Chapter 15.1 Consequences of Mutations PDF
Document Details
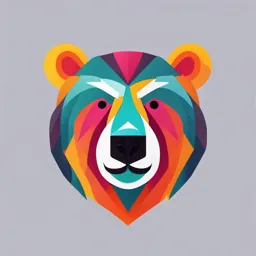
Uploaded by ClearerYew5263
University of Kansas
Tags
Related
- Molecular Biology and Genetics of Human Disease Summary PDF
- Molecular Biology and Genetics Exam January 2023 PDF
- Molecular Biology: DNA Repair Mechanisms (BIOL10221 2024)
- BIOL10221 2024 Molecular Biology Lecture Notes (Effects of Mutations) PDF
- BIOL10221 Molecular Biology Module 9 - Lecture 2 - Effects of Mutations II PDF
- Mutations: Molecular Biology and Diagnostics PDF
Summary
This document appears to be part of a larger biology textbook. It covers learning outcomes related to mutations and provides a basic overview of various types of mutations.
Full Transcript
15.1 Consequences of Mutations 12/11/24, 4:53 PM 15.1 Consequences of Mutations about:srcdoc Page 1 of 17 15.1 Consequences of Mutations 12/11/24, 4:53 PM Learning Outcomes: 1. List several ways that muta...
15.1 Consequences of Mutations 12/11/24, 4:53 PM 15.1 Consequences of Mutations about:srcdoc Page 1 of 17 15.1 Consequences of Mutations 12/11/24, 4:53 PM Learning Outcomes: 1. List several ways that mutations can alter the amino acid sequence of a polypeptide. 2. Describe examples of how mutations can cause human genetic diseases. 3. Explain how mutations that occur outside of the coding sequence can affect the expression of a gene. 4. Compare and contrast the effects of mutations in somatic cells versus germ- line cells. Page 312 How do mutations affect traits? To answer this question at the molecular level, we must understand how changes in the DNA sequence of a gene ultimately affect gene function. Most of our understanding of mutations has come from the study of experimental organisms, such as bacteria and Drosophila. Researchers can expose these organisms to agents that cause mutations and then study the consequences of the mutations that arise. In addition, because about:srcdoc Page 2 of 17 15.1 Consequences of Mutations 12/11/24, 4:53 PM these organisms have a short generation time, researchers can investigate the effects of mutations when they are passed from cell to cell and from parent to offspring. The structure and amount of genetic material can be altered in a variety of ways. For example, the structure and number of chromosomes can change. We will examine these types of genetic changes in Chapter 16. In this section, we will focus our attention on gene mutations, which are relatively small changes in the sequence of bases in a particular gene. We will also consider how the timing of new mutations during an organism’s development has important consequences. Gene Mutations May Alter the DNA Sequence of a Gene Mutations cause two basic types of changes to a gene: (1) The base sequence within a gene can be changed; and (2) one or more base pairs can be added to or removed from a gene. A point m utation affects only a single base pair within the DNA. For example, the DNA sequence shown here has been altered by a base substitution in which a T (in the top strand) has been replaced by a G and the corresponding A in the complementary strand is replaced with a C: about:srcdoc Page 3 of 17 15.1 Consequences of Mutations 12/11/24, 4:53 PM 5′–CCCGCTAGATA–3′ 5′–CCCGCGAGATA–3′ → 3′–GGGCGATCTAT–5′ 3′–GGGCGCTCTAT–5′ A point mutation could also involve the addition or deletion of a single base pair to a DNA sequence. For example, in the following sequence, a single base pair (A-T) has been added to the DNA: 5′–GGCGCTAGATC—3′ 5′–GGCAGCTAGATC–3′ → 3′–CCGCGATCTAG—5′ 3′–CCGTCGATCTAG–5′ Though point mutations may seem like small changes to a DNA sequence, they can have important consequences when genes are expressed, as we will see next. Mutation by Base Substitution about:srcdoc Page 4 of 17 15.1 Consequences of Mutations 12/11/24, 4:53 PM Gene Mutations May Affect the Amino Acid Sequence of a Polypeptide If a mutation occurs within the coding region of a protein-coding gene, the mutation may alter that sequence in a variety of ways. Table 15.1 considers the potential effects of point mutations. about:srcdoc Page 5 of 17 15.1 Consequences of Mutations 12/11/24, 4:53 PM Consequences of Point Mutations Within the Coding Sequence of a Protein- Table 15.1 Coding Gene Mutation in the DNA Effect on polypeptide Example* None None Base Silent—causes no substitution change Base Missense—changes one substitution amino acid in the polypeptide Base Nonsense—changes a substitution normal codon to a stop codon and shortens the polypeptide Addition of a Frameshift—produces a single base different amino acid sequence about:srcdoc Page 6 of 17 15.1 Consequences of Mutations 12/11/24, 4:53 PM Silent Mutations Silent mutations do not alter the amino acid sequence of the polypeptide, even though the nucleotide sequence has changed. As discussed in Chapter 12, the genetic code is degenerate; that is, more than one codon can specify the same amino acid. Silent mutations occur in the third base of many codons without changing the type of amino acid that is encoded. Missense Mutations A missense mutation is a base substitution that changes a single amino acid in a polypeptide sequence. A missense mutation doesn't always alter protein function, because it changes only a single amino acid within a polypeptide that is typically hundreds of amino acids in length. A missense mutation that substitutes an amino acid with a chemistry similar to the original amino acid is less likely to alter protein function. For example, a missense mutation that substitutes a glutamic acid for an aspartic acid may not alter protein function because both amino acids are negatively charged and have similar side chain structures. Alternatively, other missense mutations have a dramatic effect on protein function. These often involve more significant changes in amino acid structure, such as a change of a polar amino acid to a about:srcdoc Page 7 of 17 15.1 Consequences of Mutations 12/11/24, 4:53 PM nonpolar one. Nonsense Mutations A nonsense mutation involves a change from a normal codon to a stop, or termination, codon. This causes translation to be terminated earlier than expected, producing a truncated polypeptide (see Table 15.1). Compared with a normal polypeptide, a shorter polypeptide is much less likely to function properly. Frameshift Mutations Finally, a frameshift mutation involves the addition or deletion of a number of nucleotides that is not a multiple of three. For example, a frameshift mutation could involve the addition or deletion of one, two, four, or five nucleotides. Because the codons are read in multiples of three, these types of insertions or deletions shift the reading frame so that a completely different amino acid sequence occurs downstream from the mutation (see Table 15.1). Such a large change in polypeptide structure is likely to inhibit protein function. (Note: The addition or deletion of two, four, or five nucleotides is not considered a point mutation because it involves more than one nucleotide. However, it would be a frameshift mutation if it occurred within the coding sequence of a gene.) Addition and Deletion Mutations Page 313 Mutations and Natural Selection As discussed in Chapter 23, natural selection is the process by which certain traits may become more common or less common in future generations. Some mutations are neutral and are not acted upon by natural selection, because they do not affect an organism's reproductive success. In contrast, other mutations may alter protein function in a way that affects the ability of an organism to survive and to reproduce. These may be beneficial mutations that are favored by natural selection or detrimental (deleterious) mutations that tend to be eliminated by natural about:srcdoc Page 8 of 17 15.1 Consequences of Mutations 12/11/24, 4:53 PM selection. Slipped-strand Mispairing Except for silent mutations, new mutations are more likely to produce polypeptides that have reduced rather than enhanced function. However, mutations can occasionally produce a polypeptide that has an enhanced function. Such beneficial mutations may increase in frequency in a population over the course of many generations due to natural selection. Mutations in the Coding Sequence of a Gene Are Known to Cause Many Human Diseases A key reason why biologists are interested in mutations is because they are known to cause diseases. Thousands of genetic diseases are known to afflict people. Many such genetic disorders are the result of a mutation in one gene. As discussed shortly, some mutations may occur in the germ line that gives rise to sperm or egg cells. If so, these mutations can be passed from parent to offspring. Table 15.2 describes some examples of mutations that affect the coding sequence of single genes and cause disease symptoms. These mutations can be passed from parent to offspring. In addition, as discussed in Section 15.4, mutations may occur in the cells of a person's body, such as lung cells, and cause cancer. These types of mutations are not passed from parent to offspring. about:srcdoc Page 9 of 17 15.1 Consequences of Mutations 12/11/24, 4:53 PM Examples of Inherited Human Diseases That Carry a Mutation in the Coding Table 15.2 Sequence of a Single Gene Type(s) of Disease Gene mutation Symptoms Cystic CFTR Deletion of codon Water imbalance in tissues of the pancreas, intestine, sweat fibrosis 