Chapter 4 Section 16.docx
Document Details
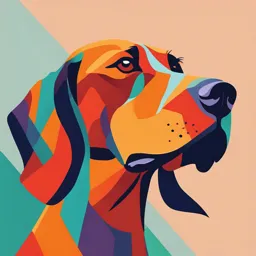
Uploaded by [email protected]
Tags
Full Transcript
Hello, aspiring aviators! Welcome back to our exploration of the Fundamentals of Flight. In our previous sessions, we soared through the details of aerodynamic efficiency and how design affects performance. Now, as we prepare for our final approach in this module, we\'ll synthesize all the aerodynam...
Hello, aspiring aviators! Welcome back to our exploration of the Fundamentals of Flight. In our previous sessions, we soared through the details of aerodynamic efficiency and how design affects performance. Now, as we prepare for our final approach in this module, we\'ll synthesize all the aerodynamic concepts we\'ve uncovered to gain a fully-formed understanding of aircraft design. Today\'s lecture will be a cumulative review, digging deeper into the core principles that enable aircraft to defy gravity and navigate the skies. By the end of this video, you\'ll have integrated knowledge essential for any pilot or aeronautical engineer, as we lead up to our comprehensive final project. Let\'s start by revisiting the airfoil -- the heart of an aircraft\'s wings. Remember, the shape of an airfoil is not a matter of aesthetic preference; it\'s the product of meticulous design aimed at achieving optimal performance. Each curve, each angle, is deliberate, with specific characteristics that influence how an aircraft interacts with the air. These shapes are the building blocks of lift -- the force that keeps planes aloft. Considering lift, we can\'t overlook how a well-designed airfoil manipulates airflow to create a pressure differential -- a concept elegantly described by Bernoulli\'s principle. Air moving over the top of the wing accelerates, leading to lower pressure compared to the slower-moving air underneath. This contrast is what gives us that uplifting force -- quite literally. Now, imagine these principles at play as an aircraft slices through the air at high speed. The design of its airfoil can make the difference between a graceful climb or a struggle against the slipstream. Speed and performance are siblings in the aerodynamic world, and the shape of your aircraft\'s wing is the family tie that binds them. A thick, cambered airfoil might offer more lift at lower speeds, ideal for a small personal aircraft. Meanwhile, a sleek, thin airfoil favors the high-speed realm of jet fighters. Next, we cannot forget the concept of the center of pressure. Like a tightrope walker\'s pole, it helps maintain the equilibrium of our aerial endeavors. This point on the aircraft\'s wing is where lift forces are said to act, in a perfectly concentrated symphony of physics. But it\'s not a stationary maestro. As the angle of attack shifts -- that is, as a plane tilts upward or downward -- the center of pressure takes a walk along the wing. Too far forward or back, and just like our tightrope walker, our aircraft might find itself off-balance. Which brings us to control. How does a pilot maintain a steady hand on the yoke when the center of pressure shifts? It\'s a delicate dance between stability and agility -- one that designers consider keenly when crafting an aircraft\'s wings. The wrong move here, and controllability takes a nosedive. So, as we pull these threads together, we create a tapestry of aerodynamic truth. We see that wing shape, lift, speed, and stability are not stand-alone ideas. They are the chorus to our flight symphony, with each element playing off the others to produce the sweet sound of controlled, purposeful flight. In our final project, you\'ll have the opportunity to apply these principles firsthand. We\'ll design airfoils, run simulations, and scrutinize how changes in design impact performance across different flight conditions. It\'s a chance to take the theoretical and make it tangible -- the ultimate test of our aerodynamic acumen. Before we land this session, let\'s recap: we\'ve reviewed airfoil designs and understood their impact on lift and speed; we\'ve balanced the shifting center of pressure within our aerodynamic focus; and we\'ve merged these aspects into a holistic approach to aircraft performance and control. As we taxi to the next phase, prepare for a hands-on approach in our upcoming video presentation: \"Airfoil Design Simulation.\" Here, you\'ll harness cutting-edge tools to test and refine your airfoil concepts. Expect an intricate venture into the role of simulation in modern aerodynamics, further equipping you with the tools to excel in aviation design. Thank you for joining me in this skyward journey. Stay curious, think critically, and always aim high. See you in the next lecture, aviators! Hello, future aviators, and welcome back to our \"Fundamentals of Flight\" course. In our previous module, we delved deep into the complex world of aerodynamic efficiency, exploring how lift, drag, and various design parameters influence an aircraft's performance. As we transition into this new module, \"Synthesis and Application,\" we gear up to combine knowledge with practice, starting with the fascinating process of airfoil design through simulation. Today's lecture is a critical one as it leads you directly into the hands-on world of aviation design. We will be exploring the essential topic of \"Airfoil Design Simulation.