Chapter 7: The Blueprint of Life, from DNA to Protein Lecture Outline PDF
Document Details
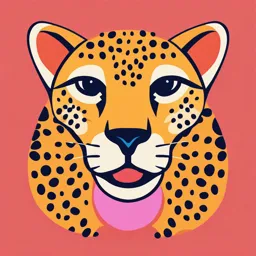
Uploaded by InexpensiveSilver
Denise Anderson, Sarah Salm, Mira Beins
Tags
Related
- Molecular Biology and Cytogenetics: Translation from mRNA to Protein (PDF)
- DNA Replication and Protein Synthesis PDF
- Molecular Biology and Genetics - Explorations: An Open Invitation to Biological Anthropology (2nd Edition) PDF
- Biology Notes: RNA and DNA PDF
- Biology 30 Review - Molecular Biology & Protein Synthesis
- DNA and Protein Synthesis PDF
Summary
This document is a lecture outline which explores the blueprint of life, from DNA to protein. It details the process and components of DNA replication and protein synthesis, and covers foundational concepts in molecular biology. The structure of DNA, RNA types, and other biological processes are outlined in detail.
Full Transcript
Because learning changes everything. ® Chapter 7 The Blueprint of Life, from DNA to Protein Lecture Outline Nester's Microbiology A Human Perspective, Tenth Edition Denise Anderson, Sarah Salm, Mira Beins © 2022 McGraw Hill, LLC. All rights reserved. Authorized only for instructor use in the clas...
Because learning changes everything. ® Chapter 7 The Blueprint of Life, from DNA to Protein Lecture Outline Nester's Microbiology A Human Perspective, Tenth Edition Denise Anderson, Sarah Salm, Mira Beins © 2022 McGraw Hill, LLC. All rights reserved. Authorized only for instructor use in the classroom. No reproduction or further distribution permitted without the prior written consent of McGraw Hill, LLC. A Glimpse of History In 1866, Gregor Mendel determined traits are inherited as physical units, now called genes In 1941, George Beadle and Edward Tatum reported that genes direct production of enzymes • Treated mold (Neurospora species) with X rays • Established metabolic defect inherited as a single gene • Concluded that single gene determines production of one enzyme • Awarded Nobel Prize in 1958, although assumption has been modified since © McGraw Hill, LLC 2 DNA: Blueprint of Life Incredible diversity of life dictated by information within DNA • Composed nucleotides, each containing a nucleobase • Adenine (A) • Thymine (T) • Cytosine (C) • Guanine (G) • 3 nucleotides encode a specific amino acid • Amino acids make up protein • Sequence of amino acids determines structure and function of protein • Structural proteins • Enzymes © McGraw Hill, LLC 3 Overview 1 Genome: complete set of genetic information • Chromosome plus (technically) plasmids • All cells have DNA genome • Viruses may have RNA genome • Functional unit is gene • Encodes gene product, usually a protein • Study of nucleotide sequence of DNA is genomics © McGraw Hill, LLC 4 Overview 2 Cells must accomplish two tasks to multiply • DNA replication • Gene expression: DNA is decoded so cell can synthesize gene products • Transcription: information in DNA is copied into RNA • Translation: RNA used to synthesize encoded protein © McGraw Hill, LLC 5 Overview - Figure 7.1 Information flow from DNA → RNA → protein • Central dogma of molecular biology © McGraw Hill, LLC 6 Characteristics of DNA - Figure 7.2 (1) DNA forms double-stranded helix • Carbon atoms of the pentose sugar are numbered • Nucleotides joined between 5’ PO4 and 3’ OH • Forms sugar-phosphate backbone • Single DNA strand will have a 5′ end and 3′ end Access the text alternative for slide images. © McGraw Hill, LLC 7 Characteristics of DNA - Figure 7.2 (2) Strands are complementary; held together by hydrogen bonds between nucleobases • Base-pairing • Adenine (A) to thymine (T) (two hydrogen bonds) • Cytosine (C) to guanine (G) (three hydrogen bonds) Strands are anti-parallel; oriented in opposite directions © McGraw Hill, LLC 8 Characteristics of RNA RNA (ribonucleic acid) • Ribose instead of deoxyribose • Uracil in place of thymine • Usually shorter single strand • Synthesized from DNA template strand • RNA molecule is transcript • Base-pairing rules apply except uracil pairs with