508 glands, and lungs due to impaired ion transport; leads to lung damage Sickle HBB Missense: codon 6 Anemia, blockages in blood circulation cell changed from Glu disease to Val Marfan FBN1 Frameshift, Tall and thin individuals with abnormalities in the skeletal, syndrome nonsense, and ocular, and cardiovascular systems due to a weakening in the deletions elasticity of certain body tissues Progeria LMNA Missense: codon Symptoms that resemble early aging 527 changed from Arg to Leu Tay- HexA Frameshift, Progressive neurodegeneration Sachs nonsense, and disease deletions Let's consider how a mutation can cause disease symptoms at the molecular and cellular level. A striking example occurs in the inherited human disease known as sickle cell disease. This disease involves a missense mutation in the HBB gene, which encodes β-globin—one of the polypeptide subunits that make up hemoglobin, the oxygen-carrying protein in red blood cells. In the most common form of this disease, a missense mutation alters the polypeptide sequence such that the sixth amino acid is changed from a glutamic acid to a valine ( Figure 15.1). Because glutamic acid is hydrophilic but valine is hydrophobic, this single amino acid substitution alters the structure and function of the hemoglobin protein. The mutant hemoglobin subunits tend to stick to one another when the oxygen concentration is low. The aggregated proteins form fiber-like structures within red blood cells, which causes the about:srcdoc Page 10 of 17 15.1 Consequences of Mutations 12/11/24, 4:53 PM cells to lose their normal disc-shaped morphology and become sickle-shaped. It is amazing that a single amino acid substitution could have such a profound effect on the structure of cells. Figure 15.1 A missense mutation that causes red blood cells to sickle in sickle cell disease. Scanning electron micrographs of (a) a normal red blood cell and (b) a sickled red blood cell. As shown above the micrographs, a missense mutation in the β-globin gene (which codes for a subunit of hemoglobin) changes the sixth amino acid in the β-globin polypeptide from glutamic acid (Glu) to valine (Val). (c) This micrograph shows how this alteration to the structure of β-globin causes the formation of abnormal fiber-like structures. In normal red blood cells, hemoglobin proteins do not aggregate together to form fibers. a: Mary Martin/Science Source; b: Science Source; c: Courtesy of Thomas Wellems and Robert Josephs. Electron Microscopy and Image Processing Laboratory, University of Chicago Concept Concept Check: Check: Based on the fiber-like structures seen in part (c) of this figure, what aspect of hemoglobin structure does a glutamic acid at the sixth position in normal β-globin prevent? Speculate as to how the charge of this amino acid may play a role. Answer about:srcdoc Page 11 of 17 15.1 Consequences of Mutations 12/11/24, 4:53 PM Gene Mutations That Occur Outside of Coding Sequences Can Influence Gene Expression Thus far, we have focused our attention on mutations in the coding regions of protein-coding genes. In Chapters 12 and 14, we explored the role of DNA sequences in gene expression. A mutation can occur within a noncoding DNA sequence and affect gene expression ( Table 15.3). For example, a mutation may alter the sequence within the promoter of a gene, thereby affecting the rate of transcription. A mutation that improves the ability of RNA polymerase to bind to the promoter may enhance transcription, whereas other mutations may inhibit transcription. Page 314 about:srcdoc Page 12 of 17 15.1 Consequences of Mutations 12/11/24, 4:53 PM Table 15.3 Effects of Mutations Outside of the Coding Sequence of a Gene Sequence Effect of mutation Promoter May increase or decrease the rate of transcription Transcriptional regulatory May alter the regulation of transcription element/operator site Splice sites May alter the ability of pre-mRNA to be properly spliced Translational regulatory element May alter the ability of mRNA to be translationally regulated Intergenic region Not as likely to have an effect on gene expression Mutations in regulatory elements or operator sites can alter the regulation of gene transcription. For example, in Chapter 14, we considered the roles of regulatory elements such as the lac operator site in E. coli, which is recognized by the lac repressor protein (refer back to Figure 14.7). Mutations in the lac operator site can disrupt the proper regulation of the lac operon. An operator mutation may change the DNA sequence so the lac repressor protein does not bind to it. This mutation would cause the operon to be constitutively expressed. about:srcdoc Page 13 of 17 15.1 Consequences of Mutations 12/11/24, 4:53 PM Mutations Can Occur in Germ-Line or Somatic Cells Let’s now consider how the timing of a mutation may have important consequences for its potential effects. Multicellular organisms typically begin their lives as a single fertilized egg cell that divides many times to produce all the cells of an adult organism. A mutation can occur in any cell of the body, either very early in life, such as in a gamete (egg or sperm) or a fertilized egg, or later in life, such as in the embryonic or adult stage. The number and location of cells with a mutation are critical both to the severity of the genetic effect and to the ability of the mutation to be passed on to offspring. Germ-Line Mutations The term germ line refers to cells that give rise to gametes, such as egg and sperm cells. A germ- line mutation can occur directly in an egg or sperm cell, or it can occur in a precursor cell that produces the gamete. If a mutant human gamete participates in fertilization, all the cells of the resulting offspring will contain the mutation, as indicated by the red color in Figure 15.2a. Likewise, when such an individual produces gametes, the mutation may be transmitted to future generations of offspring. Because humans carry two copies of most genes, a new mutation in a single gene has a 50% chance of being transmitted from parent to offspring. about:srcdoc Page 14 of 17 15.1 Consequences of Mutations 12/11/24, 4:53 PM Figure 15.2 The effects of germ-line versus somatic cell mutations. The red color indicates which cells carry the mutation. (a) In this example, a mutation occurs in a gamete. This germ-line mutation will be passed to every cell of the body. Because humans have two copies of most genes, a germ-line mutation in one of those two copies is transmitted to only half of the gametes. (b) Somatic mutations affect a limited area of the body and are not transmitted to offspring. about:srcdoc Page 15 of 17 15.1 Consequences of Mutations 12/11/24, 4:53 PM Core Concept: Information As a multicellular organism grows and develops, a germ-line mutation is transmitted to all cells of the body, whereas a somatic mutation is found only in a particular region. Click the arrowhead to expand. Additional eBook Question Somatic Mutations The somatic cells constitute all cells of the body except for the germ line. Examples include skin cells and muscle cells. Mutations can also occur within somatic cells at early or late stages of development. What are the consequences of a mutation that happens during the embryonic stage? As shown in Figure 15.2b, a mutation occurred within a single embryonic cell. This single somatic cell was the precursor for many cells of the adult. Therefore, in the adult, a patch of tissue contains cells that carry the mutation. The size of any patch depends on the timing of a new mutation. In general, the earlier a mutation occurs during development, the larger the patch. An individual with somatic regions that are genetically different from each other is called about:srcdoc Page 16 of 17 15.1 Consequences of Mutations 12/11/24, 4:53 PM a mosaic. Figure 15.3 illustrates a child who had a somatic mutation during an early stage of development. In this case, the child has a streak of white hair while the rest of his hair is black. Presumably, a single mutation happened in an embryonic cell that ultimately gave rise to the patch that produced the white hair. Figure 15.3 Example of a somatic mutation. This child has a streak of white hair. This is due to a somatic mutation in a single cell during embryonic development. This cell continued to divide to produce a streak of white hair. Otero/GTphoto Concept Concept Check: Check: Can this child with a streak of white hair transmit this trait to any future offspring? Answer Although a change in hair color is not a harmful consequence, mutations during early stages of life can be quite harmful, especially if they disrupt essential developmental processes. Even though it is sensible to avoid environmental agents that cause mutations at any stage of life, the possibility of somatic mutations is a compelling reason to avoid such agents during the early stages of life such as embryonic and fetal development, infancy, and early childhood. about:srcdoc Page 17 of 17 15.2 Causes of Mutations 12/11/24, 4:54 PM Page 315 Core Skill: Process of Science Feature Investigation | The Lederbergs Used Replica Plating to Show That Mutations Are Random Events Prior to understanding the causes of mutations at the molecular level, scientists considered the following question: Are mutations that affect the traits of an individual caused by pre-existing circumstances, or are they random