Pharmaceutical Technology Chapter 6 PDF
Document Details
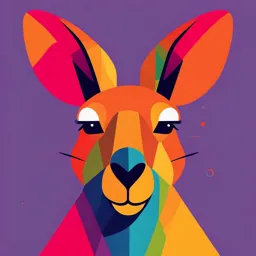
Uploaded by CharismaticHydrogen
Al-Zaytoonah University of Jordan
Dr. Suhair Sunoqrot Dr. Nisrein Jaber
Tags
Summary
This chapter provides an overview of pulmonary drug delivery, covering different aspects such as local and systemic drug activity, lung anatomy, various delivery devices (e.g., inhalers, nebulizers), and formulation considerations. It also touches upon the deposition mechanisms in the airways and the effects of environmental factors such as humidity on particle size.
Full Transcript
PHARMACEUTICAL TECHNOLOGY CHAPTER 6 Pulmonary Drug Delivery Dr. Suhair Sunoqrot Dr. Nisrein Jaber Inhaled drug delivery 1. Local drug activity Treatment and prophylaxis of airway diseases Asthma, COPD, cystic fibrosis Advantages: 1. Fa...
PHARMACEUTICAL TECHNOLOGY CHAPTER 6 Pulmonary Drug Delivery Dr. Suhair Sunoqrot Dr. Nisrein Jaber Inhaled drug delivery 1. Local drug activity Treatment and prophylaxis of airway diseases Asthma, COPD, cystic fibrosis Advantages: 1. Faster onset of action 2. Requires smaller doses compared to oral or parenteral, reducing the systemic side effects and drug costs 3. For drugs with poor oral bioavailability (Na cromoglicate) or those metabolized orally (isoprenaline) Inhaled drug delivery 2. Systemic drug activity Migraine (ergotamine), insulin, vaccines, growth hormone Advantages: 1. Large surface area for absorption 2. Abundance of capillaries 3. Thin air-blood barrier 4. Avoidance of first-pass metabolism Lung anatomy Respiratory tract: 1. Conducting (central) regions Responsible for air conduction Trachea, bronchi, bronchioles, terminal and respiratory bronchioles Lined with ciliated epithelial cells and mucus to trap foreign particles Lung anatomy Respiratory tract (cont’d): 2. Respiratory (peripheral) regions Responsible for gas exchange Respiratory bronchioles, alveolar regions Terminate in 2 – 6 x 108 alveoli (eq. 100 – 140 m2 surface area in adult males) Inhalation aerosols An aerosol is defined as a two phase system of solid particles or liquid droplets dispersed in air or other gaseous phase, having sufficiently small size to display considerable stability as a suspension. The deposition of a drug/ aerosol in the airways is dependent on four factors: 1. The physicochemical properties of the drug 2. The formulation 3. The delivery/liberating device 4. The patient (breathing patterns and clinical status) Inhalation aerosols The most important physical property of an aerosol for inhalation is its particle size. The particle size of an aerosol is standardized by calculation of its aerodynamic diameter, da, which is the physical diameter of a unit density sphere which settles through air with a velocity equal to the aerosol particle. For approximately spherical particles: ρ 𝑑𝑎 = 𝑑𝑝 ρ0 Where dp is physical diameter, ρ is particle density and ρ0 is unit density, i.e. 1 g/cm3. When dp is the mass median diameter (MMD), da is termed the mass median aerodynamic diameter (MMAD). Inhalation aerosols Large porous particles, with large physical diameters of the order of 20 μm are efficiently delivered to and deposited in the lungs. Their low density, due to the porous or hollow nature of their structure, means that such particles have a small aerodynamic diameter and are thus carried in the inspired air, deep into the lungs. Additionally, large particles are less prone to aggregation than smaller ones and are too large to be cleared from the airways by alveolar macrophages. Influence of environmental humidity on particle size As a particle enters the respiratory tract, the change from ambient to high relative humidity (approximately 99%) results in condensation of water onto the particle surface. For water-insoluble materials, this results in a negligibly thin film of water; however, with water-soluble materials a solution layer is formed on the particle surface that causes an increase in particle size (hygroscopic growth) Hygroscopic growth will affect the deposition of particles, resulting in deposition higher in the respiratory tract than would have been predicted from measurements of their initial size. Particle deposition in the airways Aerodynamic size > 5 μm: deposition in upper respiratory tract and mucocilliary clearance adverse effects Inhaled steroids of large size