Chapter 9. Molecular Structure and Replication of Genetic Material PDF
Document Details
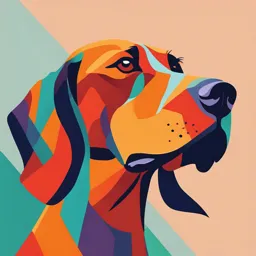
Uploaded by BreathtakingSerpent
University of Anbar
Tags
Related
Summary
This chapter covers the molecular structure and replication of genetic material, highlighting DNA and RNA. It discusses the identification of DNA as the genetic material, using classic experiments like those of Griffith and Avery, MacLeod, and McCarty. The chapter also examines the organization of genes and their expression.
Full Transcript
PA RT I I I MOLECULAR STRUCTURE AND REPLICATION OF THE GENETIC MATERIAL CHAPTER OUTLINE 9.1 Identification of DNA as the Genetic Material 9.2 Overview of DNA and RNA Structure 9.3 Nucleotide Structure 9.4 Structure of a DNA Strand 9.5 Discovery of the Double Helix 9.6...
PA RT I I I MOLECULAR STRUCTURE AND REPLICATION OF THE GENETIC MATERIAL CHAPTER OUTLINE 9.1 Identification of DNA as the Genetic Material 9.2 Overview of DNA and RNA Structure 9.3 Nucleotide Structure 9.4 Structure of a DNA Strand 9.5 Discovery of the Double Helix 9.6 Structure of the DNA Double Helix 9 9.7 RNA Structure A molecular model showing the structure of the DNA double helix. ©Scott Camazine/123RF MOLECULAR STRUCTURE OF DNA AND RNA In Chapters 2 through 8, we focused on the relationship between 9.1 IDENTIFICATION OF DNA AS the inheritance of genes and chromosomes and the outcome of an organism’s traits. In this chapter, we will shift our attention to THE GENETIC MATERIAL molecular genetics—the study of DNA structure and function at Learning Outcomes: the molecular level. An exciting goal of molecular genetics is to 1. List the four criteria that the genetic material must meet. use our knowledge of DNA structure to understand how DNA 2. Analyze the results of (1) Griffith, (2) Avery, MacLeod, and functions as the genetic material. Using molecular techniques, re- McCarty, and (3) Hershey and Chase, and explain how they searchers have determined the organization of many genes. This indicate that DNA is the genetic material. information, in turn, has helped us understand how the expression of genes governs the outcome of an individual’s inherited traits. To fulfill its role, the genetic material must meet four criteria: The past several decades have seen dramatic advances in 1. Information: The genetic material must contain the infor- techniques and approaches used to investigate and even to alter the mation necessary to construct an entire organism. In other genetic material. These advances have greatly expanded our un- words, it must provide the blueprint for determining the in- derstanding of molecular genetics and also have provided key in- herited traits of an organism. 2. Transmission: During reproduction, the genetic material sights into the mechanisms underlying transmission and population must be passed from parents to offspring. genetics. Molecular genetics technology is also widely used in 3. Replication: Because the genetic material is passed from supporting disciplines such as biochemistry, cell biology, and parents to offspring, and from mother cell to daughter cells microbiology. during cell division, it must be copied. To a large extent, our understanding of genetics comes from 4. Variation: Within any species, a significant amount of our knowledge of the molecular structure of DNA (deoxyribo- phenotypic variability occurs. For example, Mendel studied several characteristics in pea plants that varied among dif- nucleic acid) and RNA (ribonucleic acid). In this chapter, we ferent strains. These included height (tall versus dwarf) and will begin by considering classic experiments that showed that seed color (yellow versus green). Therefore, the genetic DNA is the genetic material. We will then survey the molecular material must also vary in ways that can account for the features of DNA and RNA that underlie their function. known phenotypic differences within each species. 210 9.1 IDENTIFICATION OF DNA AS THE GENETIC MATERIAL 211 In the nineteenth century, the data of many geneticists, strains of S. pneumoniae secrete a polysaccharide capsule, whereas including Mendel, were consistent with these four properties of other strains do not. When streaked onto petri plates containing a genetic material. However, the experimental study of genetic solid growth medium, capsule-secreting strains produce colonies crosses cannot, by itself, identify the chemical nature of the genetic with a smooth morphology, whereas those strains unable to secrete material. a capsule produce colonies with a rough appearance. In the 1880s, August Weismann and Carl Nägeli champi- The different forms of S. pneumoniae also vary in their viru- oned the idea that a chemical substance within living cells is lence, or ability to cause disease. When smooth strains of S. pneu- responsible for the transmission of traits from parents to offspring. moniae infect a mouse, the capsule allows the bacteria to escape The chromosome theory of inheritance was developed, and attack by the mouse’s immune system. As a result, the bacteria can experimentation demonstrated that the chromosomes are the carri- grow and eventually kill the mouse. In contrast, the nonencapsulated ers of the genetic material (see Chapter 3). Nevertheless, the story (rough) bacteria are destroyed by the animal’s immune system. was not complete because chromosomes contain both DNA and In 1928, Griffith conducted experiments that involved the proteins. Also, RNA is found in the vicinity of chromosomes. injection of live and/or heat-killed bacteria into mice. He then Therefore, further research was needed to precisely identify the observed whether or not