Chapter 6: Multiple Reactions PDF Fall 2024
Document Details
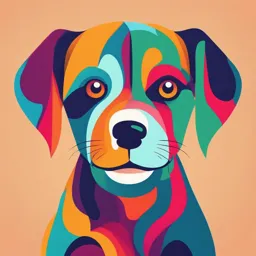
Uploaded by AwedIvy
Ovation Kuwait University
2024
Tags
Summary
This document is a lecture on chemical engineering related to multiple reactions. It covers topics such as parallel, series, independent and complex reactions. The document includes examples and calculations for understanding the concept.
Full Transcript
Chapter 6: Multiple Reactions Chapter 8 in Fifth Edition ChE 324: Kinetics and Reactor Design (A) Fall 2024 1 Reactor Design for Multiple Reactions Usually, more than one reaction occurs within a chemical reactor Min...
Chapter 6: Multiple Reactions Chapter 8 in Fifth Edition ChE 324: Kinetics and Reactor Design (A) Fall 2024 1 Reactor Design for Multiple Reactions Usually, more than one reaction occurs within a chemical reactor Minimization of undesired side reactions that occur with the desired reaction contributes to the economic success of a chemical plant Goal: determine the reactor conditions and configuration that maximizes product formation Reactor design for multiple reactions 1. Parallel reactions 2. Series reactions 3. Independent reactions 4. More complex reactions Use of selectivity factor to select the proper reactor that minimizes unwanted side reactions 2 Introduction 1. Parallel Reactions (also called competing reactions): An example of a parallel reaction Parallel reactions are reactions in which the reactant is the oxidation of ethylene to ethylene oxide. In this reaction, is consumed by at least two different reactions we want to avoid the forming at least two different products. combustion of ethylene to water In this example, reactant A is participating in two and CO2. reactions simultaneously. One resulting in product B and the other in product C. Each reaction will have a different rate law and a different rate constant. Desired product 3 Introduction 2. Series Reactions (also called consecutive reactions): In series reactions, the multiple reactions occur in series, that is, the reactant forms an intermediate product, which then reacts further to form another product. An example of series reactions is the reaction of ethylene oxide with ammonia: Desired product 4 Introduction 3. Complex Reactions: Complex reaction situations involve a combination of both series and parallel reactions: k 1 C+D k 2 →E A + B → A + C 4. Independent Reactions: Independent reactions are reactions that occur simultaneously, but neither the products nor reactants interact with one another. Crude oil cracking 5 Desired and Undesired Reactions Since we have multiple reactions occurring simultaneously, we are typically only interested in one of the reactions or interested in only one of the products. In a typical reactor, we are supplying the reactant so that only the desired product is formed. If a significant quantity of the undesired product forms, that is a loss in terms of the reactant used, as well as that would increase the cost of separation. We call the product that we are interested in the desired product, while the products that we are not interested in the undesired products. Desired and Undesired Desired and Undesired reactions in a series reactions in a parallel reaction sequence reaction