distribution can cause oral candidiasis Aerodynamic size < 5 μm: size required to penetrate the peripheral regions (fine particle fraction) Aerodynamic size < 2 μm: preferable for alveolar deposition Mechanisms of particle deposition in the airways 1. Inertial impaction Particles within the air stream, having sufficiently high momentum, will impact on the airways’ walls rather than following the changing air stream. This deposition mechanism is particularly important for large particles having a diameter greater than 5 μm, and particularly greater than 10 μm, and is common in the upper airways, being the principal mechanism for deposition in the nose, mouth, pharynx and larynx and the large conducting airways. With the continuous branching of the conducting airways, the velocity of the air stream decreases and impaction becomes a less important mechanism for deposition. Mechanisms of particle deposition in the airways 2. Gravitational sedimentation Stokes’ law: particles settling under gravity attain a constant terminal velocity (𝑉𝑡): 𝜌𝑔𝑑2 𝑉𝑡 = 18𝜂 𝜌: particle density g: gravitational constant d: particle diameter η: air viscosity Sedimentation is an important deposition mechanism for particles in the size range 1–5 μm, in the small airways and alveoli, for particles that have escaped deposition by impaction. Mechanisms of particle deposition in the airways 3. Brownian diffusion Collision and bombardment of small particles by molecules in the respiratory tract produces Brownian motion. The resultant movement of particles from high to low concentrations causes them to move from the aerosol cloud to the airways’ walls. Diffusion is inversely proportional to particle size. It is the predominant mechanism for particles smaller than 0.5 μm. Effect of particle size on deposition mechanism Different mechanisms for different sized particles: > 5 μm: inertial impaction in upper airways 1 – 5 μm: gravitational sedimentation in lower airways 0.5 – 1 μm: Brownian diffusion and sedimentation < 0.5 μm: inefficiently deposited, rapidly exhaled Breathing patterns and particle deposition Patient-dependent factors affecting particle deposition: Breathing pattern Lung physiology Pulmonary disease Larger inhaled volume: more peripheral distribution Higher inhalation flow rate: deposition in larger airways by inertial impaction Breath-holding after inhalation: sedimentation and Brownian diffusion For optimum aerosol deposition slow, deep inhalations, followed by breath-holding prior to exhalation Clearance of inhaled particles Particles deposited in the ciliated conducting airways are cleared by mucociliary clearance within 24 h and ultimately swallowed Alveolar macrophages engulf insoluble particles reaching the alveolar regions and then migrate to the mucociliary escalator or removed by the lymphatics (days – weeks) Devices for pulmonary drug delivery 1. Pressurized metered-dose inhalers (pMDIs) 2. Dry powder inhalers (DPIs) 3. Nebulizers Pressurized metered-dose inhalers (pMDIs) The most commonly used inhalation drug delivery devices Contain a drug that is dissolved or suspended in a liquid propellant ± other excipients The contents are pressurized into a canister with a metering valve Upon actuation, a metered dose is released as a spray The formulation expands in the passage within the valve forming a gas and liquid mixture before discharge from the orifice The high speed gas flow helps to break up the liquid into a fine spray of droplets Pressurized metered-dose inhalers (pMDIs) Pressurized metered-dose inhalers (pMDIs) Containers for pMDIs can be made of: Tin-plated steel Plastic-coated glass Aluminum (most common) Propellants used in pMDIs are typically liquefied gases Those exist as gases at RT and normal pressure, liquefy upon decreasing temp. or increasing pressure Propellants used in pMDIs Chlorofluorcarbons (CFCs) are now largely replaced by hydrofluoroalkanes (HFAs) due to their negative effect on the ozone layer The head space of the canister is filled with propellant vapor to produce the saturation vapor pressure at that temperature On spraying, the drug and propellant are expelled and the head volume increases More propellant evaporates to reestablish the equilibrium vapor pressure This ensures a constant pressure system with consistent spray characteristics Metering valve The metering valve of a