events that may happen in any gene of any individual? In the 19th century, French naturalist Jean-Baptiste Lamarck proposed that physiological events (such as use or disuse) determine whether traits are passed to offspring. For example, his hypothesis suggested that an individual who practiced and became adept at a physical activity, such as the long jump, would pass that quality on to his or her offspring. Alternatively, geneticists in the early 20th century suggested that genetic variation occurs as a matter of chance. about:srcdoc Page 1 of 19 15.2 Causes of Mutations 12/11/24, 4:54 PM According to this view, those individuals whose genes happen to contain beneficial mutations are more likely to survive and pass those genes to their offspring. Page 316 These opposing views were tested in bacterial studies in the 1940s and 1950s. One such study, by American microbiologists Joshua and Esther Lederberg, focused on the occurrence of mutations in bacteria ( Figure 15.4). First, the Lederbergs placed a few dozen E. coli bacteria onto growth media and incubated them overnight. Following this growth period, each bacterial cell had divided many times to form a visible bacterial colony composed of millions of cells (see step 2). This is called the master plate. (Note: The X on the side of the petri plate marks the orientation of the plate; this orientation is needed to compare the results in subsequent steps.) In step 2, the Lederberg's used a technique known as replica plating, in which a sterile piece of velvet cloth was lightly touched to the master plate to pick up bacterial cells from each colony on the master plate. They then transferred this replica to two secondary plates containing an agent that selected for the growth of bacterial cells with a particular mutation. about:srcdoc Page 2 of 19 15.2 Causes of Mutations 12/11/24, 4:54 PM 15.2 Causes of Mutations Learning Outcomes: 1. CoreSKILL » Analyze the replica plating experiments of the Lederbergs. 2. Describe the difference between spontaneous and induced mutations. 3. Define mutagen, and distinguish between chemical and physical mutagens. 4. CoreSKILL » Analyze the results of an Ames test for determining if a substance is a mutagen. As we have seen, mutations affect the expression of genes in a variety of ways, and their timing can have important consequences. Because mutations can have dramatic effects on individuals’ traits, a great deal of research has focused on their underlying causes. We begin this section with an experiment showing that mutations are random events. We will then explore how mutations can be either spontaneous (caused by mistakes in about:srcdoc Page 3 of 19 15.2 Causes of Mutations 12/11/24, 4:54 PM natural biological processes) or induced (caused by environmental agents). Finally, we will examine a testing method used to determine if a substance causes mutations. HYPOTHESIS Mutations are random events. KEY MATERIALS E. coli cells, T1 phage. Experimental level Conceptual level 1. Place individual bacterial cells onto growth media. 2. Incubate overnight to allow the formation of about:srcdoc Page 4 of 19 15.2 Causes of Mutations 12/11/24, 4:54 PM bacterial colonies. This is called the master plate. 3. Press a velvet cloth (wrapped over a cylinder) onto the master plate, and then lift gently to obtain a replica of each bacterial colony. Press the replica onto 2 secondary plates that contain T1 bacteriophage. Incubate overnight to allow bacterial growth. 4. THE DATA 5. CONCLUSION Mutations are random events. In this case, the mutations occurred on the master plate prior to exposure to T1 bacteriophage. 6. SOURCE Lederberg, J., and Lederberg, E. M. 1952. Replica Plating and Indirect Selection of Bacterial Mutants. Journal of Bacteriology 63: 399–406. Figure 15.4 The experiment performed by the Lederbergs showing that mutations are random events. about:srcdoc Page 5 of 19 15.2 Causes of Mutations 12/11/24, 4:54 PM In the example shown in Figure 15.4, the secondary plates contained T1 bacteriophages, which are viruses that infect bacteria and cause them to lyse. On these plates, the only cells that could grow were the rare ones that had acquired a mutation, termed tonr, that conferred resistance to T1. All other cells were lysed by the proliferation of bacteriophages in the bacteria. Therefore, only a few colonies were observed on the secondary plates. Strikingly, these colonies occupied the same locations on each plate. Page 317 How did the Lederbergs interpret these results? The data indicated that the tonr mutations occurred randomly while the bacterial cells were forming colonies on the nonselective master plate. The presence of T1 bacteriophages in the secondary plates did not cause the mutations to develop. Rather, the T1 bacteriophages simply selected for the growth of tonr mutants that were already in the population. These results supported the idea that mutations are random events. Experimental Questions about:srcdoc Page 6 of 19 15.2 Causes of Mutations 12/11/24, 4:54 PM Experimental Questions 1. Explain the opposing views of mutation prior to the Lederbergs’ study. Answer 2. CoreSKILL » What hypothesis was being tested by the Lederbergs? Answer 3. CoreSKILL » How did the results of the Lederbergs support or falsify the hypothesis? Answer Mutations May Be Spontaneous or Induced Biologists categorize the causes of mutation as spontaneous or induced ( Table 15.4). Spontaneous mutations result from abnormalities in biological processes. Spontaneous mutations reflect the observation that biology isn’t perfect. Enzymes, for example, can occasionally function in an abnormal way. In Chapter 11, we learned that DNA polymerase can make mistakes during DNA replication by putting the wrong base in a newly synthesized daughter strand. Though such errors are rare, due to the proofreading function of DNA polymerase, they do occur. In addition, normal metabolic processes within the cell may produce toxic chemicals such as free radicals that can react directly about:srcdoc Page 7 of 19 15.2 Causes of Mutations 12/11/24, 4:54 PM with the DNA and alter its structure. Finally, the structures of nucleotides are not absolutely stable. On occasion, the structure of a base may spontaneously change, and such a change may cause a mutation if it occurs immediately prior to DNA replication. about:srcdoc Page 8 of 19 15.2 Causes of Mutations 12/11/24, 4:54 PM Table 15.4 Some Common Causes of Gene Mutations Common cause of mutations Description Spontaneous Spontaneous Errors in DNA A mistake by DNA polymerase may cause a point mutation. replication Toxic The products of normal metabolic processes may be reactive metabolic chemicals such as free radicals that can alter the structure of products DNA. Changes in On rare occasions, the linkage between a purine and nucleotide deoxyribose can spontaneously break. Changes in base structure structure (isomerization) may cause mispairing during DNA replication. Transposons As discussed in Chapter 21, transposons are small segments of DNA that can insert at various sites in the genome. If they insert into a gene, they may inactivate the gene. Induced Induced Chemical Chemical substances, such as benzo[a]pyrene, a chemical found agents in cigarette smoke, may cause changes in the structure of DNA. Physical Physical agents such as UV (ultraviolet) light and X-rays can agents damage DNA. The rates of spontaneous mutations vary from species to species and from gene to gene. Larger genes are usually more likely to incur a mutation than are smaller ones. A common rate of spontaneous mutation among various species is approximately 1 mutation for every 1 million genes per cell division, which equals 1 in 106, or simply 10– 6. This is the expected rate of spontaneous mutation, which creates the variation that is the raw material of evolution. Induced mutations are caused by environmental agents that enter the cell and alter the about:srcdoc Page 9 of 19 15.2 Causes of Mutations 12/11/24, 4:54 PM structure of DNA. They cause the mutation rate to be higher than the spontaneous mutation rate. Agents that cause a mutation are called mutagens. Mutagenic agents can be categorized as chemical or physical mutagens ( Table 15.5). We will consider their effects next. about:srcdoc Page 10 of 19 15.2 Causes of Mutations 12/11/24, 4:54 PM Table 15.5 Examples of Mutagens Mutagen Effect(s) on DNA structure Chemical Chemical Nitrous acid Deaminates bases 5-Bromouracil Acts as a base analogue 2-Aminopurine Acts as a base analogue Nitrogen mustard Alkylates bases Ethyl Alkylates bases methanesulfonate (EMS) Benzo[a]pyrene Inserts between bases in the DNA double helix and causes additions or deletions Physical Physical X-rays Cause base deletions, single nicks in DNA strands, crosslinking, and chromosomal breaks UV light Promotes pyrimidine dimer formation, which involves covalent bonds between adjacent pyrimidines (usually T to T, but sometimes C to C) about:srcdoc Page 11 of 19 15.2 Causes of Mutations 12/11/24, 4:54 PM Mutagens Alter DNA Structure in Different Ways Researchers have discovered that an enormous array of agents act as mutagens. We often hear in the news media that we should avoid these agents in our foods and living environments. We even use products such as sunscreens that help us avoid the mutagenic effects of ultraviolet (UV) light from the Sun. The public is