sequence 6 Selectivity To quantify how much a desired and undesired product forms in a given reaction, we will use two quantities: the yield and selectivity. Selectivity tells us how much a desired product has formed relative to undesired products. The Instantaneous Selectivity 𝑺𝑺𝑫𝑫/𝑼𝑼 is defined as the ratio of the rate of formation of the desired product to the rate of formation of the undesired product: Instantaneous means that the selectivity is measured at a specific instant, that is imagine yourself inside the reactor and measure the selectivity as the reaction is progressing. 7 Selectivity Another value for the selectivity is overall selectivity, which is defined as the molar flowrate (number of moles for a batch reactor) of the desired product to the molar flowrates (number of moles for a batch reactor) of the undesired product. The overall selectivity tells us the selectivity after the reaction has occurred. 8 Yield The reaction yield tells us how much a desired product formed relative to a key reactant. Like the selectivity, we have two definitions for the yield: instantaneous yield and overall yield. The instantaneous yield is defined as the ratio of the rate of formation of the desired product to the rate of consumption of a key reactant. It is measured at a certain instant during the course of the reaction. The overall yield is the ratio of the molar flowrates of the desired product (number of moles for a batch reactor) to the molar flowrates of a key reactant that were consumed in the reaction. It is measured after the reaction has occurred. 9 Parallel Reactions Consider the two competing parallel The rate of disappearance of A reactions: is just the sum of the rates of disappearance of A from both reactions: The rates of the formation 𝐷𝐷 and 𝑈𝑈 are: 10 Parallel Reactions Based on the rates in the previous slides, the instantaneous selectivity is then: 𝛼𝛼 𝑟𝑟𝐷𝐷 𝑘𝑘𝐷𝐷 𝐶𝐶𝐴𝐴 1 𝑘𝑘𝐷𝐷 𝛼𝛼1 −𝛼𝛼2 𝑆𝑆𝐷𝐷/𝑈𝑈 = = 𝛼𝛼2 = 𝐶𝐶𝐴𝐴 𝑟𝑟𝑈𝑈 𝑘𝑘𝑈𝑈 𝐶𝐶𝐴𝐴 𝑘𝑘𝑈𝑈 In any reaction, we want the rate of formation of the desired product 𝑟𝑟𝐷𝐷 to be larger than the rate of formation of the undesired product 𝑟𝑟𝑈𝑈 , that is, we want 𝑺𝑺𝑫𝑫/𝑼𝑼 to be as large as possible. 11 Maximizing The Selectivity Since we want the selectivity to be as high as possible, we will discuss some strategies that can be implemented to do that. The strategy that we use to improve the selectivity (improve the formation of the desired product) will depend on the reaction conditions. Recognizing that 𝑟𝑟𝐷𝐷 𝑘𝑘𝐷𝐷 𝛼𝛼1−𝛼𝛼2 𝑆𝑆𝐷𝐷/𝑈𝑈 = = 𝐶𝐶𝐴𝐴 𝑟𝑟𝑈𝑈 𝑘𝑘𝑈𝑈 We want to increase 𝑆𝑆𝐷𝐷/𝑈𝑈. 12 Maximizing The Selectivity: Effect of Concentration Case 1: When 𝛼𝛼1 > 𝛼𝛼2 : In this case, the reaction order of the rate of formation of the desired product is greater than the reaction order of the rate of formation of the undesired product: 𝛼𝛼1 − 𝛼𝛼2 = 𝑎𝑎 > 0: The selectivity will then be: 𝑘𝑘𝐷𝐷 𝑎𝑎 𝑆𝑆𝐷𝐷/𝑈𝑈 = 𝐶𝐶𝐴𝐴 𝑘𝑘𝑈𝑈 That is, increasing the concentration 𝐶𝐶𝐴𝐴 will increase the selectivity, so we should run this reaction while keeping the concentration of A as high as possible If the reaction is in the gas-phase, use high pressure and run reaction without any inert species. If the reaction is in the liquid phase, we should not use dilutants. Further, this reaction should be conducted in a batch or PFR so that the concentration of A is high at the beginning. 