adenine • Transcript quickly separates from DNA © McGraw Hill, LLC 9 Three Types of RNA - Figure 7.3 • Messenger RNA (mRNA) • Ribosomal RNA (rRNA) • Transfer RNA (tRNA) Access the text alternative for slide images. © McGraw Hill, LLC 10 Regulating Gene Expression - Figure 7.4 Cells regulate expression of certain genes • Rapid degradation of mRNA transcripts © McGraw Hill, LLC 11 DNA Replication - Figure 7.5 (1) DNA replication usually bidirectional from origin of replication • Two replication forks meet at terminating site when process complete Access the text alternative for slide images. © McGraw Hill, LLC 12 DNA Replication - Figure 7.5 (2) Replication is semiconservative • DNA contains one original, one newly synthesized strand © McGraw Hill, LLC 13 Initiation of DNA Replication DNA gyrase and helicases bind to origin of replication • Break and unwind DNA helix • Expose single-stranded region that can act as template Primases synthesize short regions of RNA called primers Requires coordinated action of many enzymes and other components Many of the enzymes form “assembly lines” called replisomes © McGraw Hill, LLC 14 The Process of DNA Replication - Figure 7.6 DNA polymerases synthesize in 5’ to 3’ direction • Adds nucleotide to the 3’ end of the new strand • DNA polymerase can only add nucleotides to existing strand, not initiate • Primers are required at origin of replication Access the text alternative for slide images. © McGraw Hill, LLC 15 The Process of DNA Replication - Figure 7.7 Helicases “unzip” DNA strands • Reveals template sequences • Leading strand synthesized continuously • Lagging strand synthesized discontinuously • DNA polymerases can only add nucleotides to 3′ end • Production of Okazaki fragments • Different DNA polymerase replaces primers • DNA ligase forms covalent bond between adjacent nucleotides Access the text alternative for slide images. © McGraw Hill, LLC 16 The Process of DNA Replication Replication produces two complete copies of DNA • Each daughter cell receives one • Replication of E. coli chromosome takes approximately 40 minutes • Optimal generation time is approximately 20 minutes • Cell can initiate another round of replication before previous round is complete • Each daughter cell inherits one complete chromosome already undergoing replication © McGraw Hill, LLC 17 Gene Expression in Bacteria - Figure 7.8 Transcription: RNA polymerase synthesizes single-stranded RNA using DNA as a template • RNA polymerase binds to sequence called a promoter • Synthesizes in 5’ to 3’ direction • Can initiate without primer • Transcription stops at sequence called a terminator Access the text alternative for slide images. © McGraw Hill, LLC 18 Transcription - Figure 7.9 RNA sequence is complementary, antiparallel to DNA template strand • DNA template is minus (−) strand • Complement is plus (+) strand • RNA has same sequence as (+) DNA strand except uracil instead of thymine Access the text alternative for slide images. © McGraw Hill, LLC 19 Prokaryotic mRNA transcripts Monocistronic (one gene) Polycistronic (multiple genes) • Proteins encoded on polycistronic message generally have related functions © McGraw Hill, LLC 20 Initiation of RNA synthesis • Sigma (σ) factor on RNA polymerase recognizes promoter • Various types of sigma (σ) factors recognize different promoters • Synthesis of sigma (σ) factors controls transcription of sets of genes • Eukaryotic cells, archaea use transcription factors to recognize promoters © McGraw Hill, LLC 21 Transcription - Figure 7.10 Access the text alternative for slide images. © McGraw Hill, LLC 22 Transcription - Figure 7.11 Promoter orients direction of transcription • Found upstream of genes • Once RNA polymerase has moved beyond promoter, another RNA polymerase can bind • Allows rapid and repeated transcription of single gene Access the text alternative for slide images. © McGraw Hill, LLC 23 Elongation of the RNA Transcript • RNA polymerase moves along DNA, using the (−) strand as a template to synthesize a single-stranded RNA