the bacteria caused a lethal infection. genetic material. In this section, we will examine the first experi- Griffith was working with two strains of S. pneumoniae, a type S mental attempts to achieve this goal. (for “smooth”) and a type R (for “rough”). ∙ When injected into a live mouse, the type S bacteria prolif- Griffith’s Experiments Indicated That erated within the mouse’s bloodstream and ultimately Genetic Material Can Transform Streptococcus killed the mouse (Figure 9.1a). Following the death of the Some early work in microbiology was important in developing an mouse, Griffith found many type S bacteria within the experimental strategy for identifying the genetic material. Freder- mouse’s blood. ick Griffith studied a type of bacterium known then as pneumo- ∙ In contrast, when type R bacteria were injected into a cocci and now classified as Streptococcus pneumoniae. Certain mouse, the mouse lived (Figure 9.1b). Living type S bacteria were Living type R bacteria were Heat-killed type S bacteria Living type R and heat-killed injected into a mouse. injected into a mouse. were injected into a mouse. type S bacteria were injected into a mouse. Live type R Dead type S After After After After several several several several days days days days Mouse died. Mouse survived. Mouse survived. Mouse died. Type S bacteria were isolated No living bacteria were isolated No living bacteria were isolated Type S bacteria were isolated from the dead mouse. from the mouse. from the mouse. from the dead mouse. (a) Live type S (b) Live type R (c) Dead type S (d) Live type R + dead type S F I G U R E 9. 1 Griffith’s experiments on genetic transformation in S. pneumoniae. CONCEPT CHECK: Explain why the mouse in part (d) died. 212 C H A P T E R 9 :: MOLECULAR STRUCTURE OF DNA AND RNA ∙ To verify that the proliferation of the smooth bacteria was type S. However, if no DNA extract was added, no type S bacterial causing the death of the mouse, Griffith killed the smooth colonies were observed on the petri plates. bacteria with heat treatment before injecting them into a A biochemist might point out that a DNA extract may not be mouse. In this case, the mouse also survived (Figure 9.1c). 100% pure. In fact, any purified extract might contain small traces ∙ The critical and unexpected result was obtained in the ex- of some other substances. Therefore, one can argue that a small periment shown in Figure 9.1d. In this experiment, live amount of contaminating material in the DNA extract might actu- type R bacteria were mixed with heat-killed type S bacte- ally be the genetic material. The most likely contaminating sub- ria. Several days after receiving this mixture, the mouse stance in this case would be RNA or protein. To further verify that died. Furthermore, extracts from tissues of the dead mouse the DNA in the extract was responsible for the transformation, were found to contain living type S bacteria! Avery, MacLeod, and McCarty treated samples of the DNA ex- tract with enzymes that digest DNA (called DNase), RNA What can account for these results? Because living type R bacteria (RNase), or protein (protease) (see Figure 9.2). alone could not proliferate and kill the mouse (Figure 9.1b), the interpretation of the result in Figure 9.1d is that something from ∙ When the DNA extracts were treated with RNase or prote- the dead type S bacteria was transforming the type R bacteria ase, they still converted type R bacteria into type S. These into type S bacteria. Griffith called this process transformation, results indicated that any RNA or protein in the extract was and the unidentified substance causing this to occur was termed not acting as the genetic material. the transforming principle. The steps of bacterial transformation ∙ However, when the extract was treated with DNase, it lost are described in Chapter 7 (look back at Figure 7.11). its ability to convert type R into type S bacteria. At this point, let’s look at what Griffith’s observations mean These results indicated that the degradation of the DNA in the in genetic terms. The transformed bacteria acquired the informa- extract by DNase prevented conversion of type R to type S. This tion to make a capsule. Among different strains, variation exists in interpretation is consistent with the hypothesis that DNA is the the ability to create a capsule and to cause mortality in mice. The genetic material. A more elegant way of saying this is that “DNA genetic material that is necessary to create a capsule must be rep- is the transforming principle.” licated so it can be transmitted from mother to daughter cells dur- ing cell division. Taken together, these observations are consistent with the idea that the formation of a capsule is governed by the Hershey and Chase Provided Evidence That DNA bacteria’s genetic material, meeting the four criteria described pre- Is the Genetic Material of T2 Phage viously. Griffith’s experiments showed that some genetic material from the dead bacteria had been transferred to the living bacteria A second set of experimental results indicating that DNA is the and provided them with a new trait. However, Griffith did not genetic material came from the studies of Alfred Hershey and know what the transforming substance was. Martha Chase in 1952. Their research centered on the study of a virus known as T2. This virus infects Escherichia coli bacterial cells and is therefore known as a bacteriophage, or simply, a Avery, MacLeod, and McCarty Showed That DNA phage. The structure of the T2 phage consists of genetic material Is the Substance That Transforms Bacteria that is packaged inside a phage coat. From a molecular Important scientific discoveries