbers indicate the position of the base pair in the coding sequence of the mRNA. G GA C A CC GU pro val CCCTCAGA GAGAAG MCM G GAGA AGUC glu lys- ser CA base-pair substitution replaces a thymine with an adenine. When the altered mRNA is translated, valine replaces glutamic acid as the sixth amino acid of the polypeptide. Hemoglobin with this form of beta globin is called HbS, or sickle hemoglobin.' CAGA C GGACCCT C CCUG GGA GAA G pro -gly arg- ser leu D A deletion of one base pair causes the reading frame for the rest of the mRNA to shift, so a different protein product forms. This frameshift results in a defective beta globin chain. The outcome is beta thalassemia, a genetic disorder in which a person has an abnormally low amount of hemoglobin. GGACTCCT TC pro U CCAG AAGGUC GAGGAGA AG glu glu lys val E An insertion of one base pair causes the reading frame for the rest of the mRNA to shift, so a different protein product forms. This frameshift results in a defective beta globin chain. The outcome is beta thalassemia. Figure 9.13 Animated Examples of mutations in the human beta globin gene. If the nucleotide sequence of a gene changes, it may result in an altered gene product, with harmful effects. Links to Hydrophobicity 2.5, Hemoglobin 3.2, Protein struc- ture 3.6, Free radicals and heme cofactors 5.6, Chromosomes 8.2, DNA replication 8.5, Mutations 8.6 Mutations, remember, are permanent changes in a DNA sequence (Section 8.6). A mutation in which one nucleotide and its partner are replaced by a different base pair is called a base-pair substitution. Other muta- tions involve the loss of one or more base pairs (a dele- tion) or the addition of extra base pairs (an insertion). Mutations are relatively uncommon events in a normal cell. For example, the chromosomes in a dip- loid human cell collectively consist of about 7 × 109 base pairs, any of which may become mutated each time that cell divides. However, only about 175 bases actually do change after a division. On top of that, only about 3 percent of the cell's DNA encodes pro- tein products, so there is a low probability that any of those mutations will be in a protein coding region. When a mutation does occur in a protein coding region, the redundancy of the genetic code offers the cell a margin of safety. For example, a mutation that changes a UCU codon to UCC in an mRNA may not have further effects, because both codons specify serine. Mutations in a gene that have no effect on the gene's product are said to be silent. Other mutations are not silent: They may change an amino acid in a protein, or result in a premature stop codon that short- ens it. Both outcomes can have severe consequences for the cell-and the organism. The oxygen-binding properties of hemoglobin (Section 3.2) provide an example of how a mutation can change a protein. As red blood cells circulate through the lungs, the hemoglobin proteins inside of them bind to oxygen molecules. The cells then travel to other regions of the body, and the hemoglo- bin releases its oxygen cargo wherever the oxygen level is low. When the red blood cells return to the lungs, the hemoglobin binds to more oxygen. Hemoglobin's structure allows it to bind and release oxygen. The protein consists of four polypep- tides called globins (Figure 9.13A). Each globin folds around a heme, a cofactor with an iron atom at its center (Section 5.6). Oxygen molecules bind to hemo- globin at those iron atoms. In adult humans, two alpha globin chains and two beta globin chains make up each hemoglobin mol- ecule. Defects in either of the polypeptide chains can cause a condition called anemia, in which a person's blood is deficient in red blood cells or in hemoglobin. 158 UNIT || GENETICS sickled cell glutamic acid valine A A base-pair substitution results in the abnormal beta globin chain of sickle hemo- globin (HbS). The sixth amino acid in such chains is valine, not glutamic acid. The dif- ference causes HbS molecules to form rod- shaped clumps that distort normally round blood cells into sickle shapes. Figure 9.14 Animated Sickle-cell anemia. normal cell B The sickled cells clog small blood vessels, causing circulatory problems that result in damage to many organs. Destruction of the cells by the body's immune system results in anemia. Tionne "T-Boz" Watkins of the music group TLC is a celebrity spokesperson for the Sickle Cell Disease Association of America. She was diagnosed with sickle-cell anemia as a child. Both outcomes limit the blood's ability to carry oxy- gen, and the resulting symptoms range from mild to life-threatening. Sickle-cell anemia, a type of anemia that is most common in people of African ancestry, arises because of a base-pair substitution in the beta globin gene. The substitution causes the body to produce a version of beta globin in which the sixth amino acid is valine instead of glutamic acid (Figure 9.13B,C). Hemoglobin with this mutation in its beta globin is called sickle hemoglobin, or HbS. Unlike glutamic acid, which carries a negative charge, valine carries no charge. As a result of that one substitution, a tiny patch of the beta globin polypep- tide changes from hydrophilic to hydrophobic, which in turn causes the hemoglobin's behavior to change slightly. HbS molecules stick together and form large, rodlike clumps