often concerned about mutagens for two important reasons. First, mutagenic agents are usually involved in the development of human cancers. Second, because new mutations may be deleterious, people want to avoid mutagens to prevent mutations that may have harmful effects in their future offspring. Chemical Mutagens How do mutagens affect DNA structure? Some chemical mutagens act by covalently modifying the structure of nucleotides. For example, nitrous acid (HNO2) deaminates bases by replacing amino groups with keto groups (replacing —NH2 with ═O). This can change cytosine to uracil. When this altered DNA replicates, the modified base does not pair with the appropriate base in the newly made strand. In this case, uracil pairs with about:srcdoc Page 12 of 19 15.2 Causes of Mutations 12/11/24, 4:54 PM adenine ( Figure 15.5). Nitrous H NH2 / H oxide H O H—N N H (HNO2) H. N H NH--N N N N -N H Sugar Sugar Sugar Cytosine Uracil Adenine (pairswithguanine) (pairswithadenine) Figure 15.5 Deamination and mispairing of modified bases by a chemical mutagen. Nitrous acid changes cytosine to uracil by replacing NH2 with an oxygen. During DNA replication, uracil pairs with adenine, thereby creating a mutation in the newly replicated strand. Page 318 Similarly, 5-bromouracil and 2-aminopurine, which are called base analogues, have structures that are similar to particular bases in DNA and can substitute for them. When incorporated into DNA, they also cause errors in DNA replication. Other chemical mutagens disrupt the appropriate pairing between nucleotides by alkylating bases within the DNA. During alkylation, methyl or ethyl groups are covalently attached to the bases. Examples of alkylating agents include nitrogen mustards (used as a chemical weapon during World War I) and ethyl methanesulfonate (EMS), which is used as a mutagen in laboratory experiments. Some chemical mutagens exert their effects by interfering with DNA replication. For example, benzo[a]pyrene, which is found in automobile exhaust, cigarette smoke, and charbroiled food, is metabolized to a compound (benzopyrene diol epoxide) that inserts between the bases of the double helix, thereby distorting the helical structure. When DNA containing such a mutagen is replicated, single-nucleotide additions and deletions may be incorporated into the newly made strands. about:srcdoc Page 13 of 19 15.2 Causes of Mutations 12/11/24, 4:54 PM Physical Mutagens DNA molecules are also sensitive to physical agents such as radiation. In particular, radiation of short wavelength and high energy, known as ionizing radiation, is known to alter DNA structure. Ionizing radiation includes X-rays and gamma rays. This type of radiation can penetrate deeply into biological materials, where it creates free radicals. These molecules can alter the structure of DNA in a variety of ways. Exposure to high doses of ionizing radiation can cause base deletions, breaks in one DNA strand, or even a break in both DNA strands. Nonionizing radiation, such as UV light, contains less energy, and so it penetrates only the surface of biological materials, such as the skin. Nevertheless, UV light is known to cause mutations. For example, UV light can cause the formation of a thymine dimer, which is a site where two adjacent thymine bases become covalently crosslinked to each other ( Figure 15.6). Figure 15.6 Formation and structure of a thymine dimer. Concept Concept Check: Check: Why is a thymine dimer harmful? Answer about:srcdoc Page 14 of 19 15.2 Causes of Mutations 12/11/24, 4:54 PM Thymine dimers are typically repaired before or during DNA replication. However, if such repair fails to occur, a thymine dimer may cause a mutation when that DNA strand is replicated. When DNA polymerase attempts to replicate over a thymine dimer, proper base pairing does not occur between the template strand and the incoming nucleotides. This mispairing can cause gaps in the newly made strand or the incorporation of incorrect bases. Plants, in particular, must have effective ways to prevent UV damage because they are exposed to sunlight throughout the day. Testing Methods Determine If an Agent Is a Mutagen Because mutagens are harmful, researchers have developed testing methods to evaluate the ability of a substance to cause mutation. One commonly used test is the Ames test, which was developed by American biochemist Bruce Ames in the 1970s. This test uses a strain of a bacterium, Salmonella typhimurium, that cannot synthesize the amino acid histidine. This strain contains a point mutation within a gene that encodes an enzyme required for histidine biosynthesis. The mutation renders the enzyme inactive. The bacteria cannot grow unless histidine has been added to the growth medium. However, a about:srcdoc Page 15 of 19 15.2 Causes of Mutations 12/11/24, 4:54 PM second mutation may correct the first mutation, thereby restoring the ability to synthesize histidine. The Ames test monitors the rate at which this second mutation occurs and thereby indicates whether an agent increases the mutation rate above the spontaneous rate. Figure 15.7 outlines the steps in the Ames test. The suspected mutagen is mixed with a rat liver extract and the strain of S. typhimurium that cannot synthesize histidine. Because some potential mutagens may require activation by cellular enzymes, the rat liver extract provides a mixture of enzymes that may cause such activation. This step improves the ability to identify agents that cause mutations in mammals. As a control, bacteria that have not been exposed to the mutagen are also tested. Figure 15.7 The Ames test for mutagenicity. In this example, 2 million bacterial cells were placed on plates lacking histidine. Two colonies arose from the control sample, whereas 44 arose from the sample exposed to a suspected mutagen. Note: In step 1, the cells are incubated in a medium in which they do not divide. Therefore, each mutation that occurs during step 1 is an independent mutation that can grow when placed on the growth medium shown in step 2. about:srcdoc Page 16 of 19 15.2 Causes of Mutations 12/11/24, 4:54 PM Core Skill: Science and Society Biologists have developed many methods, including the Ames test, for determining if a substance is a mutagen. The results of these tests have prevented the use of many different chemicals in the production of food and also resulted in warning labels on products such as cigarettes. After an incubation period in which mutations may occur, a large number of bacteria are plated on a growth medium that does not contain histidine. The S. typhimurium strain is not expected to grow on these plates. However, if a mutation has occurred that allows a cell to synthesize histidine, the bacterium harboring this second mutation will proliferate during an overnight incubation period to form a visible bacterial colony. Page 319 To estimate the mutation rate, the colonies that grow in the absence of histidine are counted and compared with the total number of bacterial cells that were originally placed on the plate for both the suspected-mutagen sample and the control. The control condition is a measure of the spontaneous mutation rate, whereas the other sample measures the rate of mutation in the presence of the suspected mutagen. As an example, let’s suppose that 2 million bacteria were plated from both the control and the suspected-mutagen tubes. In the control experiment, 2 bacterial colonies were observed. The spontaneous mutation rate is calculated by dividing 2 (the number of mutants) by 2 million (the number of original cells). This equals 1 in 1 million, or 1 × 10–6. By comparison, 44 colonies arose from the suspected-mutagen sample (see Figure 15.7). In this case, the mutation rate is 44 divided by 2 million, which equals 2.2 × 10–5. The mutation rate in the presence of the mutagen is over 20 times higher than the spontaneous mutation rate. How do we judge if an agent is a mutagen? Researchers compare the mutation rate in the presence and absence of the suspected mutagen. The experimental procedure shown in Figure 15.7 is conducted several times. If statistical analysis reveals that the mutation rate in the suspected-mutagen sample is significantly higher than in the control sample, the researchers may tentatively conclude that the agent is a mutagen. Interestingly, many about:srcdoc Page 17 of 19 15.2 Causes of Mutations 12/11/24, 4:54 PM studies have used the Ames test to compare the urine from cigarette smokers with that from nonsmokers. This research has shown that urine from smokers contains much higher levels of mutagens. THE QUESTION Let’s suppose a researcher studied the effects of a suspected mutagen, mutagen X, using the protocol described in Figure Figure 15.7 15.7. The following data were obtained after placing 2 million cells on each plate: Number of colonies Control (no With Trial mutagen) mutagen X 1 3 62 2 2 77 3 5 46 4 2 55 Calculate the average mutation rate in the presence and absence of mutagen X. Conduct a t-test to determine if suspected mutagen X is significantly about:srcdoc Page 18 of 19 15.2 Causes of Mutations 12/11/24, 4:54 PM affecting the mutation rate. T OPIC What What topic topic in in biology biology does does this this question question address? address? The topic is identifying a mutagen. More specifically, the question is about analyzing results from the Ames test. I