often take place when research- perspective, this phage is rather simple, because it is composed ers recognize that someone else’s experimental observations of only two types of macromolecules: DNA and proteins. During can be used to address a particular scientific question. Oswald infection, the phage coat remains attached on the outside of the Avery, Colin MacLeod, and Maclyn McCarty realized that bacterium and does not enter the cell. Only the genetic material Griffith’s observations could be used as part of an experimental of the phage enters the bacterial cell. strategy to identify the genetic material. They asked: What sub- Hershey and Chase asked: What is the biochemical compo- stance is being transferred from the dead type S bacteria to the sition of the genetic material that enters the bacterial cell during live type R? infection? They used radioisotopes to distinguish proteins from At the time of these experiments in the 1940s, researchers DNA. Sulfur atoms are found in proteins but not in DNA, whereas already knew that DNA, RNA, proteins, and carbohydrates are phosphorus atoms are found in DNA but not in phage proteins. major constituents of living cells. To separate these components Therefore, 35S (a radioisotope of sulfur) and 32P (a radioisotope of and to determine if any of them was the genetic material, Avery, phosphorus) were used to specifically label phage proteins and MacLeod, and McCarty used established biochemical purification DNA, respectively. After phages were given sufficient time to in- procedures and prepared extracts from type S bacterial strains that fect bacterial cells, the researchers separated the phage coats from contained each type of these molecules. After many repeated at- the bacterial cells. They determined that most of the 32P had en- tempts with the different types of extracts, they discovered that tered the bacterial cells, whereas most of the 35S remained outside only one of the extracts, namely, the one that contained purified the cells. These results were consistent with the idea that the ge- DNA from type S bacteria, was able to convert type R bacteria netic material of bacteriophages is DNA, not proteins. into type S. As shown in Figure 9.2, when this extract was mixed We now know that bacteria, archaea, protists, fungi, plants, with type R bacteria, some of the bacteria were converted to and animals all use DNA as their genetic material. Many viruses, 9.2 OVERVIEW OF DNA AND RNA STRUCTURE 213 1 2 3 4 5 Type R Type R Type S Type R Type S Type R Type S Type R Type S cells cells DNA cells DNA cells DNA cells DNA extract extract extract extract + + + DNase RNase protease Mix Mix Mix Mix Allow sufficient time for the DNA to be taken up by the type R bacteria. Only a small percentage of the type R bacteria will be transformed to type S. Add an antibody that aggregates type R bacteria (that have not been transformed). The aggregated bacteria are removed by gentle centrifugation. Plate the remaining bacteria on petri plates. Incubate overnight. Transformed Transformed Transformed F I G U R E 9. 2 Experiments of Avery, MacLeod, and McCarty to identify the transforming principle. Samples of S. pneumoniae cells were either not exposed to a type S DNA extract (experiment 1, left side) or exposed to a type S DNA extract (experiments 2–5). Extracts used in experi- ments 3, 4, and 5 also contained DNase, RNase, or protease, respectively. After incubation, the cells were exposed to antibodies, which are molecules that can specifically recognize the molecular structure of macromolecules. In this experiment, the antibodies recognized the cell surface of type R bacteria and caused the bacteria to clump together. The clumped bacteria were removed by a gentle centrifugation step. Only the bacteria that were not recognized by the antibody (namely, the type S bacteria) remained in the supernatant. The cells in the supernatant were plated on solid growth media. After overnight incubation, visible colonies may be observed. CONCEPT CHECK: What was the purpose of adding RNase or protease to a DNA extract? such as T2, also use DNA as their genetic material. However, as discussed in Chapter 18, the genetic material of some viruses is 9.2 OVERVIEW OF DNA AND RNA, rather than DNA. RNA STRUCTURE Learning Outcomes: 9.1 COMPREHENSION QUESTION 1. Define nucleic acid. 2. Describe the four levels of complexity of DNA. 1. In the experiment of Avery, McLeod, and McCarty, the addition of RNase or protease to a DNA extract DNA and its molecular cousin, RNA, are known as nucleic acids. a. prevented the conversion of type S bacteria into type This term is derived from the discovery of DNA by Friedrich R bacteria. Miescher in 1869. He identified a previously unknown phosphorus- b. allowed the conversion of type S bacteria into type containing substance that was isolated from the nuclei of white blood R bacteria. cells found in waste surgical bandages. He named this substance c. prevented the conversion of type R bacteria into type nuclein. As the structure of DNA and RNA became better under- S bacteria. stood, it was determined that they are acidic molecules, which means d. allowed the conversion of type R bacteria into type they release hydrogen ions (H+) in solution and have a net negative S bacteria. charge at neutral pH. Thus, the name nucleic acid was coined. 