under certain conditions. Red blood cells that contain the clumps become distorted into a crescent (sickle) shape (Figure 9.14). Sickled cells clog tiny blood vessels, thus disrupting blood circulation throughout the body. Over time, repeated episodes of sickling can damage organs and cause death. A different type of anemia, beta thalassemia, is caused by the deletion of the twentieth base pair in the coding region of the beta globin gene (Figure 9.13D). Like many other deletions, this one causes a frame- shift, in which the reading frame of the mRNA codons shifts. A frameshift usually has drastic consequences because it garbles the genetic message, just as incor- rectly grouping a series of letters garbles the meaning of a sentence: The cat ate the rat. T hec ata tet her at. Th eca tat eth era t. The frameshift caused by the beta globin deletion results in a polypeptide that differs drastically from normal beta globin. Hemoglobin molecules do not assemble correctly with the altered polypeptides, which are only 18 amino acids long (the normal beta globin chain has 147 amino acids). This outcome is the source of anemia in beta thalassemia. Beta thalassemia can also be caused by insertion mutations, which, like deletions, often cause frame- shifts (Figure 9.13E). Insertion mutations are often caused by the activity of transposable elements, which are segments of DNA that can move spontaneously within or between chromosomes. Transposable ele- ments can be hundreds or thousands of base pairs long, so when one interrupts a gene it becomes a major insertion that changes the gene's product. Transposable elements are common in the DNA of all species; about 45 percent of human DNA consists of transposable elements or their remnants. base-pair substitution Mutation in which a single base pair changes. deletion Mutation in which one or more base pairs are lost. frameshift Mutation that causes the reading frame of mRNA codons to shift. insertion Mutation in which one or more base pairs become inserted into DNA. transposable element Segment of chromosomal DNA that can spontaneously move to a new location. Take-Home Message What happens after a gene becomes mutated? » Mutations that result in an altered protein can have drastic consequences. » A base-pair substitution may change an amino acid in a protein, or shorten it by introducing a premature stop codon. » Frameshifts that occur after an insertion or deletion change an mRNA's codon reading frame, so they garble its protein-building instructions. CHAPTER 9 FROM DNA TO PROTEIN 159 The Aptly Acronymed RIPS (revisited) The enzyme in ricin inactivates ribosomes by remov- ing a particular adenine base from one of the rRNAs in the heavy subunit. That adenine is part of an RNA binding site for proteins that help with elongation. After the base has been removed, a ribosome can no longer bind to those proteins, and elongation stops. However, the main function of RIPs may not be destroying ribosomes. Many function in immune signaling, but it is their antiviral and anti- cancer activity that has researchers abuzz. Plants that make RIPs have been used as traditional medicines for many centuries, but only recently have Western scien- tists begun testing these compounds to combat HIV and cancer. How would you vote? Exposure to ricin is unlikely, but extremists continue to attempt using it for terrorist activities. Researchers have developed a vaccine against ricin. Do you want to be vaccinated? Summary Section 9.1 The ability to make proteins is critical to all life processes. Molecules such as ricin and other proteins that inac- tivate ribosomes (RIPs) can be extremely toxic if they enter cells. Section 9.2 DNA's genetic information is encoded within its base sequence. Genes are subunits of that sequence. A cell uses the information in a gene to make an RNA or protein product. The process of gene expression involves transcription of a DNA sequence to an RNA, and translation of the information in an mRNA, or messenger RNA, to a protein product. Translation requires the participation of tRNA (transfer RNA) and rRNA (ribosomal RNA). Section 9.3 During transcription, RNA polymerase binds to a promoter near a gene region of a chromosome. The poly- merase assembles a strand of RNA by linking RNA nucleotides in the order dictated by the base sequence of the gene. Thus, the new RNA is complementary to the gene from which it was transcribed. The RNA of eukaryotes is modified before it leaves. the nucleus. Introns are removed. With alternative splic- ing, some exons may be removed also, and the remain- ing ones spliced in different combinations. A cap poly-A tail are also added to a new mRNA. and a Section 9.4 mRNA carries DNA's protein- building information. The information consists of a series of codons, sets of three nucleotides. Sixty-four codons, most of which specify amino acids, constitute the genetic code. Each tRNA has an anticodon that can base-pair with a codon, and it binds to the amino acid specified by that codon. rRNA and proteins make up the two subunits of ribosomes. Section 9.5 Genetic information carried by an mRNA directs the synthesis of a polypeptide during translation. First, an mRNA, an initiator tRNA, and two ribosomal subunits converge. The intact ribosome then catalyzes formation of a