13 Maximizing The Selectivity: Effect of Concentration Case 2: When 𝛼𝛼1 < 𝛼𝛼2 : In this case, the reaction order of the rate of formation of the desired product is less than the reaction order of the rate of formation of the undesired product: 𝛼𝛼2 − 𝛼𝛼1 = 𝑏𝑏: The selectivity will then be: 𝑘𝑘𝐷𝐷 −𝑏𝑏 𝑘𝑘𝐷𝐷 1 𝑆𝑆𝐷𝐷/𝑈𝑈 = 𝐶𝐶𝐴𝐴 = 𝑏𝑏 𝑘𝑘𝑈𝑈 𝑘𝑘𝑈𝑈 𝐶𝐶𝐴𝐴 In this case, decreasing the concentration of A and making it as low as possible will increase the formation of the desired product and improve the selectivity. This can be achieved by running the reaction with inerts and diluting the feed with them. Further, a CSTR should be used as the reactant concentrations are kept to minimum. 14 Maximizing The Selectivity: Effect of Temperature Changing the reaction’s temperature will also have an effect on selectivity. However, whether we increase or decrease the temperature to increase the selectivity will depend on the activation energies of the desired and undesired reactions. This is due to the fact that the activation energy will tell us how the rate reacts with increasing temperature (specifically, it tells us how the specific reaction rate changes with temperature). If the activation energy is large, that means that increasing the temperature will increase 𝑘𝑘 much more rapidly than when the activation energy is small. 𝐸𝐸 Remember that 𝑘𝑘 = 𝐴𝐴 exp − 𝑅𝑅𝑅𝑅 15 Maximizing The Selectivity: Effect of Temperature Case 3: 𝐸𝐸𝐷𝐷 > 𝐸𝐸𝑈𝑈 : That is, the activation energy of the desired reaction is larger than the activation energy of the undesired reaction. Increasing the temperature will lead to an increase in both (desired and undesired) reaction rates, but the rate of the desired reaction 𝑟𝑟𝐷𝐷 will increase more than the rate of the undesired 𝑟𝑟 reaction 𝑟𝑟𝑈𝑈 As such, 𝑆𝑆𝐷𝐷/𝑈𝑈 = 𝐷𝐷 will increase with temperature reaction 𝑟𝑟𝑈𝑈 should be operated at highest possible temperature. Assuming that the reaction orders for the desired and undesired reactions are equal: 𝐸𝐸 𝐸𝐸 Since 𝑘𝑘𝐷𝐷 = 𝐴𝐴𝐷𝐷 exp − 𝐷𝐷 , & 𝑘𝑘𝑈𝑈 = 𝐴𝐴𝑈𝑈 exp − 𝑈𝑈 𝑅𝑅𝑅𝑅 𝑅𝑅𝑅𝑅 𝑟𝑟𝐷𝐷 𝑘𝑘𝐷𝐷 𝐴𝐴𝐷𝐷 𝐸𝐸𝐷𝐷 − 𝐸𝐸𝑈𝑈 𝑆𝑆𝐷𝐷/𝑈𝑈 = = = exp − 𝑟𝑟𝑈𝑈 𝑘𝑘𝑈𝑈 𝐴𝐴𝑈𝑈 𝑅𝑅𝑅𝑅 16 Maximizing The Selectivity: Effect of Temperature Case 4: 𝐸𝐸𝑈𝑈 > 𝐸𝐸𝐷𝐷 : That is, the activation energy of the desired reaction is smaller than the activation energy of the undesired reaction. Increasing the temperature will lead to an increase in both (desired and undesired) reaction rates, but the rate of the undesired reaction 𝑟𝑟𝑈𝑈 will increase more than the rate of the undesired reaction 𝑟𝑟𝑈𝑈 As such, 𝑆𝑆𝐷𝐷/𝑈𝑈 = 𝑟𝑟𝐷𝐷 will decrease with temperature reaction should be operated at lowest possible 𝑟𝑟𝑈𝑈 temperature, but high enough to have an acceptable rate of reaction. Assuming that the reaction orders for the desired and undesired reactions are equal: 𝐸𝐸𝐷𝐷 𝐸𝐸 Since 𝑘𝑘𝐷𝐷 = 𝐴𝐴𝐷𝐷 exp − , & 𝑘𝑘𝑈𝑈 = 𝐴𝐴𝑈𝑈 exp − 𝑈𝑈 𝑅𝑅𝑅𝑅 𝑅𝑅𝑅𝑅 𝑟𝑟𝐷𝐷 𝑘𝑘𝐷𝐷 𝐴𝐴𝐷𝐷 𝐸𝐸𝐷𝐷 − 𝐸𝐸𝑈𝑈 𝑆𝑆𝐷𝐷/𝑈𝑈 = = = exp − 𝑟𝑟𝑈𝑈 𝑘𝑘𝑈𝑈 𝐴𝐴𝑈𝑈 𝑅𝑅𝑅𝑅 17 Effect of Temperature on Selectivity 18 Maximize SD/U for Parallel Reactions: Temperature k D ( D − ED −EU ) A AD SD U = e RT CAα1−α 2 AU U kU What reactor temperature maximizes the selectivity? ED = 20 kcal/mol, EU = 10 kcal/mol, T = 25 ◦C (298K) or 100 ◦C (373K) T = 100 ◦C a) ED > EU (373K): 𝑐𝑐𝑎𝑎𝑐𝑐 𝑐𝑐𝑎𝑎𝑐𝑐 