214 C H A P T E R 9 :: MOLECULAR STRUCTURE OF DNA AND RNA C 9.3 NUCLEOTIDE STRUCTURE G A T Nucleotides Learning Outcomes: 1. Describe the structure of a nucleotide. A G A A G A A T C C T C C G A A T 2. Compare and contrast the structures of nucleotides found in T C C DNA and in RNA. Single strand The nucleotide is the repeating structural unit of both DNA and C A G T C A G T G T T G T RNA. A nucleotide has three components: at least one phosphate A C C T A G A T A group, a pentose sugar, and a nitrogenous base. As shown in G A T A Figure 9.4, nucleotides vary with regard to the sugar and the Double helix nitrogenous base. ∙ The two types of sugars are deoxyribose and ribose, which are found in DNA and RNA, respectively. ∙ The five different bases are subdivided into two catego- ries: the purines and the pyrimidines. ∙ The purine bases, adenine (A) and guanine (G), contain a double-ring structure; the pyrimidine bases, thymine (T), cytosine (C), and uracil (U), contain a single-ring structure. Three-dimensional structure ∙ The base thymine is found in DNA, whereas uracil is found in RNA instead of thymine. Adenine, guanine, and cyto- F I G U RE 9.3 Levels of nucleic acid structure. sine occur in both DNA and RNA. As shown in Figure 9.4, the bases and sugars have a standard num- bering system. The nitrogen and carbon atoms found in the ring structures of the bases are given numbers 1 through 9 for the pu- Geneticists, biochemists, and biophysicists have been inter- rines and 1 through 6 for the pyrimidines. In comparison, the num- ested in the molecular structure of nucleic acids for several de- bers given to the five carbons found in the sugars have primes, as cades. Both DNA and RNA are macromolecules composed of in 1′, to distinguish them from the numbers used for the bases. smaller building blocks. To fully appreciate their structures, we Figure 9.5 shows the repeating units of nucleotides found in need to consider four levels of complexity (Figure 9.3): DNA and RNA. The locations of the attachment sites of the base and phosphate to the sugar molecule are important to the nucleotide’s 1. Nucleotides form the repeating structural unit of nucleic function. In the sugar ring, carbon atoms are numbered in a clockwise acids. direction, beginning with a carbon atom adjacent to the ring’s oxygen 2. Nucleotides are linked together in a linear manner to form atom. The fifth carbon is outside the ring structure. In a single nucleo- a strand of DNA or RNA. tide, the base is always attached to the 1′ carbon atom, and one or 3. Two strands of DNA (or sometimes strands of RNA) inter- more phosphate groups are attached at the 5′ position. As discussed act with each other to form a double helix. later, the —OH group attached to the 3′ carbon is important in allow- 4. The three-dimensional structure of DNA results from the ing nucleotides to form covalent linkages with each other. folding and bending of the double helix. Within living The terminology used to describe nucleic acid units is based cells, DNA is associated with a wide variety of proteins on three structural features: the type of sugar, the type of base, and that influence its structure. Chapter 10 examines the roles the number of phosphate groups. When a sugar is attached to only of these proteins in creating the three-dimensional structure a base, this pair is a nucleoside. If ribose is attached to adenine, of DNA found within chromosomes. this nucleoside is called adenosine (Figure 9.6). Nucleosides composed of ribose and guanine, cytosine, or uracil are named guanosine, cytidine, and uridine, respectively. Nucleosides made of deoxyribose and adenine, guanine, thymine, or cytosine are 9.2 COMPREHENSION QUESTION called deoxyadenosine, deoxyguanosine, deoxythymidine, and de- oxycytidine, respectively. 1. Going from simple to complex, which of the following is the The covalent attachment of one or more phosphate mole- proper order for the structure of DNA? cules to a nucleoside creates a nucleotide. One or more phosphate a. Nucleotide, double helix, DNA strand, chromosome groups are attached to a sugar via an ester bond. If a nucleotide b. Nucleotide, chromosome, double helix, DNA strand contains ribose, adenine, and one phosphate, it is named adenos- c. Nucleotide, DNA strand, double helix, chromosome ine monophosphate, abbreviated AMP. A nucleotide composed of d. Chromosome, nucleotide, DNA strand, double helix ribose, adenine, and three phosphate groups is called adenosine 9.3 NUCLEOTIDE STRUCTURE 215 Bases Phosphate group Sugars Purines Pyrimidines (double ring) (single ring) 5′ NH2 O O HOCH2 O OH H H N 6 CH3 4 H 4 1′ 7 5 1 N 5 3N 5 3N 4′ H H H 8 H H 9 4 2 6 2 6 2 3 1 1 N H H N O H N O 3′ 2′ N O– HO H H H H D-Deoxyribose (in DNA) O P O Adenine (A) Thymine (T) (in DNA) Uracil (U) (in RNA) O– O NH2 5′ N 6 H H HOCH2 OH 4 O 1N 5 3N 7 5 4′ 1′ H 8 2 H H 9 4 6 2 H H N 3 NH NH H 1 O N 2 N 3′ 2′ HO OH H H D-Ribose (in RNA) Guanine (G) Cytosine (C) FIG U R E 9.4 The components of nucleotides. The three building blocks of a nucleotide are one or more phosphate groups, a sugar, and a base. The bases are categorized as purines (adenine and guanine) and pyrimidines (thymine, cytosine, and uracil). Note: The location of a double bond in a phosphate group is not fixed. Because the sharing of electrons between phosphorus and the oxygen atoms is delocalized, phosphate exists as multiple resonance structures. CONCEPT CHECK: Which of these components of nucleotides are not found in DNA? Adenosine triphosphate (nucleotide) Adenosine diphosphate (nucleotide) O O Adenosine monophosphate (nucleotide) Base Base Adenosine (nucleoside) O– P O CH2 O– P O CH2 5′ O 5′ O O 4′ 1′ O 4′ 1′ H H H H Adenine NH2 H H H H Phosphate Phosphate 3′ 2′ 3′ 2′ N N OH H OH OH H Deoxyribose Ribose O– O– O– N H N (a) Repeating unit of (b) Repeating unit of deoxyribonucleic ribonucleic acid (RNA) O– P O P O P O CH2 O 5′ acid (DNA) O O O 4′ 1′ H H H H FIGURE 9.5 The structure of nucleotides found Phosphate groups 3′ 2′ in (a) DNA and (b) RNA. DNA contains deoxyribose as HO OH its sugar and the bases A, T, G, and C. RNA contains ribose Ribose as its sugar and the bases A, U, G, and C. In a DNA or RNA strand, the oxygen on the 3′ carbon of the sugar is linked to the phos- F IG U RE 9. 