peptide bond between successive amino acids, which are delivered by tRNAs in the order specified by the codons in the mRNA. Translation ends when the ribosome encounters a stop codon. Section 9.6 Insertions, deletions, and base-pair substitutions are mutations. The activity of transposable elements causes some mutations. A mutation that changes a gene's product may have harm- ful effects. Sickle-cell anemia, which is caused by a base- pair substitution in the gene for the beta globin chain of hemoglobin, is one example. Beta thalassemia is an out- come of a frameshift mutation in the beta globin gene. Self-Quiz Answers in Appendix III 1. A chromosome contains many different gene regions that are transcribed into different a. proteins b. polypeptides CRNAS d. a and b c. codon d. protein 2. A binding site for RNA polymerase is called a a. gene b. promoter 3. Energy that drives transcription is provided mainly by a. ATP b. RNA nucleotides 4. An RNA molecule is typically is typically c. GTP d. all are correct ; a DNA molecule a. single-stranded; double-stranded b. double-stranded; single-stranded c. both are single-stranded d. both are double-stranded 160 IINIT II GENETICS DATA ANALYSIS ACTIVITIES RIPs as Cancer Drugs Researchers are taking a page from the structure-function relationship of RIPs in their quest for cancer treatments. The most toxic RIPs, remember, have one domain that interferes with ribosomes, and another that carries them into cells. Melissa Cheung and her colleagues incorporated a peptide that binds to skin cancer cells into the enzymatic part of the E. coli entero- toxin RIP. The researchers created a new RIP that specifi- cally kills skin cancer cells, which are notoriously resistant to established therapies. Some of their results are shown in Figure 9.15. 1. Which cells had the greatest response to an increase in concentration of the engineered RIP? 2. At what concentration of RIP did all of the different kinds of cells survive? 3. Which cells survived best at 10-6 grams per liter RIP? 4. Why are some of the data points linked by straight lines? Cell Survival (%) 100- 50 0 10-7 10-6 10-5 Concentration of RIP (grams/liter) Figure 9.15 Effect of an engineered RIP on cancer cells. The model (left) shows the enzyme portion of E. coli enterotoxin that has been engineered to carry a small sequence of amino acids (in blue) that targets skin cancer cells. (Red indicates the active site.) The graph (right) shows the effect of this engineered RIP on human cancer cells of the skin (); breast (); liver (▲); and prostate (). Source: Cheung et al. Molecular Cancer, 9:28, 2010. 5. RNAs form by 6. ; proteins form by a. replication; translation b. translation; transcription c. transcription; translation d. replication; transcription a. 3 b. 64 different codons constitute the genetic code. 7. Most codons specify a(n) a. protein b. polypeptide 8. Anticodons pair with a. mRNA codons b. DNA codons c. 20 d. 120 c. amino acid d. mRNA c. RNA anticodons d. amino acids 9. What is the maximum length of a polypeptide encoded by an mRNA that is 45 nucleotides long? 10. a. Introns b. Exons are removed from new mRNA transcripts. c. Telomeres d. Amino acids 11. Where does transcription take place in a eukaryotic cell? a. the nucleus b. extracellular fluid c. the cytoplasm d. b and c are correct 12. Where does translation take place in a typical eukaryotic cell? a. the nucleus b. extracellular fluid c. the cytoplasm d.b and c are correct 13. Energy that drives translation is provided mainly by a. ATP b. amino acids c. GTP d. all are correct bases in d. 120 14. Each amino acid is specified by a set of an mRNA transcript. 15. a. 3 b. 20 are mutations. a. Transposable elements b. Base-pair substitutions c. Insertions c. 64 d. Deletions e. b, c, and d are correct f. all of the above a. coding region 16. Match the terms with the best description. genetic message promoter polysome exon genetic code intron transposable element Critical Thinking b. gets around c. read as base triplets d. removed before translation e. occurs only in groups f. complete set of 64 codons g. binding site for RNA polymerase 1. Antisense drugs help us fight some types of cancer and viral diseases. The drugs consist of short mRNA strands that are complementary in base sequence to mRNAs linked to the diseases. Speculate on how antisense drugs work. 2. An anticodon has the sequence GCG. What amino acid does this tRNA carry? What would be the effect of a muța- tion that changed the C of the anticodon to a G? 3. Each position of a codon can be occupied by one of four nucleotides. What is the minimum number of nucleotides per codon necessary to specify all 20 of the amino acids that are typical of eukaryotic proteins? 4. Using Figure 9.7, translate this nucleotide sequence into an amino acid sequence, starting at the first base: 5'-GGUUUCUUGAAGAGA-3′ 5. Translate the sequence of bases in the previous question, starting at the second base. 6. The termination of prokaryotic DNA transcription often depends on the structure of a newly form- ing RNA. Transcription stops where the mRNA folds back on itself, forming a hairpin-looped structure like the one shown at right. How do you think that this structure stops transcription? CCCAC A U- G A ఆ C 10 5 5 CHAPTER 9 FROM DNA TO PROTEIN 161 JUU...