Protein Biosynthesis - A Detailed Overview
Document Details
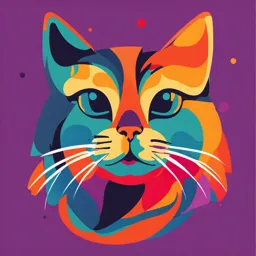
Uploaded by EasiestEuler
University of the Punjab
Tags
Summary
This document provides an overview of the biological process of protein biosynthesis. It details the stages involved, including transcription and translation, and explains the importance of protein folding and post-translational modifications. The document also touches on the role of protein biosynthesis in diseases.
Full Transcript
Protein biosynthesis E:\\800px-Summary\_of\_the\_protein\_biosynthesis\_process.png  **Protein biosynthesis** (or **protein synthesis**) is a core biological process, occurring inside [[cell...
Protein biosynthesis E:\\800px-Summary\_of\_the\_protein\_biosynthesis\_process.png  **Protein biosynthesis** (or **protein synthesis**) is a core biological process, occurring inside [[cells]](https://en.wikipedia.org/wiki/Cell_(biology)), [[balancing]](https://en.wikipedia.org/wiki/Homeostasis) the loss of cellular [[proteins]](https://en.wikipedia.org/wiki/Protein) (via [[degradation]](https://en.wikipedia.org/wiki/Proteolysis) or [[export]](https://en.wikipedia.org/wiki/Protein_targeting)) through the production of new proteins. Protein synthesis can be divided broadly into two phases - [[transcription]](https://en.wikipedia.org/wiki/Transcription_(biology)) and [[translation]](https://en.wikipedia.org/wiki/Translation_(biology)). - During transcription, a section of [[DNA]](https://en.wikipedia.org/wiki/DNA) encoding a protein, known as a [[gene]](https://en.wikipedia.org/wiki/Gene), is converted into a template molecule called [[messenger RNA]](https://en.wikipedia.org/wiki/Messenger_RNA) (mRNA). This conversion is carried out by enzymes, known as [[RNA polymerases]](https://en.wikipedia.org/wiki/RNA_polymerases), in the [[nucleus of the cell]](https://en.wikipedia.org/wiki/Cell_nucleus). In eukaryotes, this mRNA is initially produced in a premature form ([[pre-mRNA]](https://en.wikipedia.org/wiki/Primary_transcript)) which undergoes [[post-transcriptional modifications]](https://en.wikipedia.org/wiki/Post-transcriptional_modification) to produce [[mature mRNA]](https://en.wikipedia.org/wiki/Mature_messenger_RNA). The mature mRNA is exported from the cell nucleus via [[nuclear pores]](https://en.wikipedia.org/wiki/Nuclear_pore) to the [[cytoplasm]](https://en.wikipedia.org/wiki/Cytoplasm) of the cell for translation to occur. - During translation, the mRNA is read by [[ribosomes]](https://en.wikipedia.org/wiki/Ribosome) which use the [[nucleotide]](https://en.wikipedia.org/wiki/Nucleotide) sequence of the mRNA to determine the sequence of [[amino acids]](https://en.wikipedia.org/wiki/Amino_acid). The ribosomes catalyze the formation of [[covalent]](https://en.wikipedia.org/wiki/Covalent_bond) [[peptide bonds]](https://en.wikipedia.org/wiki/Peptide_bond) between the encoded amino acids to form a [[polypeptide chain]](https://en.wikipedia.org/wiki/Polypeptide_chain). **Folding of Polypeptide:** Following translation, the polypeptide chain must fold to form a functional protein; for example, to function as an enzyme the polypeptide chain must fold correctly to produce a functional [[active site]](https://en.wikipedia.org/wiki/Active_site). In order to adopt a functional three-dimensional (3D) shape, the polypeptide chain must first form a series of smaller underlying structures called [[secondary structures]](https://en.wikipedia.org/wiki/Protein_secondary_structure). The polypeptide chain in these secondary structures then folds to produce the overall 3D [[tertiary structure]](https://en.wikipedia.org/wiki/Protein_tertiary_structure). **Post-translational modifications:** Once correctly folded, the protein can undergo further maturation through different [[post-translational modifications]](https://en.wikipedia.org/wiki/Post-translational_modification). Post-translational modifications can alter the protein\'s ability to function, where it is located within the cell (e.g. cytoplasm or nucleus) and the protein\'s ability to [[interact with other proteins]](https://en.wikipedia.org/wiki/Protein-protein_interaction). **Errors in Proteins:** Protein biosynthesis has a key role in