6 A comparison of the structures of phorus atom of the phosphate in the adjacent nucleotide. The two atoms an adenine-containing nucleoside and nucleotides. (O and H) shown in red are found within individual nucleotides but not when nucleotides are joined together to make strands of DNA and RNA. T OPIC: What topic in genetics does this question address? The triphosphate, or ATP. By comparison, a nucleotide made of de- topic is the structure of nucleosides and nucleotides. oxyribose, adenine, and three phosphate groups is referred to as deoxyadenosine triphosphate (dATP). I NFORMATION: What information do you know based on the question and your understanding of the topic? In the question, you are given the components of a molecule. From your GENETIC TIPS THE QUESTION: A molecule contains understanding of the topic, you may remember that a nucleoside and a nucleotide differ with regard to their phosphate content, adenine, deoxyribose, and one phosphate. Is it a nucleoside or a nucleotide? Would it be found in DNA or RNA? whereas the nucleotides found in DNA and RNA differ with regard to their sugars and the presence of thymine or uracil. 216 C H A P T E R 9 :: MOLECULAR STRUCTURE OF DNA AND RNA Backbone Bases P ROBLEM-SOLVING S TRATEGY: Define key terms. Compare O and contrast. A nucleoside is composed of a sugar and a base, 5′ CH3 H whereas a nucleotide has a sugar, a base, and one or more phosphate N groups. Compare the components found in DNA and RNA. Thymine (T) H N O O– ANSWER: The molecule is a nucleotide. It is composed of a sugar, O P O CH2 5′ O a base, and a phosphate. Because it contains deoxyribose, it could be O– 4′ 1′ H H found in DNA, but not in RNA. H H 3′ 2′ NH2 H N N Phosphodiester Ester H Adenine (A) 9.3 COMPREHENSION QUESTIONS linkage O bonds N N H O P O CH2 1. Which of the following could be the components of a single nu- 5′ O O– cleotide found in DNA? 4′ H H H H 1′ a. Deoxyribose, adenine, and thymine 3′ 2′ NH2 H b. Ribose, phosphate, and cytosine H N Cytosine (C) c. Deoxyribose, phosphate, and thymine H O d. Ribose, phosphate, and uracil O N O P O CH2 2. A key difference between the nucleotides found in DNA and 5′ O O– those in RNA is that 4′ H H H H 1′ Guanine (G) a. those in DNA have phosphate, and those in RNA do not. 3′ 2′ O H b. those in DNA have deoxyribose, and those in RNA have ribose. N H N c. those in DNA have thymine, and those in RNA have uracil. H d. Both b and c are correct. O N N NH NH2 Single O P O CH2 5′ O DNA O– 9.4 STRUCTURE OF A DNA 4′ 1′ nucleotide H H Phosphate H H STRAND 3′ 2′ OH H Sugar (deoxyribose) Learning Outcome: 3′ 1. Describe the structural features of a DNA strand. FIGURE 9.7 A short strand of DNA containing four nucleotides. Nucleotides are covalently linked together to form a strand of DNA. CONCEPT CHECK: Which components of nucleotides form the backbone of A strand of DNA (or RNA) has nucleotides that are linked to each a DNA strand? other in a linear fashion. Figure 9.7 depicts a short strand of DNA with four nucleotides. A few structural features are worth noting. ∙ A phosphate group connects two sugar molecules via two for the sequence is written 5′–TACG–3′. The nucleotides within a ester bonds. For this reason, the linkage in DNA (or RNA) strand are covalently attached to each other via phosphodiester strands is called a phosphodiester linkage. linkages, so the sequence of bases cannot shuffle around and ∙ The phosphates and sugar molecules form the backbone become rearranged. Therefore, the sequence of bases in a DNA of the strand. The bases project from the backbone. The strand remains the same over time, except in rare cases when backbone is negatively charged due to the negative charge mutations occur. As we will see throughout this textbook, the on each phosphate. sequence of bases within DNA and RNA is the defining feature ∙ A phosphodiester linkage involves attachment of a phos- that allows them to carry information. phate to the 3′ carbon in one nucleotide and to the 5′ carbon in the next nucleotide. In a strand, all sugar molecules are oriented in the same direction. Therefore, a strand has a 9.4 COMPREHENSION QUESTION directionality because all sugar molecules have the same orientation. In Figure 9.7, the direction of the strand is 5′ 1. In a DNA strand, a phosphate connects a 3′ carbon atom in one to 3′ proceeding from top to bottom. deoxyribose to a. a 5′ carbon in an adjacent deoxyribose. A critical aspect regarding DNA and RNA structure is that a strand contains a specific sequence of bases. In Figure 9.7, the sequence b. a 3′ carbon in an adjacent deoxyribose. of bases is thymine–adenine–cytosine–guanine, abbreviated c. a base in an adjacent nucleotide. TACG. Furthermore, to show the directionality, the abbreviation d. none of the above. 9.5 DISCOVERY OF THE DOUBLE HELIX 217 9.5 DISCOVERY OF THE DOUBLE HELIX Learning Outcome: 1. Outline the key experiments that led to the discovery of the DNA double helix. A major discovery in molecular genetics was made in 1953 by James Watson and Francis Crick. At that time, DNA was already known to be composed of nucleotides. However, it was not under- stood how the nucleotides are bonded together to form the struc- (a) Rosalind Franklin ture of DNA. Watson and Crick committed themselves to determining the structure of DNA because they felt this knowl- edge was needed to understand the functioning of genes. Other researchers, such as Rosalind Franklin and Maurice Wilkins, shared this view. Before we examine the characteristics of the double helix, let’s consider the events that provided the scientific X-rays diffracted by DNA framework for Watson and Crick’s breakthrough. Wet DNA fibers A Few Key Events Led to the Discovery of the X-ray beam Double-Helix Structure One method that proved important in the discovery of the struc- ture of the DNA double helix was model building. In the early The pattern represents the atomic array in wet fibers. H (b) X-ray diffraction of wet DNA fibers C C N F IG U RE 9. 