NFORMATION What What information information dodo you you know know based based on on the the question question and and your your understanding understanding of of the the topic? topic? In the question, you are given data regarding the outcome of four trials using the Ames test. From your understanding of the topic, you may remember that a higher number of colonies on the experimental plate may indicate that a substance is a mutagen. P ROBLEM-SOLVING S TRATEGIES Make Make aa calculation. calculation. Use Use statistics. statistics. To begin to solve this problem, you first need to calculate the average mutation rates. To do this, take the average of the four trials and then divide the average number of mutant colonies by the total number of cells applied to each plate (in this case, 2 million). You also need to conduct a t-test to determine if the control and experimental data are significantly different. A description of a t-test can be found in various statistics textbooks. ANSWER In the control trials, the average mutation rate is 1.5 in 1 million, or 1.5 × 10−6. In the presence of the suspected mutagen, the average rate is 30 in 1 million, or 30 × 10−6. From a t-test of these data, P < 0.01, so you can reject the null hypothesis that the control and experimental data are not different from each other. Therefore, you can accept the hypothesis that the suspected mutagen is causing a higher mutation rate. (Note: This hypothesis is not proven; you are simply able to accept it based on this statistical outcome.) about:srcdoc Page 19 of 19 15.3 DNA Repair 12/11/24, 4:55 PM Page 320 15.3 DNA Repair about:srcdoc Page 1 of 9 15.3 DNA Repair 12/11/24, 4:55 PM Learning Outcomes: 1. List the general features of DNA repair systems. 2. Describe the steps of nucleotide excision repair. 3. Explain the connection between a defect in DNA repair and the inherited human disease xeroderma pigmentosum. In the previous sections, we considered the consequences and causes of mutations. As we have seen, mutations are random events that often have negative consequences. To minimize mutations, all living organisms have the ability to repair changes that occur in the structure of DNA. For example, in Chapter 11, we considered how DNA polymerase has a proofreading function that helps to prevent mutations from arising during DNA replication. In this section, we will examine DNA repair systems that can detect abnormalities in DNA structure and repair them. The importance of these systems becomes evident when they are missing. For example, as discussed at the beginning of this chapter, persons with xeroderma pigmentosum are highly susceptible to the harmful effects of sunlight because they are missing about:srcdoc Page 2 of 9 15.3 DNA Repair 12/11/24, 4:55 PM a single DNA repair system. Direct Repair How do organisms minimize the occurrence of mutations? Cells contain several DNA repair systems that can fix different types of DNA alterations ( Table 15.6). Each repair system is composed of one or more proteins that play specific roles in the repair mechanism. DNA repair requires two coordinated events. In the first step, one or more proteins in the repair system detect an irregularity in DNA structure. In the second step, the abnormality is repaired. about:srcdoc Page 3 of 9 15.3 DNA Repair 12/11/24, 4:55 PM Table 15.6 Common Types of DNA Repair Systems* System Description Direct repair A repair enzyme recognizes an incorrect structure in the DNA and directly restores the correct structure. Base excision An abnormal base or nucleotide is recognized, and a portion of the and nucleotide strand containing the abnormality is removed. The complementary excision repair DNA strand is then used as a template to synthesize a normal DNA strand. Methyl-directed Similar to excision repair except that the DNA defect is a base-pair mismatch mismatch in the DNA, not an abnormal nucleotide. The mismatch is repair recognized, and a strand of DNA in this region is removed. The complementary strand is used to synthesize a normal strand of DNA. Mismatch Repair In some cases, the change in DNA structure can be directly repaired. For example, DNA may be modified by the attachment of an alkyl group, such as —CH2CH3, to a base. In direct repair, an enzyme removes this alkyl group, thereby restoring the structure of the original base. More commonly, however, the altered DNA is removed, and a new segment of DNA is synthesized. In this section, we will examine nucleotide excision repair as an example of how such systems operate. This system, which is found in all species, is an important mechanism of DNA repair. about:srcdoc Page 4 of 9 15.3 DNA Repair 12/11/24, 4:55 PM Nucleotide Excision Repair Removes Segments of Damaged DNA In nucleotide excision repair (NER), a region encompassing several nucleotides in the damaged strand is removed from the DNA, and the intact undamaged strand is used as a template for the resynthesis of a normal complementary strand. NER can fix many different types of DNA damage, including UV-induced damage, such as thymine dimers, chemically modified bases, and missing bases. The system is found in all species, although its molecular mechanism is best understood in bacteria. Thymine Dimers: Formation and Repair In E. coli, the NER system is composed of four key proteins: UvrA, UvrB, UvrC, and UvrD. They are named Uvr because they are involved in ultraviolet light repair of thymine dimers, although these proteins are also important in repairing chemically damaged DNA. In addition, DNA polymerase and DNA ligase are required to complete the repair process. How does the NER system work? 1. Two UvrA proteins and one UvrB protein form a complex that tracks along the DNA ( Figure 15.8). Damaged DNA will have a distorted double helix, which is sensed by the UvrA-UvrB complex. 2. When the complex identifies a damaged site, the two UvrA proteins are released, and UvrC binds to UvrB at the site. 3. The UvrC protein makes incisions in one DNA strand on both sides of the damaged site. 4. After this incision process, UvrC is released. UvrD binds to UvrB. UvrD then begins to separate the DNA strands, and UvrB is released. The action of UvrD unravels the DNA, which removes a short DNA strand that contains the damaged region. UvrD is released. 5. After the damaged DNA strand is removed, a gap is left in the double helix. DNA polymerase fills in the gap using the undamaged strand as a template. Finally, DNA ligase makes the final covalent connection between the newly made DNA and the original DNA strand. about:srcdoc Page 5 of 9 15.3 DNA Repair 12/11/24, 4:55 PM Page 321 about:srcdoc Page 6 of 9 15.3 DNA Repair 12/11/24, 4:55 PM about:srcdoc Page 7 of 9 15.3 DNA Repair 12/11/24, 4:55 PM Figure 15.8 Nucleotide excision repair in E. coli coli. Concept Check: Which components of the NER system are responsible for removing the damaged DNA? Answer Nucleotide Excision Repair Human Genetic Diseases Occur When a about:srcdoc Page 8 of 9 15.3 DNA Repair 12/11/24, 4:55 PM Human Genetic Diseases Occur When a Component of the NER System Is Missing Thus far, we have considered the NER system in E. coli. In humans, NER systems were discovered by the analysis of genetic diseases that affect DNA repair. These include xeroderma pigmentosum (XP), which was discussed at the beginning of this chapter, and Cockayne syndrome (CS). Photosensitivity is a common characteristic in individuals with these syndromes because of an inability to repair UV-induced lesions. Therefore, people with either of these syndromes must avoid prolonged exposure to sunlight, as do the children at Camp Sundown, described at the beginning of this chapter (see chapter-opening photo). Exposure to sunlight may cause pigmentation changes, precancerous lesions, and a predisposition to developing skin cancer. about:srcdoc Page 9 of 9 15.4 Cancer 12/11/24, 4:57 PM 15.4 Cancer about:srcdoc Page 1 of 29 15.4 Cancer 12/11/24, 4:57 PM Learning Outcomes: 1. Outline the steps in the development of cancer. 2. Describe the general functions of oncogenes. 3. List the four common types of genetic changes that convert proto-oncogenes into oncogenes. 4. Identify the two general functions of the proteins encoded by tumor- suppressor genes. 