Respiratory Chain & Oxidative Phosphorylation PDF
Document Details
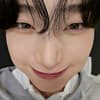
Uploaded by AstoundedGuitar9441
IIUM
Tags
Summary
These notes explain the respiratory chain and oxidative phosphorylation, focusing on how aerobic organisms capture energy from substrates metabolism. The process occurs in mitochondria, where respiration is coupled to oxidative phosphorylation to generate ATP. The notes also provide detail of various components and processes involved, including diagrams and figures.
Full Transcript
Harper’s Illustrated Biochemistry Lippincotts Explain how aerobic organisms capture the available free energy from substrates metabolism. Occur in mitochondria. Respiration is coupled to oxidative phosphorylation ▪ Generate ATP Gib...
Harper’s Illustrated Biochemistry Lippincotts Explain how aerobic organisms capture the available free energy from substrates metabolism. Occur in mitochondria. Respiration is coupled to oxidative phosphorylation ▪ Generate ATP Gibbs free energy Reactions with large G are made possible by coupling endergonic process with exergonic process. absorbs energy from surround release energy Eg: endergonic movement of ions against concentration gradient across membrane. ▪ Coupled with exergonic hydrolysis of ATP Outer membrane – permeable to most metabolites Inner membrane - selectively permeable A matrix within. Inner mitochondrial membrane. Electrons are passed down. Lose much of free energy. Some are captured-stored by ATP production. energy released Energy liberated during the oxidation of carbohydrate, fatty acids, and amino acids ▪ as reducing equivalents (H or electrons). Reducing equivalents, produce most by ▪ β-oxidation ▪ citric acid cycle Mitochondria contain the respiratory chain ▪ collects and transports reducing equivalents ▪ directing them to their final reaction with oxygen to form water ▪ the machinery for trapping the liberated free energy as high-energy phosphate The reduced NADH oxidized by a metalloflavoprotein enzyme—NADH dehydrogenase. Passes reducing equivalents on to Q. Electrons flow from Q through the series of cytochromes to molecular oxygen. The terminal cytochrome aa3 (cytochrome oxidase) ▪ the final combination of reducing equivalents with molecular oxygen ▪ has a very high affinity for oxygen ▪ allowing the respiratory chain to function at maximum rate until the tissue has become depleted of O2. Protein complexes ▪ I, II, III, IV and V. ▪ Carriers are integral protein ▪ Prostetic groups that accept/donate electron. Complex I-IV : contain part of ETC. ▪ Each complex accepts/donates electron to mobile electron carriers. ▪ Coenzyme Q, cytochrome c ▪ Electron eventually combine with oxygen & protons – water Complex V ▪ Spans the inner mitochondria membrane. ▪ Catalyzes ATP – ATP synthase. The iron-sulfur protein (FeS; nonheme iron). Associated with flavoproteins, cytochrome b. Are thought to take part in the oxidoreduction mechanism between flavin and Q ▪ involves only a single e− change ▪ the iron atom undergoing oxidoreduction between Fe2+ and Fe3+. Flow of electrons spontaneous ▪ next carrier has greater affinity for electrons compared to previous. In addition to NADH, FADH2, types of redox centers in the complexes ▪ FMN (flavin mononucleotide) ▪ Fe-S (iron-sulfur clusters) ▪ CoQ (ubiquinone) ▪ Cytochromes NADH dehydrogenase/NADH:ubiquinone oxidoreductase. Embedded in the inner membrane of mitochondria. Has FMN (flavin mononucleotide) molecule ▪ coenzyme structurally related to FAD. ▪ accept the two H (:H-, free H+) ▪ FMNH2 Electron transfers to Fe-S cluster → ubiquinone (CoQ). ▪ form ubiquinol, QH2 (freely diffuses within the membrane). 4 H+ translocated from the mitochondrial matrix to intermembrane space. Mobile carriers. Quinone derivative. The structure very similar to vitamin K & E, plastoquinone (found in chloroplasts). Oxidized-quinone, reduced-quinol, under aerobic or anaerobic conditions, respectively. Collects reducing equivalents from fixed flavoprotein complexes ▪ passes them on to the cytochromes. 2 forms exist ▪ Succinate dehydrogenase (TCA) ▪ Acyl-CoA dehydrogenase (-oxidation). Associated with FAD - flavoprotein. Has Fe-S clusters as prostetic groups. Direct the transfer of electrons from their substrate molecule (succinate, acyl CoA) to CoQ via FADH2. The remaining members of ETC. Contain heme group (a porphyrin ring & iron). Iron: reversibly converted from ferric (Fe3+) to ferrous (Fe2+) form –reversible carrier of electron. Electrons → CoQ → cytochromes bc1 (complex III) → c → a + a3 (complex IV). Cytochrome c ▪ Mobile carrier with the intermembrane space. Cytochrome bc1 complex. Electrons transfer from ubiquinol. Fe-S clusters. Cytochromes ▪ Electron-transfering proteins. ▪ Contains heme prosthetic group. ▪ Accept & donate ONE electron per redox cycle. Electrons transfer translocate more H+ (4). Cytochrome a + a3. Combined - cytochrome c oxidase. 