molecule. • Nucleotides are added only to the 3′ end • When RNA polymerase advances, it denatures a new stretch of DNA and the previous portion renatures. • A new region of the template is exposed so elongation can continue. • Once elongation has proceeded beyond the promoter, another molecule of the RNA polymerase can bind, initiating a new round of transcription. • A single gene can be transcribed repeatedly very quickly. © McGraw Hill, LLC 24 Termination of Transcription • A distinct nucleotide sequences of DNA signals the termination of transcription. • When RNA polymerase encounters a sequence called a terminator, it falls off the DNA template and releases the newly synthesized RNA. © McGraw Hill, LLC 25 Table 7.2 Components of Transcription in Bacteria Component Comment (-) strand of DNA Strand of DNA that serves as the template for RNA synthesis; the resulting RNA molecule is complementary to this strand. (+) strand of DNA Strand of DNA complementary to the one that serves as the template for RNA synthesis; the nucleotide sequence of the RNA molecule is the same as this strand, except it has uracil rather than thymine. Promoter Nucleotide sequence to which RNA polymerase binds to initiate transcription. RNA polymerase Enzyme that synthesizes RNA using one strand of DNA as a template; synthesis always occurs in the 5′ to 3′ direction. Sigma (σ) factor Component of RNA polymerase that recognizes the promoter regions. A cell can have different types of σ factors that recognize different promoters, allowing the cell to transcribe specialized sets of genes as needed. Terminator Nucleotide sequence at which RNA synthesis stops; the RNA polymerase falls off the DNA template and releases the newly synthesized RNA. © McGraw Hill, LLC 26 Translation 1 Process of decoding information in mRNA to synthesize a specific protein • The process requires mRNA, ribosomes (rRNA), tRNAs, and accessory proteins • mRNA is temporary copy of genetic information Cells must decode information in nucleotides of mRNA into amino acids of proteins Genetic code: three nucleotides = a codon = one amino acid • Practically universal; with minor exceptions, it is used by all living things © McGraw Hill, LLC 27 The Role of mRNA A codon is a triplet of any combination of the four nucleotides • 64 different possible codons (43 ). • Three are stop codons, which signal the end of translation. • 61 codons translate to the 20 different amino acids. • More than one codon can code for a specific amino acid. • For example. both ACA and ACG encode the amino acid threonine. • The genetic code is said to be degenerate. © McGraw Hill, LLC 28 Table 7.3 The Genetic Code 1 © McGraw Hill, LLC 29 Table 7.3 The Genetic Code 2 © McGraw Hill, LLC 30 The Role of mRNA - Figure 7.12 A given sequence can have three possible reading frames (ways in which triplets of nucleotides can be grouped) • If translation begins in wrong reading frame, a very different, and likely nonfunctional protein results Access the text alternative for slide images. © McGraw Hill, LLC 31 The Role of mRNA - Figure 7.13 Nucleotide sequence defines coding region • Designates beginning, end of region to be translated Access the text alternative for slide images. © McGraw Hill, LLC 32 The Role of Ribosomes Serves as a translation “machine” • Aligns and forms peptide bond between amino acid • Locate punctuation sequences on mRNA molecule • In prokaryotes, the ribosome will begin to assemble at a sequence in mRNA called the ribosome-binding site • The first AUG after that site is the start codon • Ribosome moves along mRNA in the 5′ to 3′ direction “presenting” each codon in a sequential order for decoding while maintaining the correct reading frame • Translation ends at a stop codon • Prokaryotic ribosome comprised of 30S and 50S subunits • Made up of protein and ribosomal RNA (rRNA) © McGraw Hill, LLC 33 The Role of Transfer RNAs - Figure 7.14 Delivers correct amino acid • Each has specific anticodon sequence; base-pairs with codon • Each carries appropriate amino acid specified by