5. Describe three common ways that tumor-suppressor genes are silenced. Cancer is a disease of multicellular organisms characterized by uncontrolled cell division. Worldwide, cancer is the second leading cause of death in humans, exceeded only by heart disease. In the United States, approximately 1.5 million people are diagnosed with cancer each year; over 0.5 million will die from the disease. Overall, about one in four Americans will die from cancer. about:srcdoc Page 2 of 29 15.4 Cancer 12/11/24, 4:57 PM For about 10% of cancers, a higher predisposition to develop the disease is an inherited trait. Most cancers, though, perhaps 90%, do not involve genetic changes that are passed from parent to offspring. Rather, cancer is usually an acquired condition that typically occurs later in life. At least 80% of all human cancers are related to exposure to carcinogens, agents that increase the likelihood of developing cancer. Most carcinogens, such as UV light and certain chemicals in cigarette smoke, are mutagens that promote genetic changes in somatic cells. These genetic changes can alter gene expression in a way that ultimately affects cell division, leading to cancer. In this section, we will explore such genetic abnormalities. How does cancer occur? In most cases, the development of cancer is a multistep process ( Figure 15.9). Cancers originate from a single cell. This single cell and its lineage of daughter cells undergo a series of mutations and other genetic changes that cause the cells to grow abnormally. At an early stage, the cells form a tumor, which is an abnormal overgrowth of cells. For most types of cancer, growth begins as a precancerous mass, or a benign tumor. Such tumors do not invade adjacent tissues and do not spread throughout the body. This may be followed by additional genetic changes that cause some cells in the tumor to lose their normal growth regulation, and it becomes a malignant tumor. At this stage, the individual has cancer. Page 322 Cancerous tumors invade adjacent healthy tissues, and cancer cells may spread through the bloodstream or surrounding body fluids, a process called metastasis. If left untreated, malignant cells will cause the death of the organism. about:srcdoc Page 3 of 29 15.4 Cancer 12/11/24, 4:57 PM Figure 15.9 Cancer: its typical progression and effects. (a) In a healthy individual, a few mutations convert a normal cell into a tumor cell. This cell divides to produce a benign tumor. Additional mutations and other changes in the tumor cells may occur, leading to a malignant tumor. At a later stage in malignancy, the tumor cells invade surrounding tissues, and some malignant cells may metastasize by traveling through the bloodstream to other parts of the body. (b) On the left in the photo is a human lung that was obtained from a healthy nonsmoker. The lung shown on the right has been ravaged by lung cancer. This lung was taken from a person who was a heavy smoker. b: St. Bartholomew’s Hospital/Science Source Over the past few decades, researchers have identified many genes that promote cancer when they are mutant. By comparing the function of each mutant gene with the corresponding nonmutant gene found in healthy cells, these cancer-promoting genes have been placed into two categories. In some cases, a mutation causes a gene to be overactive—have an abnormally high level of expression. This overactivity contributes to the uncontrolled cell growth that is observed in cancer cells. This type of mutant gene is called an oncogene. Alternatively, when a tumor-suppressor gene is normal (that is, not mutant), it encodes a about:srcdoc Page 4 of 29 15.4 Cancer 12/11/24, 4:57 PM protein that helps to prevent cancer. However, when a mutation eliminates its function, cancer may occur. Thus, the two categories of cancer-causing genes are based on the effects of mutations. Oncogenes are the result of mutations that cause overactivity, whereas cancer-causing mutations in tumor-suppressor genes are due to a loss of activity. In this section, we will begin with a discussion of oncogenes and then consider tumor-suppressor genes. Oncogenes May Result from Mutations That Cause the Overactivity of Proteins Involved with Cell Division Over the past four decades, researchers have identified many oncogenes. A large number of oncogenes encode proteins that function in signal transduction pathways involved in cell growth. Cell division is regulated, in part, by growth factors. A growth factor binds to a receptor, which results in receptor activation ( Figure 15.10). This stimulates an intracellular signal transduction pathway that activates transcription factors. In this way, the transcription of specific genes is activated in response to a growth factor. After they are made, the gene about:srcdoc Page 5 of 29 15.4 Cancer 12/11/24, 4:57 PM products promote cell division. Figure 15.10 General features of a signal transduction pathway involving a growth factor that promotes cell division. A detailed description of this pathway is found in Chapter 9 (look back at Figure 9.10). Concept Concept Check: Check: How does the presence of a growth factor ultimately affect the function of a cell? Answer about:srcdoc Page 6 of 29 15.4 Cancer 12/11/24, 4:57 PM Core Skill: Connections Look back at Figure 9.10. Could drugs that inhibit protein kinases be used to combat cancer? Explain. Answer Click the arrowheads to expand. Figure 9.10 Additional eBook Question Eukaryotic species produce many different growth factors that play a role in cell division. Likewise, cells have several different types of signal transduction pathways, which are composed of proteins that respond to growth factors and promote cell division. Mutations in the genes that encode these signal transduction proteins can change them into oncogenes ( Table 15.7). about:srcdoc Page 7 of 29 15.4 Cancer 12/11/24, 4:57 PM Examples of Genes That Encode Signal Transduction Table 15.7 Proteins and Can Become Oncogenes Gene* Cellular function of encoded protein erbB Growth factor receptor for EGF (epidermal growth factor) ras Intracellular signaling protein raf Intracellular signaling protein src Intracellular signaling protein fos Transcription factor jun Transcription factor How does an oncogene promote cancer? In some cases, an oncogene may keep a signal transduction pathway for cell division in a permanent “on” state. One way oncogenes keep cell division turned on is by producing a functionally overactive protein. Page 323 As a specific example, let’s consider how a mutation alters an intracellular signaling protein called Ras (refer back to Figure 9.10). The Ras protein is a GTPase that hydrolyzes GTP to GDP + Pi ( Figure 15.11). When a signal transduction pathway is activated, the Ras protein releases GDP and binds GTP. When GTP is bound, the activated Ras protein promotes cell division. The Ras protein returns to its inactive state by hydrolyzing its bound GTP, and cell division is inhibited. about:srcdoc Page 8 of 29 15.4 Cancer 12/11/24, 4:57 PM Figure 15.11 The function of Ras, a protein that is part of signal transduction pathways. When GTP is bound, the activated Ras protein promotes cell division. When GTP is hydrolyzed to GDP and Pi, Ras is inactivated, and cell division is no longer activated. Stimulation of Cell Replication Mutations that convert the normal ras gene into an oncogene either decrease the ability of Ras protein to hydrolyze GTP or increase the rate of exchange of bound GDP for GTP. Both of these functional changes result in a greater amount of the active GTP-bound form of the Ras protein. In this way, these mutations keep the signal transduction pathway turned on when it should not be, resulting in uncontrolled cell division. Page 324 about:srcdoc Page 9 of 29 15.4 Cancer 12/11/24, 4:57 PM Mutations in Proto-Oncogenes Convert Them to Oncogenes Thus far, we have examined the functions of proteins that cause cancer when they become overactive, resulting in uncontrolled cell division. Let’s now consider the common types of genetic changes that create such oncogenes. A proto-oncogene is a normal gene that, if mutated, can become an oncogene. An oncogene is a gene that has been altered in a way that causes it to be overexpressed or expressed in the wrong cell type. Several types of genetic changes may convert a proto-oncogene into an oncogene. Figure 15.12 describes four common types: missense mutations, gene amplifications, chromosomal translocations, and retroviral insertions. about:srcdoc Page 10 of 29 15.4 Cancer 12/11/24, 4:57 PM about:srcdoc Page 11 of 29 15.4 Cancer 12/11/24, 4:57 PM Figure 15.12 Common genetic changes that convert proto-oncogenes to oncogenes. Missense Mutation A missense mutation ( Figure 15.12a), which changes a single amino acid in a protein, alters the function of the encoded protein in a way that promotes cancer. This type of mutation is responsible for the conversion of the ras gene into an oncogene. An example is a mutation in the ras gene that changes a specific glycine to a valine in the Ras protein. This mutation decreases the ability of the Ras protein to hydrolyze GTP, which keeps the Ras protein in the GTP-bound form and thereby promotes cell division (see Figure 15.11). Experimentally, chemical mutagens have been shown to cause this missense mutation, thereby leading to cancer. about:srcdoc Page 12 of 29 15.4 Cancer 12/11/24, 4:57 PM Gene Amplification Another genetic event that occurs in some cancer cells is an increase in the number of copies of a proto-oncogene ( Figure 15.12b). An abnormal increase in the number of genes results in too much of the encoded protein. Many human cancers are associated with the amplification of particular proto-oncogenes. In 1982, American molecular biologist Mark Groudine discovered that the myc gene, which encodes a transcription factor, is amplified in a human leukemia. Chromosomal Translocation A third type of genetic alteration that can lead to cancer is a chromosomal translocation ( Figure 15.12c). This occurs when one segment of a chromosome becomes attached to a different chromosome. In 1960, American pathologist Peter Nowell discovered that a form of leukemia—a type of cancer involving white blood cells—called chronic myelogenous leukemia (CML) is correlated with the presence of a shortened version of a human chromosome. This shortened chromosome is the result of a chromosome translocation in which two different chromosomes, chromosomes 9 and 22, exchange pieces. This translocation activates