9 X-ray diffraction of DNA fibers. H O C H C (a): ©World History Archive/Alamy N C C N O Carbonyl C oxygen 1950s, Linus Pauling proposed that regions of proteins can fold O H into a secondary structure known as an α helix (Figure 9.8a). To C H Amide O N C hydrogen elucidate this structure, Pauling built large models by linking to- C N gether simple ball-and-stick units (Figure 9.8b). By carefully O C scaling the objects in his models, he could visualize whether at- H H C C N oms fit together properly in a complex three-dimensional struc- N C O C ture. As we will see, Watson and Crick also used ball-and-stick HO Hydrogen modeling to solve the structure of the DNA double helix. Interest- C H bond ingly, they were well aware that Pauling might figure out the struc- O N C C N ture of DNA before they did. This stimulated a rivalry between the O C researchers. It is worth noting that the use of models is still an H H C important tool in understanding structural features of biomole- C N N C O cules. However, modern-day molecular geneticists usually con- O struct their three-dimensional models on computers. A second development that led to the elucidation of the dou- (a) An α helix in a protein (b) Linus Pauling ble helix was improvement in the use of X-ray diffraction. When a purified substance, such as DNA, is subjected to X-rays, it pro- F I G U R E 9. 8 Linus Pauling and the α-helix protein structure. (a) An α helix is a secondary structure found in proteins. The polypep- duces a well-defined diffraction pattern if the molecular structure tide backbone is shown as a tan ribbon. Hydrogen bonding between has a repeating pattern. An interpretation of the diffraction pattern hydrogen and oxygen atoms stabilizes the helical conformation. (using mathematical theory) can ultimately provide information (b) Pauling with a ball-and-stick model. concerning the structure of the molecule. Rosalind Franklin (b): ©Tom Hollyman/Science Source (Figure 9.9a), working in the same laboratory as Maurice Wilkins, 218 C H A P T E R 9 :: MOLECULAR STRUCTURE OF DNA AND RNA used X-ray diffraction to study wet DNA fibers. Franklin made ∙ The pattern was consistent with a helical structure. marked advances in X-ray diffraction techniques while working ∙ The diameter of the helical structure was too wide to be with DNA. She adjusted her equipment to produce an extremely only a single-stranded helix. fine beam of X-rays. She extracted finer DNA fibers than ever ∙ The diffraction pattern indicated that the helix contains before and arranged them in parallel bundles. Franklin also stud- about 10 base pairs (bp) per complete turn. ied the fibers’ reactions to humid conditions. A diffraction pattern These observations were instrumental in solving the structure of of Franklin’s DNA fibers is shown in Figure 9.9b. It suggested DNA. several structural features of DNA. EXPERIMENT 9A Chargaff Found That DNA Has a Biochemical Chargaff’s experimental protocol is described in Figure 9.10. Composition in Which the Amounts of A and T He began with cells from various species as starting material. The chromosomes were extracted from cells and then treated Are Equal, As Are the Amounts of G and C with protease to separate the DNA from chromosomal proteins. Another piece of information that led to the discovery of the dou- The DNA was then treated with a strong acid, which cleaved ble-helix structure came from the studies of Erwin Chargaff. In the bonds between the sugars and bases, thereby releasing the the 1940s and 1950s, he pioneered many of the biochemical tech- individual bases from the DNA strands. This mixture of bases niques for the isolation, purification, and measurement of nucleic was then subjected to paper chromatography to separate the acids from living cells. This was no trivial undertaking, because four types. The amounts of the four bases were determined the biochemical composition of living cells is complex. At the spectroscopically. time of Chargaff’s work, researchers already knew that the build- ing blocks of DNA are nucleotides containing the bases adenine, thymine, guanine, or cytosine. Chargaff analyzed the base compo- T H E G O A L ( D I S C O V E R Y- B A S E D S C I E N C E ) sition of DNA, which was isolated from many different species. He expected that the results might provide important clues con- An analysis of the base composition of DNA in different organ- cerning the structure of DNA. isms may reveal important features about the structure of DNA. ACHIEVING THE GOAL F I G U R E 9. 