𝐴𝐴𝐷𝐷 − 20,000 − 10,000 𝐴𝐴𝐷𝐷 𝑚𝑚𝑚𝑚𝑐𝑐 𝑚𝑚𝑚𝑚𝑐𝑐 𝑆𝑆𝐷𝐷/𝑈𝑈 = exp 𝐶𝐶𝐴𝐴 𝛼𝛼1−𝛼𝛼2 → 𝑆𝑆𝐷𝐷/𝑈𝑈 = 1.4 × 10−6 𝐶𝐶𝐴𝐴 𝛼𝛼1−𝛼𝛼2 𝐴𝐴𝑈𝑈 𝑐𝑐𝑎𝑎𝑐𝑐 𝐴𝐴𝑈𝑈 1.987 373𝐾𝐾 𝑚𝑚𝑚𝑚𝑐𝑐 ⋅ 𝐾𝐾 T = 25 ◦C kD/U (298K): 𝑐𝑐𝑎𝑎𝑐𝑐 𝑐𝑐𝑎𝑎𝑐𝑐 𝐴𝐴𝐷𝐷 − 20,000 − 10,000 𝐴𝐴𝐷𝐷 𝑚𝑚𝑚𝑚𝑐𝑐 𝑚𝑚𝑚𝑚𝑐𝑐 𝛼𝛼1 −𝛼𝛼2 𝑆𝑆𝐷𝐷/𝑈𝑈 = exp 𝐶𝐶𝐴𝐴 → 𝑆𝑆𝐷𝐷/𝑈𝑈 = 4.6 × 10−8 𝐶𝐶𝐴𝐴 𝛼𝛼1−𝛼𝛼2 𝐴𝐴𝑈𝑈 𝑐𝑐𝑎𝑎𝑐𝑐 𝐴𝐴𝑈𝑈 1.987 298𝐾𝐾 𝑚𝑚𝑚𝑚𝑐𝑐 ⋅ 𝐾𝐾 SD/U is greater at 373K, higher temperature to favors desired product formation 19 Maximizing SD/U for Parallel Reactions: Concentration k D ( D) − ED −EU A+B kU SD U = AD AU e RT (CAα1−α2 ) CBβ1−β2 U What reactor conditions and configuration maximizes the selectivity? Now evaluate concentration: a) α1 > α 2 → α1 − α 2 > 0 b) α1 < α 2 → α1 − α 2 < 0 CAα1−α 2 CAα1−α 2 → Use large CA → Use small CA c) β1 > β2 → β1 − β 2 > 0 d) β1 < β2 → β1 − β 2 < 0 CB β1− β2 CB β1− β2 → Use large CB → Use small CB How do these concentration requirements affect reactor selection? 20 Reactor Selection and Operating Conditions In this section, we want to know what is the appropriate type of reactor to choose for a given set of conditions such that the selectivity is maximized 𝑆𝑆𝐷𝐷/𝑈𝑈 is maximized. Consider the following two simultaneous (parallel) reactions: The instantaneous selectivity is defined as: 𝛼𝛼 𝛽𝛽 𝑟𝑟𝐷𝐷 𝑘𝑘1 𝐶𝐶𝐴𝐴 1 𝐶𝐶𝐵𝐵 1 𝑘𝑘1 𝛼𝛼1 −𝛼𝛼2 𝛽𝛽1 −𝛽𝛽2 𝑆𝑆𝐷𝐷/𝑈𝑈 = = = 𝐶𝐶 𝐶𝐶𝐵𝐵 𝑟𝑟𝑈𝑈 𝑘𝑘2 𝐶𝐶 𝛼𝛼2 𝐶𝐶 𝛽𝛽2 𝑘𝑘2 𝐴𝐴 𝐴𝐴 𝐵𝐵 We want to maximize the above quantity What reactor to use? 21 Reactor Selection and Operating Conditions 𝑘𝑘1 𝛼𝛼1 −𝛼𝛼2 𝛽𝛽1 −𝛽𝛽2 max 𝐶𝐶𝐴𝐴 𝐶𝐶𝐵𝐵 →? 𝑘𝑘2 22 Concentration Requirements & Reactor Selection kD D How do concentration requirements play into A+B reactor selection? kU U CA0υ0 CAυ0 CB0υ0 CBυ0 PFR/PBR PFR (or PBR): concentration is high CSTR: concentration is at the inlet & progressively drops always at its lowest value to the outlet concentration (that at outlet) CBυ0 Semi-batch: concentration of Batch: concentration one reactant (A as shown) is is high at t=0 & high at t=0 & progressively CA(t) CB(t) progressively drops drops with increasing time, with increasing time CA whereas concentration of B can be kept low at all times 23 What reactor/reactors scheme and conditions would you use to maximize the selectivity parameters for the following parallel reaction? kD −2000 E/R −300 A+C D desired kU1 rD = 800e T CA 0.5 CC rU = 10e T CA CC 1 A+C U1 undesired −E Need to maximize SD/U1 ( ) k T = AeRT ( − ED −EU 1 ) r AD αD −αU βD − βU SD U = D = e T CA 1 CC 1 1 r AU U 1 1 −( 2000 −300 ) −1700 (CA0.5−1) CC1−1 Plug in 800 numbers: SD U1 = e T → SD U1 = 80e T CA −0.5 10 To maximize the production of the desired product, the temperature should be a) As high as possible (without decomposing the reactant or product) b) Neither very high or very low ED > EU, so use higher T c) As low as possible (but not so low the rate = 0) d) Doesn’t matter, T doesn’t affect the selectivity e) Not enough info to answer the question 24 What reactor/reactors scheme and conditions would you use to maximize the selectivity parameters for the following parallel reaction? kD −2000 −300 A+C D desired kU1 rD = 800e T CA 0.5 CC rU1 = 10e T C A CC A+C U1 undesired −E Need to maximize SD/U1 k (T) = AeRT ( − ED −EU 1 ) r AD C αD −αU1 C βD − βU1 SD U = D = e T A C 1 r AU U1 1 −( 2000 −300 ) −1700 (CA0.5−1) CC1−1 Plug in 800 numbers: SD U1 = e