a proto-oncogene, abl, in an unusual way ( Figure 15.13). In about:srcdoc Page 13 of 29 15.4 Cancer 12/11/24, 4:57 PM healthy individuals, the bcr gene and the abl gene are located on different chromosomes. In CML, these chromosomes break and rejoin such that the promoter and the first part of bcr fuse with part of abl. This fused gene acts as an oncogene and encodes a fusion protein whose functional overactivity leads to leukemia. Figure 15.13 The formation of a fused gene found in people with certain forms of leukemia. The fusion of the bcr and abl genes creates a fused gene that encodes a fusion protein, leading to leukemia. The blue regions are the promoters for the bcr and abl genes. Concept Concept Check: Check: The bcr gene is normally expressed in white blood cells. Explain how this observation is related to the type of cancer caused by the translocation between chromosomes 9 and 22. Answer about:srcdoc Page 14 of 29 15.4 Cancer 12/11/24, 4:57 PM Retroviral Insertion Certain types of viruses convert proto-oncogenes into oncogenes during the viral replication cycle (see Figure 15.12d). Retroviruses insert their DNA into the chromosomal DNA of the host cell. The viral genome contains promoter and regulatory elements that cause a high level of expression of viral genes. On occasion, the viral DNA may insert into a host chromosome in such a way that a viral promoter and regulatory elements are next to a proto-oncogene. This may result in the overexpression of the proto-oncogene, thereby promoting cancer. This is one way for a virus to cause cancer. Alternatively, a virus may cause cancer because it carries an oncogene in its viral genome. This phenomenon is described next. Page 325 Some Types of Cancer Are Caused by Viruses The majority of cancers are caused by mutagens or other changes that alter the structure and expression of genes that are found in somatic cells. A few viruses, however, are known to cause cancer in plants and animals, including humans ( Table 15.8). about:srcdoc Page 15 of 29 15.4 Cancer 12/11/24, 4:57 PM Table 15.8 Examples of Viruses That Cause Cancer Virus Description Rous sarcoma virus Causes sarcomas in chickens Simian sarcoma virus Causes sarcomas in monkeys Abelson leukemia virus Causes leukemia in mice Hardy-Zuckerman 4 feline Causes sarcomas in cats sarcoma virus Hepatitis B Causes liver cancer in several species, including humans In 1911, the first cancer-causing virus to be discovered was isolated from chicken sarcomas by American pathologist Peyton Rous. A sarcoma is a tumor of connective tissue such as bone or cartilage. The virus was named the Rous sarcoma virus (RSV). In the 1970s, research involving RSV led to the identification of a viral gene that acts as an oncogene. Researchers investigated RSV by using it to infect chicken cells grown in the laboratory. This infection causes the chicken cells to grow like cancer cells, continuously and in an uncontrolled manner. Researchers identified mutant RSV strains that infected and proliferated within chicken cells without transforming them into malignant cells. These RSV strains were missing a gene that is found in the form of the virus that does cause cancer. This gene was called the src gene because it causes sarcoma. Cell Proliferation Signaling Pathway Later, other American researchers, biologist Harold Varmus and microbiologist Michael Bishop, in collaboration with molecular biologist Peter Vogt, discovered that normal (nonviral- infected) chicken cells also contain a copy of the src gene in their chromosomes. This gene is a proto-oncogene. When it is incorporated into a viral genome, it is overexpressed because it is transcribed from a very active viral promoter. This overexpression ultimately produces too much of the Src protein in infected cells and promotes uncontrolled cell division. about:srcdoc Page 16 of 29 15.4 Cancer 12/11/24, 4:57 PM Tumor-Suppressor Genes Prevent Mutation or Cell Proliferation Thus far, we have examined one category of genes that promote cancer, namely oncogenes. We now turn our attention to the second category, those called tumor-suppressor genes. The functioning of a normal (nonmutant) tumor-suppressor gene prevents cancerous growth. The proteins encoded by tumor-suppressor genes usually have one of two functions: maintenance of genome integrity or negative regulation of cell division ( Table 15.9). about:srcdoc Page 17 of 29 15.4 Cancer 12/11/24, 4:57 PM Table 15.9 Functions of Selected Tumor-Suppressor Genes Gene Function Maintenance Maintenance of of genome genome integrity integrity p53 p53 is a transcription factor that acts as a sensor of DNA damage. It can promote DNA repair, prevent the advancement through the cell cycle, and promote apoptosis. BRCA-1 BRCA-1 and BRCA-2 proteins are both involved BRCA-2 in the cellular defense against DNA damage. They play a role in sensing DNA damage and facilitate DNA repair. These genes are mutant in persons with certain inherited forms of breast cancer. XPD This represents one of several different genes whose products function in DNA repair. These genes are defective in patients with xeroderma pigmentosum. Negative Negative regulation regulation of of cell cell division division Rb The Rb protein is a negative regulator that represses the transcription of genes required for DNA replication and cell division. NF1 The NF1 protein stimulates Ras to hydrolyze its GTP to GDP. Loss of NF1 function causes the Ras protein to be overactive, which promotes cell division. p16 The p16 protein is a negative regulator of cyclin- dependent kinases (cdks). Page 326 about:srcdoc Page 18 of 29 15.4 Cancer 12/11/24, 4:57 PM Maintenance of Genome Integrity Some tumor-suppressor genes encode proteins that maintain the integrity of the genome by monitoring and/or repairing genome alterations. The proteins encoded by these genes are vital for the prevention of abnormalities such as gene mutations, DNA breaks, and improperly segregated chromosomes. Therefore, when these proteins are functioning properly, they minimize the chance that a cancer-causing mutation will occur. In some cases, the proteins encoded by tumor-suppressor genes prevent a cell from advancing through the cell cycle if an abnormality is detected. These are termed checkpoint proteins because their role is to check the integrity of the genome and prevent a cell from progressing past a certain point in the cell cycle. Checkpoint proteins are not always required to promote normal, healthy cell division, but they can stop cell division if an abnormality is detected. How do checkpoint proteins stop the cell cycle? One way is by controlling proteins called cyclins and cyclin-dependent kinases (cdks), which are responsible for advancing a cell through the four phases of the cell cycle (see Chapter 16). The formation of activated cyclin/cdk complexes can be stopped by checkpoint proteins. A specific example of a tumor-suppressor gene that encodes a checkpoint protein is p53, discovered in 1979 by American biologist Arnold Levine. Its name refers to the molecular mass of the p53 protein, which is 53 kDa (kilodaltons). About 50% of all human cancers, including malignant tumors of the lung, breast, esophagus, liver, bladder, and brain, as well as leukemias and lymphomas (cancer of the lymphatic system), are associated with mutations in this gene. As shown in Figure 15.14, p53 is a protein that controls the ability of cells to advance from the G1 stage of the cell cycle to the S phase. The expression of the p53 gene is induced when DNA is damaged. The p53 protein functions as a regulatory transcription factor that activates several different genes, leading to the synthesis of proteins that stop the cell cycle and other proteins that repair the DNA. If the DNA is eventually repaired, a cell may later proceed through the cell cycle. about:srcdoc Page 19 of 29 15.4 Cancer 12/11/24, 4:57 PM Figure 15.14 The cell cycle and checkpoints. As discussed in Chapter 16, eukaryotic cells advance through a cell cycle composed of G1, S, G2, and M phases (look ahead to Figure 16.2). The yellow bars indicate common checkpoints where the cell cycle is stopped if genetic abnormalities are detected. The p53 protein stops a cell at the G1 checkpoint if it senses DNA damage. Concept Concept Check: Check: Why is it an advantage for an organism to have checkpoints where the cell cycle can be stopped? Answer Alternatively, if the DNA damage is too severe, the p53 protein will also activate other genes that promote programmed cell death. This process, called apoptosis, involves cell shrinkage and DNA degradation. As described in Chapter 9, enzymes known as caspases are activated during apoptosis (refer back to Figure 9.19). They function as proteases that are sometimes called the “executioners” of the cell. Caspases digest selected cellular proteins such as microfilaments, which are components of the cytoskeleton. The destruction of the microfilaments causes the cell to break into small vesicles that are eventually phagocytized by cells of the immune system. It is beneficial for a multicellular organism to kill an occasional cell with cancer-causing potential. How Tumor Suppressor Genes Block Cell Division about:srcdoc Page 20 of 29 15.4 Cancer 12/11/24, 4:57 PM Negative Regulation of Cell Division A second category of tumor-suppressor genes encodes proteins that