1 0 An analysis of base composition among different DNA samples. Starting material: The following types of cells were obtained: Escherichia coli, Streptococcus pneumoniae, yeast, turtle red blood cells, salmon sperm cells, chicken red blood cells, and human liver cells. Experimental level Conceptual level Solution of DNA + 1. For each type of cell, extract the chromosomal proteins chromosomal material. This can be extract done in a variety of ways, including treatment with concentrated salt solution, detergent, or mild alkali. Note: The chromosomes contain both DNA and protein. Protease DNA 2. Remove the protein. This can be done in several ways, including treatment with protease. Individual Acid T bases 3. Hydrolyze the DNA to release the bases A from the DNA strands. A common way G C A to do this is by strong acid treatment. C several ways, including treatment with protease. Individual Acid T bases 3. Hydrolyze the DNA to release the bases A from the DNA strands. A common way G C A to do this is by strong acid treatment. C G T C G 4. Separate the bases by chromatography. AA A Paper chromatography provides an easy A A A way to separate the four types of bases. (The technique of chromatography is C C C C CC C described in Appendix A.) G G G G GG G 5. Extract bands from paper into solutions Origin and determine the amounts of each base T T by spectroscopy. Each base will absorb TT T T light at a particular wavelength. By examining the absorption profile of a sample of base, it is then possible to calculate the amount of the base. (Spectroscopy is described in Appendix A.) Spectrophotometer 6. Compare the base content in the DNA from different organisms. T H E DATA I N T E R P R E T I N G T H E DATA Base Content in the DNA from a Variety of Organisms* The data shown in the table are only a small sampling of Char- Percentage of Base Content gaff’s results. During the late 1940s and early 1950s, Chargaff (Based on Molarity) published many papers concerned with the chemical composition of DNA from biological sources. Hundreds of measurements were Organism Adenine Thymine Guanine Cytosine made. The compelling observation was that the amount of adenine E. coli 26.0 23.9 24.9 25.2 was similar to that of thymine, and the amount of guanine was S. pneumoniae 29.8 31.6 20.5 18.0 similar to that of cytosine. The idea that the amount of A in DNA Yeast 31.7 32.6 18.3 17.4 equals the amount of T, and the amount of G equals that of C, is Turtle red blood 28.7 27.9 22.0 21.3 known as Chargaff’s rule. cells These results were not sufficient to propose a model for the structure of DNA. However, they provided the important clue that Salmon sperm 29.7 29.1 20.8 20.4 DNA is structured so that each molecule of adenine interacts with Chicken red blood 28.0 28.4 22.0 21.6 a thymine, and each molecule of guanine interacts with a cytosine. cells A DNA structure in which A binds to T and G binds to C would Human liver cells 30.3 30.3 19.5 19.9 explain the equal amounts of A and T and of G and C observed in *When the base compositions from different tissues within the same species Chargaff’s experiments. As we will see, this observation became were measured, similar results were obtained. These data were compiled from crucial evidence that Watson and Crick used to elucidate the struc- several sources. See E. Chargaff and J. Davidson, Eds. (1995) The Nucleic ture of the double helix. Acids. Academic Press, New York. 219 220 C H A P T E R 9 :: MOLECULAR STRUCTURE OF DNA AND RNA Watson and Crick Deduced the Double-Helix the model, with the bases projecting toward each other. At first, a Structure of DNA structure was considered in which the bases form hydrogen bonds with the identical base in the opposite strand (A to A, T to T, G to Thus far, we have examined key pieces of information used to G, and C to C). However, the model building revealed that the determine the structure of DNA. In particular, the X-ray dif- bases could not fit together this way. The final hurdle was over- fraction work of Franklin suggested a helical structure com- come when it was realized that the hydrogen bonding of adenine posed of two or more strands with 10 bases per turn. In addition, to thymine was structurally similar to that of guanine to cytosine. Chargaff’s work indicated that the amounts of A and T are With interactions between A and T and between G and C, the ball- equal and so are the amounts of G and C. Furthermore, Watson and-stick model showed that the two strands fit together properly. and Crick were familiar with Pauling’s success in using ball- This ball-and-stick model, shown in Figure 9.11, was consistent and-stick models to deduce the secondary structure of proteins. with all of the known data regarding DNA structure. With these key observations, they set out to solve the structure For their work, Watson, Crick, and Wilkins were awarded of DNA. the 1962 Nobel Prize in physiology or medicine. The contribution Watson and Crick assumed that DNA is composed of nu- of Franklin to the discovery of the double helix was also critical cleotides linked together in a linear fashion. They also assumed and has been acknowledged in several books and articles. Franklin that the chemical linkage between two nucleotides is always the was independently trying to solve the structure of DNA. However, same. With these ideas in mind, they tried to build ball-and- Wilkins, who worked in the same laboratory, shared Franklin’s X- stick models that incorporated the known experimental observa- ray diffraction data with Watson and Crick, presumably without tions. During this time, Franklin had produced even clearer her knowledge. This important information helped them solve the X-ray diffraction patterns, which provided greater detail con- structure of DNA, which was published in the journal Nature in cerning the relative locations of the bases and backbone of April 1953. Though Franklin was not given credit in the original DNA. This major breakthrough suggested a two-strand interac- publication of the double-helix structure, her key contribution be- tion that was helical. came known in later years. Unfortunately, however, she died in In their model building, Watson and Crick’s emphasis 1958, and so could not share in the Nobel Prize because it is not shifted to models containing the two backbones on the outside of awarded posthumously. 