pMDI permits the reproducible delivery of small volumes (25–100 μL) of product. The metering valve in pMDIs is used in the inverted position. Depression of the valve stem allows the contents of the metering chamber to be discharged through the orifice in the valve stem and made available to the patient. After actuation, the metering chamber refills with liquid from the bulk and is ready to dispense the next dose. The device needs to be primed prior to the first use by the patient. The dimensions of the orifice and the propellant vapor pressure play a crucial role in determining the shape and speed of the emitted aerosol plume. Formulation of pMDIs Pressurized aerosols may be formulated as either solutions or suspensions of drug in the liquefied propellant. For solution formulations, cosolvents may be needed (ethanol and isopropanol), but their volatility may be a problem (it increases the particle size of the aerosol droplet) Therefore, most pMDIs must be formulated as suspensions However, suspensions are harder to formulate (caking, agglomeration, particle growth, etc..). Formulation of pMDIs Factors to be considered in suspension formulations: 1. Particle size of the solid (2 – 5 μm) to prevent valve clogging 2. Solubility of the API in the propellant 3. Relative densities of API and propellant 4. Use of surfactants Filling of pMDIs Cold filling: filling the canisters by liquefying the propellant at reduced temp. Drug, excipients and propellant are chilled and filled at about –60 ºC Additional propellant is added as needed and the canister sealed with the valve at the same temp. Pressure filling: filling the vapor at elevated pressure A drug/propellant (CFC-11) concentrate is produced and filled at RT and pressure. The valve is then crimped onto the canister Additional propellant (CFC-12) is filled at elevated pressure through the valve (gassing) HFAs are filled in a single step; the pressurized concentrate is filled through the valve, followed by adding additional propellant Filling of pMDIs After filling, the canisters are leak tested by placing them in a water bath at elevated temp. (50 – 60 ºC) The canisters are then stored to allow equilibration of the contents The contents are weighed to check for further leakage prior to spray testing and insertion into actuators Advantages of pMDIs Portable Low cost Disposable Many doses can be stored Doses are reproducible Protect drugs against oxidative degradation and microbial contamination Disadvantages of pMDIs 1. Inefficient drug delivery The first propellant droplets exit at high velocity and much of the drug can be lost upon impaction in the upper respiratory tract The mean emitted droplet size can be > 40 μm, and propellants may not evaporate quickly enough for their size to decrease for deep lung deposition 2. Incorrect use by the patient Failure to remove the protective cap covering the mouthpiece Using the inhaler in an inverted position Failure to shake the canister Failure to inhale slowly and deeply Inadequate breath-holding following inhalation Poor actuation/inhalation synchronization Spacers-actuated metered-dose inhalers Some of the disadvantages of pMDIs (inhalation/actuation coordination and the premature deposition of large droplets high in the airways) can be overcome by using extension devices or ‘spacers’ positioned between the pMDI and the patient Frequently employed with a pMDI, for administering aerosol medications to young children. Spacers-actuated metered-dose inhalers When using a spacer, the dose is discharged into a reservoir prior to inhalation, which reduces the initial droplet velocity. In addition, large droplets may be removed by impaction inside the spacer, and more efficient propellant evaporation can occur. It also eliminates the need for actuation/inhalation coordination. The spacer is added as a separate piece, or it can be built-in as an extended mouthpiece. Spacers-actuated metered-dose inhalers Breath-actuated metered-dose inhalers Breath-actuated pMDIs do not release the drug until inspiration occurs. Breath actuated devices overcome the coordination problems of conventional pMDIs and are easy to use without adding bulk to the device (no spacer needed). Dry powder inhalers (DPIs) The drug is inhaled as a cloud of fine particles Drug is either preloaded in an inhalation device or filled into hard gelatin