4 electrons from 4 molecules of cytochrome c transferred to O2 - two molecules of water. O2 + 4e- + 4H+ → 2H2O 2 protons are translocated across the membrane. Reduced products must be recycled: ▪ Oxidation of NADH & FADH2 by electron acceptor, O2. ADP + Pi ATP NADH + H+ ½ O2 → NAD+ + H2O ADP + Pi ATP FADH2 + ½ O2 → FAD + H2O Oxidized coenzymes reformed. Energy released is coupled to ATP synthesis. Complex I (NADH dehydrogenase) Complex II (succinate dehydrogenase) Complex III (cytochrome bc1 complex) Complex IV (cytochrome c oxidase) Reactions by dehydrogenase (eg: TCA cycle) Remove two H from substrate. Transferred two electrons & one proton (hydride ions, :H-) to NAD+. Form NADH + H+. NADH carries ▪ :H-, free H+ Pyruvate and α-ketoglutarate dehydrogenase have complex systems involving lipoate and FAD prior to the passage of electrons to NAD. Electron transfers from other dehydrogenases, eg, L(+)-3- hydroxyacyl- CoA dehydrogenase, couple directly with NAD. Preparatory step. Mediated by Substrate Shuttles. NADH cannot penetrate the mitochondrial membrane, but it is produced continuously in the cytosol (glycolysis). Under aerobic conditions - extramitochondrial NADH does not accumulate ▪ presumed to be oxidized by the respiratory chain. The transfer of reducing equivalents through the mitochondrial membrane requires substrate pairs ▪ linked by suitable dehydrogenases on each side of the mitochondrial membrane. The mechanism of transfer using the glycerophosphate shuttle. Present in some tissues (eg, brain, white muscle), in others (eg, heart muscle) it is deficient. Combination of 2 activities: ▪ The flow of electrons to O2 via ETC ▪ NADH + H+ + ½ O2 → H2O + NAD+ ▪ Down hill flow of electrons release large amount of E. ▪ Drive another process. ▪ Phosphorylation of ADP by Pi synthesizing ATP ▪ ADP + Pi ATP + H2O ▪ Catalyzed by ATP synthase. Mitchell hypothesis ▪ ETC and oxidative phosphorylation are coupled by a proton gradient across the inner mitochondria membrane ▪ The proton motive force caused by the electrochemical potential difference (negative on the matrix side) drives the mechanism of ATP synthesis. Each of the respiratory chain complexes I, III, and IV acts as a proton pump. Inner mitochondria membrane is permeable to protons: ▪ Accumulate in intermembrane space: creates a PROTON GRADIENT. ▪ Electrochemical potential difference across the membrane. Electrochemical potential ▪ Consists of: ▪ a chemical potential (difference in pH). ▪ an electrical potential. Chemiosmotic hypothesis ▪ Protons re-enter matrix. ▪ Dissipating the pH & electrical gradients. Once established, inhibits further transport of reducing equivalents through the respiratory chain. Discharged by back translocation of protons through ATP synthase ▪ depends on availability of ADP and Pi. Synthesizes ATP in the presence of Pi + ADP. Consist of several protein subunits: ▪ F1 ▪ projects into the matrix ▪ contain the phosphorylation mechanism. ▪ three α- and three β-subunits ▪ F0 ▪ consists of several protein subunits ▪ spans the membrane ▪ forms the proton channel. ▪ γ-subunit. ▪ Protons flow through F0 –F1 ▪ Cause the F0 causes to rotate ▪ drive ATP production in the F1 complex ▪ ADP and Pi are taken up sequentially by the β-subunits to form ATP. The respiratory chain provides most of the energy captured during catabolism Approximately 68% of the free energy from the oxidation of foodstuffs captured. The remaining free energy - liberated as heat. Availability of ADP Oxidation and phosphorylation are tightly coupled ▪ oxidation cannot proceed via the respiratory chain without phosphorylation of ADP. ▪ Energy metabolism-maintain constant ATP level. ▪ Electrons flow down ETC to O2 in the presence of ADP for phosphorylation. ▪ [ADP] regulate the electrons flow. ▪ Level of ADP triggers increase in rate of catabolic reactions. Availability of ADP When work is performed, ATP is converted to ADP, allowing more respiration to occur - replenishes the store of ATP. Concentration of inorganic phosphate. Rate of respiration increases ▪ If increase - as in exercise ▪ the capacity of the respiratory chain becomes saturated ▪ or the PO2 decreases below the Km for cytochrome a3. ADP/ATP transporter ▪ facilitates entry of cytosolic ADP into and ATP out of the mitochondrion might also becomes ratelimiting. Transporter systems in the inner mitochondrial membrane. 1 , phosphate transporter; 2 , pyruvate symport; 3 , dicarboxylate transporter; 4 , tricarboxylate transporter; 5 , α-ketoglutarate transporter; 6 , adenine nucleotide transporter. Also present (but not shown) are transporter systems for glutamate/aspartate , glutamine, ornithine, neutral amino acids, and carnitine. Uncoupling by ▪ uncoupling protein/thermogenin – animals ▪ uncoupling agent-2,4-dinitrophenol (dieting aid). Provides a channel to return pumped protons from intermembrane space to matrix. Proton flow bypass ATP synthase ▪ Energy release as heat instead of ATP. Site specific inhibitors of electron transport.