disease as changes and errors in this process, through underlying [[DNA mutations]](https://en.wikipedia.org/wiki/Mutation) or protein misfolding, are often the underlying causes of a disease. DNA mutations change the subsequent mRNA sequence, which then alters the mRNA encoded amino acid sequence. - [[Mutations can cause the polypeptide chain to be shorter]](https://en.wikipedia.org/wiki/Nonsense_mutation) by generating a [[stop sequence]](https://en.wikipedia.org/wiki/Stop_codon) which causes early termination of translation. - May [[changes the specific amino acid encoded at that position]](https://en.wikipedia.org/wiki/Missense_mutation) in the polypeptide chain. This amino acid change can impact the protein\'s ability to function or to fold correctly. Misfolded proteins are often implicated in disease as improperly folded proteins have a tendency to stick together to form [[dense protein clumps]](https://en.wikipedia.org/wiki/Protein_aggregation). These clumps are linked to a range of diseases, often [[neurological]](https://en.wikipedia.org/wiki/Neurological_disorder), including [[Alzheimer\'s disease]](https://en.wikipedia.org/wiki/Alzheimer%27s_disease) and [[Parkinson\'s disease]](https://en.wikipedia.org/wiki/Parkinson%27s_disease). **Transcription:**Transcription occurs in the nucleus using DNA as a template to produce mRNA. In eukaryotes, this mRNA molecule is known as pre-mRNA as it undergoes post-transcriptional modifications in the nucleus to produce a mature mRNA molecule. However, in prokaryotes post-transcriptional modifications are not required so the mature mRNA molecule is immediately produced by transcription. **Template DNA:** Initially, an enzyme known as a [[helicase]](https://en.wikipedia.org/wiki/Helicase) acts on the molecule of DNA. DNA has an [[antiparallel]](https://en.wikipedia.org/wiki/Antiparallel_(biochemistry)), double helix structure composed of two, complementary [[polynucleotide]](https://en.wikipedia.org/wiki/Polynucleotide) strands, held together by [[hydrogen bonds]](https://en.wikipedia.org/wiki/Hydrogen_bond) between the base pairs. The helicase disrupts the hydrogen bonds causing a region of DNA - corresponding to a gene - to unwind, separating the two DNA strands and exposing a series of bases. Despite DNA being a double stranded molecule, only one of the strands acts as a template for pre-mRNA synthesis - this strand is known as the template strand. The other DNA strand (which is [[complementary]](https://en.wikipedia.org/wiki/Complementarity_(molecular_biology)) to the template strand) is known as the coding strand. The coding strand of DNA runs in a 5\' to 3\' direction and the complementary, template DNA strand runs in the opposite direction from 3\' to 5\'. Two strands of DNA separated with an RNA polymerase attached to one of the strands and an RNA molecule coming out of the RNA polymerase Illustrates the conversion of the template strand of DNA to the pre-mRNA molecule by RNA polymerase. **Synthesis of mRNA:** The enzyme [[RNA polymerase]](https://en.wikipedia.org/wiki/RNA_polymerase) binds to the exposed template strand and reads from the gene in the 3\' to 5\' direction. Simultaneously, the RNA polymerase synthesizes a single strand of pre-mRNA in the 5\'-to-3\' direction by catalysing the formation of [[phosphodiester bonds]](https://en.wikipedia.org/wiki/Phosphodiester_bonds) between activated nucleotides (free in the nucleus) that are capable of complementary [[base pairing]](https://en.wikipedia.org/wiki/Base_pair) with the template strand. Behind the moving RNA polymerase the two strands of DNA rejoin, so only 12 base pairs of DNA are exposed at one time. RNA polymerase builds the pre-mRNA molecule at a rate of 20 nucleotides per second enabling the production of thousands of pre-mRNA molecules from the same gene in an hour. **Proofreading:** Despite the fast rate of synthesis, the RNA polymerase enzyme contains its own proofreading mechanism. The proofreading mechanisms allows the RNA polymerase to remove incorrect nucleotides (which are not complementary to the template strand of DNA) from the growing pre-mRNA molecule through an excision reaction. **Termination:** When RNA polymerase reaches a specific