T → SD U1 = 80e T CA −0.5 10 To maximize the production of the desired product, CA should be a) As high as possible b) Neither very high or very low c) As low as possible d) Doesn’t matter, CA doesn’t affect the selectivity e) Not enough info to answer the question 25 Series Reactions For multiple reactions that occur in series: where, B is the desired product, and C is the undesired product. To increase the selectivity for reactions occurring in series, the important parameter to control is the space time 𝜏𝜏 for continuous reactors, or time for batch reactors why? This is because in reactions occurring in series, we want to run the reaction as fast as possible to the point we do not allow the formation of the undesired product. For example, if the desired reaction is fast (A → B is fast) and the undesired reaction (B → C is slow), the space-time 𝜏𝜏 is reduced so that the undesired reaction does not occur to a large extent. If the desired reaction is slow, while the undesired reaction is fast, it is almost impossible to form the desired product it will be converted to the undesired product very fast. 26 Series (Consecutive) Reactions k1 k2 A D U Time is the key factor here!!! (desired) (undesired) Spacetime τ for a flow reactor Real time t for a batch reactor To maximize the production of D, use: Batch CSTRs in series or PFR/PBR or n and carefully select the time (batch) or spacetime (flow) 27 Example Problem Example Problem: Develop a relationship between the instantaneous and overall selectivity for a CSTR. 28 Example Problem Parallel Reactions 29 Example Problem Parallel Reactions 30 Example Problem Series Reactions 31 Example Problem Series Reactions 32 Concentrations in Series Reactions k1 k2 -rA = k1CA A B C rB,net = k1CA – k2CB How does CA depend on τ? dFA dC A = −k1C A → υ0 = −k1C A → C A = C A0e −k1τ dV dV How does CB depend on τ? dFB dV = k1C A − k 2CB → υ0 dCB dV = k1 C A0e −k1τ − k 2CB ( ) Substitute V υ0 =τ → dCB dτ ( = k1 CA0 e−k1τ − k 2CB → ) dCB dτ + k 2CB = k1 CA0 e−k1τ ( ) Use integrating factor → ( d CBek 2τ ) =k C e e−k1τ − e−k 2τ (k 2 −k1)τ → CB = k1CA0 1 A0 k 2 − k1 dτ CC = CA0 − CA − CB 33 Reactions in Series: Cj & Yield B CA = CA0 e−k1τ A C e−k1τ − e−k 2τ CB = k1CA0 k 2 − k1 CC = CA0 − CA − CB τopt The reactor V (for a given υ0) and τ that maximizes CB occurs when dCB/dt=0 dCB k1CA0 = dτ k 2 − k1 −( k1e −k1τ + k 2 e −k 2τ )=0 1 k τ opt = ln 1 k1 − k 2 k 2 V = τ so Vopt = υ0τ opt υ0 34 kD D High CA favors desired product High CA favors undesired product A+B α1 > α2 α1 < α2 formation formation (keep CA low) kU U PFR/PBR β 1 > β2 Batch reactor Side streams feed low CA High CB favors CA When CA & CB are low (end time or position), all rxns Semi-batch reactor slowly desired will be slow feed A to large amount of B ←High CB product PFR/PBR formation High P for gas-phase rxn, do not add inert gas (dilutes CA CA CA CSTRs in series reactants) PFR/PBR w/ side CSTR PFR/PBR w/ high recycle β1 < β2 streams feeding low CB CB CA0υ0 CAυ0 High CB favors CB0υ0 CBυ0 Semi-batch reactor, slowly undesired feed B to large amount of A ←High CA Dilute feed with inerts that are product easily separated from product formation CSTRs in series CB CB CB B consumed before leaving Low P if gas phase (keep CB low) CSTRn 35