are negative regulators or inhibitors of cell division. These proteins must function properly to halt cell division. If their function is lost, cell division is abnormally accelerated. An example of such a tumor-suppressor gene is the Rb gene. It was the first tumor-suppressor gene to be identified in humans, from studies of patients with a disease called retinoblastoma, a cancerous tumor that occurs in the retina of the eye. The Rb protein negatively controls a regulatory transcription factor called E2F that activates genes required for cell cycle progression from G1 to S phase. The binding of the Rb protein to E2F inhibits E2F's activity and thereby prevents cell division ( Figure 15.15). When a normal cell is supposed to divide, cyclins bind to cyclin-dependent kinases (cdks). This binding activates the kinases, which catalyze the transfer of a phosphate to the Rb protein. The phosphorylated form of the Rb protein is released from E2F, thereby allowing E2F to activate genes needed to advance through the cell cycle. about:srcdoc Page 21 of 29 15.4 Cancer 12/11/24, 4:57 PM Figure 15.15 Function of the Rb protein. The Rb protein inhibits the function of E2F, which turns on genes that cause a cell to divide. When cells are supposed to divide, Rb is phosphorylated by a cyclin-dependent kinase, which allows E2F to function. Concept Concept Check: Check: Would cancer occur if both copies of the Rb gene and both copies of the E2F gene were rendered inactive due to mutations? Answer Figure 15.15 illustrates the normal functioning of Rb and E2F proteins. However, when both copies of Rb are defective due to mutations, the E2F protein is always active. This explains why uncontrolled cell division occurs in retinoblastoma. about:srcdoc Page 22 of 29 15.4 Cancer 12/11/24, 4:57 PM Gene Mutations, Chromosome Loss, and Changes in Chromatin Structure Can Inhibit the Expression of Tumor-Suppressor Genes Cancer biologists want to understand how tumor-suppressor genes are inactivated, because this knowledge may ultimately help them to prevent or combat cancer. How are tumor-suppressor genes silenced? The function of tumor-suppressor genes is lost in three common ways. Page 327 Inactivation of Tumor-Suppressor Genes via Mutation First, a mutation can occur within a tumor-suppressor gene to inactivate its function. For example, a mutation could abolish the function of the promoter for a tumor-suppressor gene or introduce an early stop codon into its coding sequence. Either of these would prevent the expression of a functional protein. about:srcdoc Page 23 of 29 15.4 Cancer 12/11/24, 4:57 PM Chromosome Loss Chromosome loss is a second way that the function of a tumor-suppressor gene is lost. Chromosome loss may contribute to the progression of cancer if the missing chromosome carries one or more tumor-suppressor genes. Epigenetic Changes Researchers have discovered a third way that tumor-suppressor genes may be inactivated. Epigenetic changes involve changes in chromatin structure that alter gene expression without altering the base sequence of DNA (see Chapter 18). For example, tumor-suppressor genes found in cancer cells are sometimes abnormally methylated. As discussed in Chapter 14, transcription is inhibited when CpG islands near a promoter are methylated. Such DNA methylation near the promoters of tumor-suppressor genes has been found in many types of tumors, suggesting that this form of gene inactivation plays an important role in the formation and/or progression of malignancy. about:srcdoc Page 24 of 29 15.4 Cancer 12/11/24, 4:57 PM Most Forms of Cancer Are Caused by a Series of Genetic Changes That Progressively Alter the Growth Properties of Cells The discovery of oncogenes and tumor-suppressor genes has allowed researchers to study the progression of certain forms of cancer at the molecular level. In most cases, multiple genetic changes to the same cell lineage, perhaps in the range of 10 or more changes, are needed for cancer to occur. Such changes involve the overexpression of oncogenes and the inactivation of tumor-suppression genes. Many cancers begin with a benign genetic alteration that, over time and with additional genetic changes, leads to malignancy. Furthermore, a malignancy can continue to accumulate genetic changes that make it even more difficult to treat because the cells divide faster or invade surrounding tissues more readily. As an example, let's consider lung cancer, which is diagnosed in approximately 170,000 women and men each year in the United States. More than 1.2 million cases are diagnosed worldwide. Nearly 90% of these cases are caused by tobacco smoking and are thus preventable. Unlike other cancers for which early diagnosis is possible, lung cancer is usually detected only after it has become advanced and is difficult if not impossible to cure. The 5-year survival rate for lung cancer patients is approximately 15%. What is the cellular basis for lung cancer? Most cancers of the lung are carcinomas—cancers of epithelial cells ( Figure 15.16). (Epithelial cells, which form the lining of all internal and external body surfaces, are described in Chapter 10.) The top images in Figure 15.16 show the normal epithelium found in a healthy lung. The rest of the figure shows the progression of a carcinoma that is due to mutations in basal cells, a type of epithelial cell. 1. Cancer occurs due to the accumulation of mutations in a cell lineage, beginning with an initial mutant cell that then divides multiple times to produce a population of many daughter cells (see Figure 15.9). As mutations accumulate in a lineage of basal cells, the number of these cells increases dramatically. This causes a thickening of the epithelium, a condition called hyperplasia. 2. The proliferation of the basal cells causes the loss of the ciliated, columnar epithelial cells about:srcdoc Page 25 of 29 15.4 Cancer 12/11/24, 4:57 PM that normally line the airways and help remove mucus and its trapped particles from the lungs. Page 328 3. As additional mutations accumulate in this cell lineage, the basal cells develop more abnormal morphologies, a condition known as dysplasia. In the early stages of dysplasia, the abnormal basal cells are precancerous. If the source of chronic irritation (usually cigarette smoke) is eliminated, the abnormal cells are likely to disappear. 4. If smoking continues, these abnormal cells may accumulate additional genetic changes and lose the ability to stop dividing. Such cells have become cancerous—the person has basal cell carcinoma. about:srcdoc Page 26 of 29 15.4 Cancer 12/11/24, 4:57 PM Figure 15.16 Progression of changes leading to about:srcdoc Page 27 of 29 15.4 Cancer 12/11/24, 4:57 PM lung cancer. Lung tissue is largely composed of different types of connective tissue and epithelial cells, including columnar and basal cells. A progression of cellular changes in basal cells, caused by the accumulation of mutations and epigenetic changes, leads to basal cell carcinoma, a common type of lung cancer. ©Dr. Oscar Auerbach, reproduced with permission The basement membrane is a sheetlike layer of extracellular matrix that provides a barrier between the lung cells and the bloodstream. If the cancer cells have not yet penetrated the basement membrane, they will not have metastasized, that is, spread into the blood and to other parts of the body. If the entire tumor is removed at this stage, the patient should be cured. The lower images in Figure 15.16 show a tumor that has broken through the basement membrane. The metastasis of these cells to other parts of the body will likely kill the patient, usually within a year of diagnosis. The cellular changes that lead to lung cancer are correlated with genetic changes. These include the occurrence of mutations that create oncogenes and inhibit tumor-suppressor genes. The order of mutations is not absolute. It takes time for multiple changes to accumulate, so cancer is usually a disease of older people. Reducing your exposure to mutagens such as cigarette smoke throughout your lifetime helps minimize the risk of mutations to your genes that could promote cancer. about:srcdoc Core Concept: Evolution Page 28 of 29 15.4 Cancer 12/11/24, 4:57 PM Core Concept: Evolution Mutations in Approximately 300 Human Genes May Promote Cancer Researchers have identified a large number of genes that are mutated in cancer cells. Though not all of these mutant genes have been directly shown to affect the growth rate of cells, such mutations are likely to be found in tumors because they provide some type of growth advantage for the cell population from which the cancer developed. For example, certain mutations may affect the functions of proteins that enable cells to metastasize to neighboring locations. These mutations may not affect growth rate, but they provide a growth advantage in that cancer cells are not limited to growing in a particular location. They can migrate to new locations. How many genes can contribute to cancer when they become mutant? Researchers have estimated that about 300 different genes may play a role in the development of human cancer. With an approximate human genome size of 22,000 protein-coding genes, this observation indicates that over 1% of our genes have the potential to promote cancer if their expression is altered by a mutation or an epigenetic change. about:srcdoc Page 29 of 29