DNA sequence which [[terminates]](https://en.wikipedia.org/wiki/Stop_codon) transcription, RNA polymerase detaches and pre-mRNA synthesis is complete. The pre-mRNA molecule synthesized is complementary to the template DNA strand and shares the same nucleotide sequence as the coding DNA strand. However, there is one crucial difference in the nucleotide composition of DNA and mRNA molecules. DNA is composed of the bases - [[guanine]](https://en.wikipedia.org/wiki/Guanine), [[cytosine]](https://en.wikipedia.org/wiki/Cytosine), [[adenine]](https://en.wikipedia.org/wiki/Adenine) and [[thymine]](https://en.wikipedia.org/wiki/Thymine) (G, C, A and T) - RNA is also composed of four bases - guanine, cytosine, adenine and [[uracil]](https://en.wikipedia.org/wiki/Uracil). In RNA molecules, the DNA base thymine is replaced by uracil which is able to base pair with adenine. Therefore, in the pre-mRNA molecule, all complementary bases which would be thymine in the coding DNA strand are replaced by uracil. **Post-transcriptional modifications**  **Post-transcriptional modifications:** Once transcription is complete, the pre-mRNA molecule undergoes [[post-transcriptional modifications]](https://en.wikipedia.org/wiki/Post-transcriptional_modification) to produce a mature mRNA molecule. Following are three post-transcriptional modifications: 1. **Addition of a [[5\' cap]](https://en.wikipedia.org/wiki/Five-prime_cap):** The 5\' cap is added to the 5\' end of the pre-mRNA molecule and is composed of a guanine nucleotide modified through [[methylation]](https://en.wikipedia.org/wiki/Protein_methylation). The purpose of the 5\' cap is to prevent break down of mature mRNA molecules before translation, the cap also aids binding of the ribosome to the mRNA to start translation  and enables mRNA to be differentiated from other RNAs in the cell. **Addition of a 3\' [[poly(A) tail]](https://en.wikipedia.org/wiki/Polyadenylation):** The 3\' Poly(A) tail is added to the 3\' end of the mRNA molecule and is composed of 100-200 adenine bases. These distinct mRNA modifications enable the cell to detect that the full mRNA message is intact if both the 5\' cap and 3\' tail are present. 2. 3. **Removal of [[introns]](https://en.wikipedia.org/wiki/Intron) via [[RNA splicing]](https://en.wikipedia.org/wiki/RNA_splicing):** The modified pre-mRNA molecule then undergoes the process of RNA splicing. Genes are composed of a series of introns and [[exons]](https://en.wikipedia.org/wiki/Exon), introns are nucleotide sequences which do not encode a protein while, exons are nucleotide sequences that directly encode a protein. Introns and exons are present in both the underlying DNA sequence and the pre-mRNA molecule, therefore, in order to produce a mature mRNA molecule encoding a protein, splicing must occur. During splicing, the intervening introns are removed from the pre-mRNA molecule by a multi-protein complex known as a [[spliceosome]](https://en.wikipedia.org/wiki/Spliceosome) (composed of over 150 proteins and RNA). This mature mRNA molecule is then exported into the cytoplasm through nuclear pores in the envelope of the nucleus. **Translation** During translation, ribosomes synthesize polypeptide chains from mRNA template molecules. In eukaryotes, translation occurs in the cytoplasm of the cell, where the ribosomes are located either free floating or attached to the [[endoplasmic reticulum]](https://en.wikipedia.org/wiki/Endoplasmic_reticulum). In prokaryotes, which lack a nucleus, the processes of both transcription and translation occur in the cytoplasm. [[Ribosomes]](https://en.wikipedia.org/wiki/Ribosome) are complex [[molecular machines]](https://en.wikipedia.org/wiki/Molecular_machine), made of a mixture of protein and [[ribosomal RNA]](https://en.wikipedia.org/wiki/Ribosomal_RNA), arranged into two subunits (a large and a small subunit), which surround the mRNA molecule. The ribosome reads the mRNA molecule in a 5\'-3\' direction and uses it as a template to determine the order of amino acids in the polypeptide chain. In order to translate the mRNA molecule, the ribosome uses small molecules, known as [[transfer RNAs]](https://en.wikipedia.org/wiki/Transfer_RNA) (tRNA), to deliver the