capsules or foil blister discs loaded into a device Advantages over pMDIs 1. DPIs are propellant-free 2. Do not require an excipient other than a carrier (usually lactose) 3. Breath-actuated 4. Can deliver larger doses than pMDIs Disadvantages of DPIs 1. Liberation of powders from the device and the deaggregation of particles are limited by the patient’s ability to inhale 2. Turbulent air flow created by the increase in inhaled air velocity increases the potential for inertial impaction in upper airways 3. Exposure to ambient conditions which can affect formulation stability (pMDIs are completely sealed) 4. Less efficient at drug delivery (require double the dose of pMDIs) Formulation of DPIs Drug powders need to be micronized (~5 μm) Micronization produces powders with poor flow properties Alternative methods are spray drying, spray freeze drying, and supercritical fluid technology To improve their flow properties, poorly flowing particles are usually mixed with larger carrier particles (30 – 150 μm) e.g. lactose The small drug particles attach to the surface of the larger carrier particles This approach improves drug liberation from the inhalation device and powder filling uniformity Formulation of DPIs The turbulent air flow generated within the inhaler should be sufficient for the deaggregation of the drug/carrier aggregates Carrier particles impact in the throat, allowing the drug particles to be carried in the inhaled air deeper into the respiratory tract Adhesion and detachment of the drug particles depend on the morphology of the drug and carrier particles, and surface energies In some cases, a 3rd component is added to occupy high energy sites on the carrier particles (e.g. fine particle size lactose) Leucine or Mg stearate can also be added to modify particle adhesion Unit-dose DPIs The drug is presented in hard gelatin capsules Each capsule is placed individually into the device and then pierced by 2 metal needles Inhaled air flow through the device results in the powder being dispersed out through the perforations into the inspired air Examples: HandiHaler® and Aerolizer/ Cyclohaler® Unit-dose DPIs Multi-dose DPIs with drug in foil blisters The drug is mixed with a coarse lactose carrier and filled into an aluminum foil blister disc The disc is loaded by the patient into the device on a support wheel Each disc contains 4 – 8 doses The blisters are pierced with a needle upon mechanical leverage of the lid Air flow through the blister disperses the powder as the patient inhales through the mouthpiece The foil blisters are numbered to keep track of the dose Multi-dose DPIs with drug in foil blisters Multi-dose DPIs with drug preloaded in inhaler Newer devices have the drug preloaded into the inhaler e.g. Accuhaler® and Diskus® inhalers The drug/carrier mix is preloaded in foil-covered blister pockets containing 60 doses The foil lid is peeled off the pockets as each dose is advanced Accuhaler® and Diskhaler® are insensitive to humidity compared to hard gelatin capsule-based systems Multi-dose DPIs with drug preloaded in inhaler Accuhaler® Multi-dose DPIs with drug preloaded in inhaler Another approach is a reservoir type device Each dose is accurately measured and delivered from the drug reservoir e.g. Turbuhaler® Contains up to 200 doses of undiluted loosely aggregated micronized drug Requires a higher respiratory effort than the Diskhaler due to higher internal resistance More sensitive to humidity if not closed quickly after each use Multi-dose DPIs with drug preloaded in inhaler Nebulizers Deliver relatively large volumes of drug solutions and suspensions Used when the drug cannot be formulated as a pMDI or DPI, or when the dose is too large Drug can be inhaled during normal tidal breathing through a mouthpiece or facemask Useful for children and the elderly Categories: jet, ultrasonic, and mesh nebulizers Jet nebulizers Use compressed gas to convert a liquid into a spray The jet of high-velocity gas is passed through a narrow nozzle. The rate of gas flow driving atomization determines aerosol droplet size and drug delivery rate Ultrasonic nebulizers The energy required to atomize the liquid comes from a piezoelectric crystal vibrating at high frequency Some models have a fan to blow the droplets out In other devices the aerosol becomes available only when the patient inhales Mesh