codon • After delivering amino acid, tRNA can be recycled Access the text alternative for slide images. © McGraw Hill, LLC 34 Initiation - Figure 7.15 Part of ribosome binds to mRNA sequence called the ribosome-binding site First AUG after that site serves as start codon, where complete ribosome assembles Initiating tRNA brings altered form of methionine • Occupies P-site Access the text alternative for slide images. © McGraw Hill, LLC 35 Elongation - Figure 7.16 (1) Ribosome has two sites to which tRNAs can bind • tRNA that recognizes next codon occupies empty A-site; brings correct amino acid A-site and P-site now occupied by correct tRNAs • rRNA creates peptide bond between their amino acids • Amino acid from tRNA in P-site added to amino acid carried by tRNA in A-site Access the text alternative for slide images. © McGraw Hill, LLC 36 Elongation - Figure 7.16 (2) Ribosome advances along mRNA in 5′ to 3′ direction • Initiating tRNA exits through E-site • Remaining tRNA carrying both amino acids occupies P-site • A-site temporarily empty • A tRNA that recognizes codon in A-site quickly attaches © McGraw Hill, LLC 37 Elongation– Figure 7.16 (3) Peptide bond formed between amino acids Ribosome advances one codon on mRNA • tRNA exits E-site • tRNA with growing protein occupies P site • new tRNA occupies A-site The process repeats © McGraw Hill, LLC 38 Termination - Figure 7.17 (1) Elongation continues until ribosome reaches stop codon • Not recognized by tRNA • Enzymes break covalent bond joining to polypeptide to tRNA Access the text alternative for slide images. © McGraw Hill, LLC 39 Termination - Figure 7.17 (2) Ribosome falls off mRNA • Disassociates into component subunits (30S and 50S) • Subunits can be reused to initiate translation at other sites © McGraw Hill, LLC 40 Translation in Prokaryotes- Figure 7.18 Translation in prokaryotes begins before transcription is complete • Once ribosome clears initiating sequences, another ribosome can bind: forms polyribosome, or polysome Access the text alternative for slide images. © McGraw Hill, LLC 41 Differences Between Eukaryotic and Prokaryotic Gene Expression 1 Eukaryotic mRNA synthesized in precursor form: pre-mRNA • Must be processed during and after transcription • Capping adds methylated guanine derivative to 5′ end • Binds specific proteins: stabilize, enhance translation • 3′ end modified via polyadenylation • Addition of approximately 200 adenine derivatives to new 3′ end • Poly A tail stabilizes transcript, enhances translation © McGraw Hill, LLC 42 Differences Between Eukaryotic and Prokaryotic Gene Expression - Figure 7.19 Splicing removes segments of eukaryotic transcript • Non-coding intervening sequences called introns are removed • Expressed regions called exons remain © McGraw Hill, LLC 43 Differences Between Eukaryotic and Prokaryotic Gene Expression 2 Eukaryotic mRNA produced within membrane-bound nucleus and must be transported to cytoplasm • Translation cannot begin during transcription • mRNA typically monocistronic Translation begins at first AUG Ribosomes composed of 40s and 60s subunits • Differences from prokaryotic ribosomes important in targeting antibiotics © McGraw Hill, LLC 44 Sensing and Responding to Environmental Fluctuations Microorganisms constantly face changing environment and must adapt quickly to survive • E. coli in mammalian intestinal tract • Alternating periods of feast and famine • Host eats, bacteria take up amino acids, vitamins, nutrients • Bacteria shut down biosynthetic pathways, channel energy into production of cellular components (for example, DNA, protein) • Between meals, nutrients depleted • Bacterial biosynthetic pathways activated • Energy consumed, growth slowed • Later, some E. coli eliminated in feces • Face entirely different set of conditions © McGraw Hill, LLC 45 Signal Transduction • Transmission of information from outside cell to inside • Allows cells to monitor and react to environmental conditions © McGraw Hill, LLC 46 Chorum Sensing - Figure 7-20 Allows some organisms to “sense” density of their own population • Cells activate genes useful only when expressed by a critical mass producing a signaling molecule • For example , biofilm formation, pathogens′ infective process • Some can detect or interfere with signaling molecules of • other species © McGraw Hill, LLC 47 Two Component Regulatory System - Figure 7.21 Consists of two different proteins: • Membrane-spanning sensor • Modifies internal region in response to specific environmental variations • Phosphorylates amino acid • Response regulator • Phosphate group transferred from sensor • Regulator turns genes on or off • Example: pathogen sensing magnesium levels to recognize presence in host cell Access the text alternative for slide images. © McGraw Hill, LLC 48 Natural Selection Natural selection can play role in gene expression • Expression of some genes changes randomly in cells • Enhances survival of at least part of population • Antigenic variation is alteration of characteristics of surface proteins • Allows pathogens to stay one step ahead of host defenses • For example, Neisseria gonorrhoeae has many genes for pilin, protein subunit of pili • • • • Only expresses gene in expression locus Randomly moves genes in and out of expression locus Immune system responds initially to dominant pilin type Bacteria that have “switched” type survive • Phase variation involves switching genes on and off • Allows E. coli to attach via pili, detach by turning off © McGraw Hill, LLC 49 Bacterial Gene Regulation Genes can be routinely expressed or regulated • A set of regulated genes transcribed as single mRNA along with its control sequences is termed operon • For example, lac operon for lactose metabolism • Separate operons controlled by single regulatory mechanism constitute regulon • Global control is simultaneous regulation of numerous genes © McGraw Hill, LLC 50 Bacterial Gene Regulation - Figure 7.22 Enzymes can be grouped by type of regulation • Constitutive enzymes synthesized constantly • Typically indispensable roles in central metabolism (for example, enzymes of glycolysis) • Inducible enzymes synthesized only when needed (for example, β-galactosidase turned on only when lactose present) • Avoids waste of resources • Repressible enzymes produced routinely, but are turned off when not needed Access the text alternative for slide images. © McGraw Hill, LLC 51 Mechanisms to Control Gene Regulation Mechanisms to Control Transcription • Must be readily reversible, allow cells to control relative number of transcripts produced • Two most common regulatory mechanisms are alternative sigma factors and DNA-binding proteins © McGraw Hill, LLC 52 Alternative Sigma Factors Standard sigma factor is loose component of RNA polymerase; recognizes promoters for genes expressed during routine growth conditions Alternative sigma factors recognize different sets of promoters to control expression of specific groups of genes • For example, sporulation in Bacillus subtilis controlled by multiple different alternative sigma factors © McGraw Hill, LLC 53 DNA Binding Proteins Can act as repressors • Repressor blocks transcription (negative regulation) • Binds to operator, stops RNA polymerase • Repressors are allosteric: have binding site that alters ability to bind to DNA Repressors can play a role in two types of transcriptional regulatory systems: • Induction: repressor binds to operator, blocks transcription • Inducer binds to repressor, repressor unable to bind • Repression: repressor cannot bind to operator • Corepressor attaches to repressor, complex now binds to operator and blocks transcription © McGraw Hill, LLC 54 Transcriptional Regulation by Repressors - Figure 7.23 Access the text alternative for slide images. © McGraw Hill, LLC 55 DNA Binding Proteins - Figure 7.24 Can act as activators • Activator facilitates transcription (positive regulation) • Ineffective promoter preceded by activator-binding site • Binding of activator enhances ability of RNA polymerase to initiate transcription at promoter • Inducer binding to activator