correct amino acids to the ribosome. Each tRNA is composed of 70-80 nucleotides and adopts a characteristic cloverleaf structure due to the formation of hydrogen bonds between the nucleotides within the molecule. There are around 60 different types of tRNAs, each tRNA binds to a specific sequence of three nucleotides (known as a [[codon]](https://en.wikipedia.org/wiki/Codon)) within the mRNA molecule and delivers a specific amino acid. The ribosome initially attaches to the mRNA at the [[start codon]](https://en.wikipedia.org/wiki/Start_codon) (AUG) and begins to translate the molecule. The mRNA nucleotide sequence is read in [[triplets]](https://en.wikipedia.org/wiki/Genetic_code) - three adjacent nucleotides in the mRNA molecule correspond to a single codon. Each tRNA has an exposed sequence of three nucleotides, known as the anticodon, which are complementary in sequence to a specific codon that may be present in mRNA. For example, the first codon encountered is the start codon composed of the nucleotides AUG. The correct tRNA with the anticodon (complementary 3 nucleotide sequence UAC) binds to the mRNA using the ribosome. This tRNA delivers the correct amino acid corresponding to the mRNA codon', in the case of the start codon, this is the amino acid methionine. The next codon (adjacent to the start codon) is then bound by the correct tRNA with complementary anticodon, delivering the next amino acid to ribosome. The ribosome then uses its [[peptidyl transferase]](https://en.wikipedia.org/wiki/Peptidyl_transferase) enzymatic activity to catalyze the formation of the covalent peptide bond between the two adjacent amino acids. The ribosome then moves along the mRNA molecule to the third codon. The ribosome then releases the first tRNA molecule, as only two tRNA molecules can be brought together by a single ribosome at one time. The next complementary tRNA with the correct anticodon complementary to the third codon is selected, delivering the next amino acid to the ribosome which is covalently joined to the growing polypeptide chain. This process continues with the ribosome moving along the mRNA molecule adding up to 15 amino acids per second to the polypeptide chain. Behind the first ribosome, up to 50 additional ribosomes can bind to the mRNA molecule forming a [[polysome]](https://en.wikipedia.org/wiki/Polysome), this enables simultaneous synthesis of multiple identical polypeptide chains. Termination of the growing polypeptide chain occurs when the ribosome encounters a stop codon (UAA, UAG, or UGA) in the mRNA molecule. When this occurs, no tRNA can recognise it and a [[release factor]](https://en.wikipedia.org/wiki/Release_factor) induces the release of the complete polypeptide chain from the ribosome. Dr. [[Har Gobind Khorana]](https://en.wikipedia.org/wiki/Har_Gobind_Khorana), a scientist originating from India, decoded the RNA sequences for about 20 amino acids. He was awarded the [[Nobel Prize]](https://en.wikipedia.org/wiki/Nobel_prize) in 1968, along with two other scientists, for his work. Protein folding three individual polypeptide chains at different levels of folding and a cluster of chains Figure Shows the process of a polypeptide chain folding from its initial primary structure through to the quaternary structure. Once synthesis of the polypeptide chain is complete, the polypeptide chain folds to adopt a specific structure which enables the protein to carry out its functions. Post-translational modifications When protein folding into the mature, functional 3D state is complete, it is not necessarily the end of the protein maturation pathway. A folded protein can still undergo further processing through post-translational modifications. There are over 200 known types of post-translational modification, these modifications can alter protein activity, the ability of the protein to interact with other proteins and where the protein is found within the cell e.g. in the cell nucleus or cytoplasm. Through post-translational modifications, the diversity of proteins encoded by the genome is expanded by 2 to 3 [[orders of magnitude]](https://en.wikipedia.org/wiki/Order_of_magnitude). There are four key classes of post-translational modification: 