9.5 COMPREHENSION QUESTIONS 1. Evidence that led to the discovery of the DNA double helix included a. the determination of structures using ball-and-stick models. b. the X-ray diffraction data of Franklin. c. the base composition data of Chargaff. d. all of the above. 2. Chargaff’s analysis of the base composition of DNA is consistent with base pairing between a. A and G, and T and C. b. A and A, G and G, T and T, and C and C. c. A and T, and G and C. d. A and C, and T and G. 9.6 STRUCTURE OF THE DNA DOUBLE HELIX Learning Outcomes: 1. Outline the key structural features of the DNA double helix. FIGURE 9.11 Watson and Crick and their model of the DNA 2. Compare and contrast B DNA and Z DNA. double helix. James Watson is on the left and Francis Crick on the right, next to the molecular model they originally proposed for the double helix. Each strand contains a sugar-phosphate backbone. Between the opposite As we have seen, the discovery of the DNA double helix required strands, A hydrogen bonds with T, and G hydrogen bonds with C. different kinds of evidence. In this section, we will examine the ©A. Barrington Brown/Science Source double-helix structure in greater detail. 9.6 STRUCTURE OF THE DNA DOUBLE HELIX 221 The Molecular Structure of the DNA Double Helix indicates that purines (A and G) always bond with pyrimi- Has Several Key Features dines (T and C). This pairing of bases keeps the width of the double helix relatively constant. The general structural features of the double helix are shown in ∙ Three hydrogen bonds occur between G and C but only Figure 9.12. two between A and T. For this reason, DNA sequences ∙ In a DNA double helix, two DNA strands are twisted with a high proportion of G and C tend to form more stable together around a common axis to form a structure that double-stranded structures. resembles a spiral staircase. ∙ The AT/GC rule allows us to predict the sequence in one ∙ This double-stranded structure is stabilized by base pairs DNA strand if the sequence in the opposite strand is known. (bp)—pairs of bases in opposite strands that are hydrogen For example, let’s consider a DNA strand with the sequence bonded to each other. If you count the bases along one strand, 5′–ATGGCGGATTT–3′. The opposite strand has to be once you reach 10, you have gone 360° around the axis of the 3′–TACCGCCTAAA–5′. Using genetic terms, we say that helix. The linear distance along a strand through such a com- these two sequences are complementary to each other. plete turn is 3.4 nm; each base pair traverses 0.34 nm. ∙ The direction of DNA strands is depicted in the inset to ∙ A distinguishing feature of the hydrogen bonding between Figure 9.12. Going from the top of this figure to the bottom, base pairs is its specificity. An adenine in one strand one strand is running in the 5′ to 3′ direction, and the other hydrogen bonds with a thymine in the opposite strand, or a strand is 3′ to 5′. This opposite orientation of the two DNA guanine hydrogen bonds with a cytosine. This AT/GC rule strands is referred to as an antiparallel arrangement. Key Features 2 nm 5′ P 3′ Two strands of DNA form a right-handed double helix. S P P A S The bases in opposite strands P S 5′ end 3′ end hydrogen bond according to the S G C P CH3 H H HO AT/GC rule. P S H N NH2 O T N H H H S G C P O – O N O– H O The 2 strands are antiparallel with O P O CH2 N A P S N H O CH2 O P O O regard to their 5′ to 3′ directionality. O– N O P S C H H H H S H There are ~10.0 nucleotides in each P S H strand per complete 360° turn of P H NH2 N H H O the helix. S P S O– A T S H C N H N G N H H H P O P O CH2 N N O– O O O H2N H C G S O– H H CH2 O P O S P H H O P T A S H H CH3 S P G C P N T O H 2N N H H S O N P S O P O CH2 O O H N A N H H H O– P O– H H N H O P H H H CH2 O P O P S One complete G S H O P S turn 3.4 nm C G P S H N O H 2N H P T A O S O P O CH2 N G N H N C H H S G C P O O– N N H H H P S H H NH2 O H H O– A T One nucleotide H O CH2 O P O S P S 0.34 nm OH H O– P S PS P 3′ end 5′ end S C G S P C G S S 5′ P 3′ FIGURE 9.12 Key features of the structure of the double helix. Note: In the drawing on the left and in the inset, the planes of the bases and sugars are shown parallel to the central axis of the double helix in order to show the hydrogen bonding between the bases. In an actual DNA molecule, the bases are rotated about 90°, so their planes are perpendicular to the helix’s axis, as shown in Figure 9.13a. CONCEPT CHECK: What holds the DNA strands together? 222 C H A P T E R 9 :: MOLECULAR STRUCTURE OF DNA AND RNA F I GU R E 9. 13 Two models of the double helix. (a) Ball-and-stick model of the double helix. The deoxyribose-phosphate backbone Minor is shown in detail, whereas the groove bases are depicted as flattened rectangles. (b) Space-filling model of the double helix. Minor (b): ©Laguna Design/Science Source groove CONCEPT CHECK: Describe the major and minor grooves. Major groove Major groove (a) Ball-and-stick model of DNA (b) Space-filling model of DNA Figure 9.13a is a schematic model that emphasizes certain the indentations where atoms of the bases are in contact with the molecular features of DNA structure. The bases in this model are water in the surrounding cellular fluid. The DNA helix has two depicted as flat rectangular structures that hydrogen bond to form grooves winding around its outer surface: a narrow minor groove base pairs. (The hydrogen bonds are represented by the dotted and a wider major groove. lines.) Although the bases are not actually rectangular, they do As we will discuss in lat