nebulizers Aerosols are generated by passing liquids through a vibrating mesh or plate with multiple apertures Vibration energy comes from a piezoelectric crystal attached to a transducer which transmits vibrations to the perforated plate with up to 6,000 holes Formulating nebulizer fluids Nebulizer fluids are typically aqueous solutions or suspensions Co-solvents such as ethanol, and surfactants may be added for suspension formulations Low pH, hypo-osmotic and hyper-osmotic solutions may cause bronchoconstriction iso-osmotic solutions of pH greater than 5 are usually used Stabilizers such as antioxidants may also be included (except sulfites are generally avoided as they cause bronchospasm Formulations are presented as sterile isotonic unit doses (1 – 2.5 mL) without a preservative Formulating nebulizer fluids Most nebulizer formulations are solutions For suspensions, mesh nebulizers are more suitable Suspensions are poorly delivered from ultrasonic nebulizers For jet nebulizers, the efficiency depends on the particle size of the micronized drug (the smaller the better) Ultrasonic nebulizers have not been successful for delivering either biopharmaceuticals or liposomes, because of denaturation resulting from the elevated temperatures produced during nebulization Mesh and jet nebulizers are used to deliver some peptides, nucleic acids and liposome formulations, although the shearing forces that occur in jet nebulizers may produce damage to some materials. Physicochemical properties of nebulizer fluids Viscosity and surface tension of the liquid being nebulized affect the output of nebulizers Aerosol droplet size is inversely proportional to viscosity for jet and mesh nebulizers Aerosol droplet size is directly proportional to viscosity in ultrasonic nebulizers A decrease in surface tension is associated with a reduction in aerosol droplet size with all devices Temperature effects during nebulization Jet nebulizers: part of the solvent evaporates with the drug, causing a decrease in the remaining solution temperature by 10 – 15 ºC This can cause complications for some asthmatic patients The cooling effect can also reduce drug solubility, and increase surface tension and viscosity In such instances, the use of an ultrasonic nebulizer may be appropriate, as the operation of such devices may increase solution temperature by up to 10– 15 °C At the same time, this increase may have detrimental effects on heat-sensitive materials e.g. proteins Mesh nebulizers have a negligible effect on fluid temperature Residual volume during nebulization Regardless of the duration of nebulization, not all the fluid can be atomized Jet and ultrasonic nebulizers are associated with a relatively large residual (dead) volume (~1 mL) Small volume nebulizer fluids may be diluted with sterile saline to minimize the loss Mesh nebulizers have a much smaller residual volume Size analysis of aerosols In vivo: Using γ-scintigraphy by radiolabeling droplets or particles with 99mTc In vitro: microscopy, laser diffraction, cascade impaction Microscopy: Laborious, hard to predict deposition in humid airways Laser diffraction: Aerosolized particles or droplets are analyzed as they pass a laser beam. Cannot measure aerodynamic properties. Operation conditions may result in solvent evaporation Cascade impactors and Impingers Cascade impactors: Comprise a series of progressively finer jets and collection plates that allow fractionation of aerosols according to their aerodynamic size distribution Large dense particles will deposit higher in the impactor, while smaller, less dense particles follow the air flow Cascade impactors and Impingers https://www.youtube.com/watch?v=zYMSEaga19M Cascade impactors and Impingers Multistage liquid impingers work on the same principle as cascade impactors For routine analysis, a glass 2-stage impinger is often employed Provides information on the fine particle fraction, Particle size distribution is better obtained using multistage impingers Cascade impactors and Impingers Disadvantages: 1. High flow rates (28 – 100 L/min) result in solvent evaporation and particles bouncing off the collection plates The plates are coated with a silicon fluid or glycerol 2. Operate at constant air flow rate Employing an electronic lung (computer-controlled piston draws air through the inhaler into the impaction sizer)