1. Cleavage 2. Addition of chemical groups 3. Addition of complex molecules 4. Formation of intramolecular bonds **Cleavage**  Figure Shows a post-translational modification of the protein by protease cleavage, illustrating that pre-existing bonds are retained even if when the polypeptide chain is cleaved. [[Cleavage]](https://en.wikipedia.org/wiki/Proteolysis) of proteins is an irreversible post-translational modification carried out by enzymes known as [[proteases]](https://en.wikipedia.org/wiki/Proteases). These proteases are often highly specific and cause [[hydrolysis]](https://en.wikipedia.org/wiki/Hydrolysis) of a limited number of peptide bonds within the target protein. The resulting shortened protein has an altered polypeptide chain with different amino acids at the start and end of the chain. This post-translational modification often alters the proteins function, the protein can be inactivated or activated by the cleavage and can display new biological activities. **Addition of chemical groups** Three polypeptide chains with one amino acid side chain showing, two have a lysine and one has a serine. Three arrows indicating different post-translational modifications with the new chemical group added to each side chain. The first is methylation then acetylation followed by phosphorylation. Following translation, small chemical groups can be added onto amino acids within the mature protein structure. Examples of processes which add chemical groups to the target protein include methylation, [[acetylation]](https://en.wikipedia.org/wiki/Protein_acetylation) and [[phosphorylation]](https://en.wikipedia.org/wiki/Protein_phosphorylation). **Addition of complex molecules**  Post-translational modifications can incorporate more complex, large molecules into the folded protein structure. One common example of this is [[glycosylation]](https://en.wikipedia.org/wiki/Glycosylation), the addition of a polysaccharide molecule, which is widely considered to be most common post-translational modification.[^[\[15\]]^](https://en.wikipedia.org/wiki/Protein_biosynthesis#cite_note-Schubert_2015-15) In glycosylation, a [[polysaccharide]](https://en.wikipedia.org/wiki/Polysaccharide) molecule (known as a [[glycan]](https://en.wikipedia.org/wiki/Glycan)) is covalently added to the target protein by [[glycosyltransferases]](https://en.wikipedia.org/wiki/Glycosyltransferases) enzymes and modified by [[glycosidases]](https://en.wikipedia.org/wiki/Glycoside_hydrolases) in the [[endoplasmic reticulum]](https://en.wikipedia.org/wiki/Endoplasmic_reticulum) and [[Golgi apparatus]](https://en.wikipedia.org/wiki/Golgi_apparatus). Glycosylation can have a critical role in determining the final, folded 3D structure of the target protein. In some cases glycosylation is necessary for correct folding. **Formation of covalent bonds** Formation of a disulfide bond between two cysteine amino acids within a single polypeptide chain and formation of a disulphide bond between two cysteine amino acids on different polypeptide chains, thereby joining the two chains. Shows the formation of disulphide covalent bonds as a post-translational modification. Disulphide bonds can either form within a single polypeptide chain (left) or between polypeptide chains in a multi-subunit protein complex (right). Many proteins produced within the cell are secreted outside the cell to function as [[extracellular]](https://en.wikipedia.org/wiki/Extracellular) proteins. Extracellular proteins are exposed to a wide variety of conditions. In order to stabilize the 3D protein structure, covalent bonds are formed either within the protein or between the different polypeptide chains in the quaternary structure. The most prevalent type is a [[disulfide bond]](https://en.wikipedia.org/wiki/Disulfide) (also known as a disulfide bridge). A disulfide bond is formed between two [[cysteine]](https://en.wikipedia.org/wiki/Cysteine) amino acids using their side chain chemical groups containing a Sulphur atom, these chemical groups are known as [[thiol]](https://en.wikipedia.org/wiki/Thiol) functional groups. Disulfide bonds act to stabilize the [[pre-existing structure]](https://en.wikipedia.org/wiki/Protein_structure) of the protein.