allows binding to DNA • Inducer may also interfere with repressor © McGraw Hill, LLC 56 The lac Operon as a Model Encodes proteins involved with transport and degradation of lactose • Turned on when glucose is not available, but lactose is • Turned off when glucose is available • Cell uses most efficiently metabolized carbon source © McGraw Hill, LLC 57 Lactose and the lac Operon - Figure 7.25 When lactose is not available • Repressor prevents transcription; binds operator When lactose is present • Some converted to inducer allolactose; binds repressor • Repressor releases operator • RNA polymerase transcribes operon • Only occurs when glucose is not available Access the text alternative for slide images. © McGraw Hill, LLC 58 Glucose and the lac Operon - Figure 7.26 When both glucose and lactose are present • Carbon catabolite repression (CCR) prevents expression of genes that metabolize lactose in presence of glucose • Prioritize carbon/energy sources; yields diauxic growth Access the text alternative for slide images. © McGraw Hill, LLC 59 Glucose and the lac Operon Glucose transport system senses glucose • Catabolite activator protein (CAP) required for transcription of lac operon • Functional only when bound by inducer called cAMP • cAMP made only when glucose level is low • Enzymes that make cAMP activated by idle form of glucose transporter • Inducer exclusion: lactose transporter blocked during • glucose transport by active glucose transporter © McGraw Hill, LLC 60 Glucose and the lac Operon - Figure 7.27 Access the text alternative for slide images. © McGraw Hill, LLC 61 Eukaryotic Gene Regulation Eukaryotic regulation more complicated than in prokaryotes Variety of control methods: • Modification of chromosome structure • Regulation of initiation of transcription • Altering pre-mRNA processing and modification • RNA interference (RNAi) © McGraw Hill, LLC 62 RNA Interference (RNAi) - Figure 7.28 Short RNA strand joins multi-protein unit • RNA-induced silencing complex (RISC) RNA strand serves as probe for binding to mRNA Tags mRNA for destruction • RISC is catalytic • Rapidly silences transcripts microRNA (miRNA) and short interfering RNA (siRNA) • About 2 dozen nucleotides; • produced differently, but functionally equivalent Access the text alternative for slide images. © McGraw Hill, LLC 63 Genomics 1 Haemophilus influenzae first genome published (1995) • Microbial genome sequencing now common • Data interpretation complex due to: • Promoter orientation • Which DNA strand is template • Reading frame • New field of bioinformatics creates the computer technology needed to store, retrieve, and analyze sequence data © McGraw Hill, LLC 64 Genomics 2 Analyzing a Prokaryotic DNA Sequence • (+) strand used to represent sequence of RNA transcript • ATG in (+) DNA indicates possible start codon • Computers search for open reading frames (ORFs) • Stretches of nucleotides generally longer than 300 bp • Begin with start codon, end with stop codon • ORF potentially encodes protein • Other characteristics (for example, upstream sequence serving as ribosome-binding site) also suggest ORF encodes protein • Can be compared with published sequences • Presumed function can be assigned © McGraw Hill, LLC 65 Metagenomics Analysis of total microbial genomes in environment Can study all microbes in community • Not limited to those that grow in culture • Can track changes in composition of microbiota of individual over time (healthy, diseased) • Compare microbiota at different body sites • Also between different individuals • Study microbial life in oceans, soils • New understanding of extent of biodiversity • May lead to discoveries of useful compounds (for example, antibiotics) Tremendous amount of data presents challenges © McGraw Hill, LLC 66 Because learning changes everything. www.mheducation.com © 2022 McGraw Hill, LLC. All rights reserved. Authorized only for instructor use in the classroom. No reproduction or further distribution permitted without the prior written consent of McGraw Hill, LLC. ®