\" Buckle up as we prepare to use state-of-the-art simulation tools to design, test, and optimize airfoil shapes for ultimate aerodynamic efficiency. To kick things off, let\'s begin by understanding why simulation is a crucial tool in the field of aerodynamics. Real-world testing can be expensive, time-consuming, and sometimes, infeasible. This is where simulations come in. They allow us to model complex scenarios, predict the performance of airfoil designs, and make adjustments before any actual manufacturing takes place. This way, engineers and designers can ensure the efficiency and safety of their designs without the need to physically test each iteration. Now, diving into the simulation process, we start by defining our airfoil shape in a virtual environment. At this stage, our main focus will align with the learning outcome I1 - identifying the key elements of an efficient airfoil design. These include the camber, thickness, and chord length, all of which directly affect how the air flows over the wing and how much lift is produced. Next, we'll integrate the principles of lift production into the airfoil shape, adhering to learning outcome I2. It's essential to understand how changes in the airfoil\'s geometry can influence the lift-to-drag ratio, and therefore, the overall aircraft performance. This step ensures that we incorporate scientific principles into our design to meet the desired flight characteristics. Moving forward to outcome I3, our task is to optimize our airfoil for aerodynamic efficiency. Aerodynamic efficiency is how we measure the lift produced relative to the drag. An efficient airfoil is one that maximizes lift while minimizing drag, thus improving the aircraft's performance particularly in terms of speed and fuel consumption. Setting parameters in our simulation is next. From the wind speed and angle to environmental conditions such as temperature and pressure, every element can alter the results. It's crucial to define these parameters accurately to ensure a realistic and reliable simulation. Once our simulation runs its course, interpreting the results is our gateway to refinement. We'll explore how computational fluid dynamics, or CFD, comes into play. Utilizing CFD, we\'re able to see detailed flow patterns around the airfoil, identify areas of separation or turbulence, and determine the pressure distribution. This sophisticated analysis gives us unmatched insights into the aerodynamics at play. Furthermore, let's talk about how these simulations play out in the real world. Aircraft designers rely on the data to predict the behavior of new wing designs under various conditions, helming advancements in speed, efficiency, and safety in aviation. From commercial airliners to agile fighter jets, simulation is at the heart of design. Let\'s summarize what we\'ve tackled in this lecture. We\'ve seen the significance of airfoil simulations in aircraft design, understood the essential parameters and elements constituting an efficient airfoil, and learned how to utilize CFD for detailed aerodynamic analysis. Looking ahead, our next step will take us to a reading on \"Simulation in Aerodynamics,\" where we will delve into the depth of simulation\'s role beyond airfoil design, and its impact on the future of aviation. Thank you for joining me in this crucial component of aircraft design. Keep your heads in the clouds and your minds on the mechanics, and I'll see you in our next lecture. Keep soaring high! In the realm of aerodynamics, particularly in the design and analysis of airfoils, simulation plays a pivotal role. Simulation allows engineers and designers to explore the intricate behaviors of airfoils under various conditions without the need for expensive and time-consuming physical prototypes. Understanding the role of simulation requires a grasp of the variables involved. The shape of an airfoil is a critical design characteristic that directly influences its performance. Different airfoil shapes, from flat-bottomed to symmetrical or even supercritical profiles, have unique effects on the airflow around the wing. These shapes dictate parameters such as lift coefficient, drag coefficient, and critical angle of attack. Through simulation, designers can identify these design characteristics (G1) and make predictions about how they will perform in real-world scenarios. One of the primary functions of an airfoil is to generate lift. The curvature of the airfoil, the thickness distribution, and the angle of attack all contribute to this phenomenon. Simulations can assess how modifications to an airfoil\'s design alter the pressure distribution across its surface, which in turn affects lift generation (G2). By observing the lift forces in a simulated environment, designers can optimize the airfoil for maximum lift with minimal drag, contributing to more efficient flight. Aerodynamic efficiency is not just about lift; it\'s also about how the airfoil design impacts the speed and overall performance of the aircraft (G3). A well-designed airfoil will reduce drag, allowing for higher speeds and better fuel efficiency. Simulation tools enable the analysis of these performance metrics, giving insights into how the airfoil will behave at different airspeeds and flight regimes. The center of pressure is a vital concept in airfoil design, representing the point along the chord line where the total pressure forces are considered to act. Its position is crucial for maintaining aerodynamic balance and stability (H1). As the angle of attack changes, the center of pressure can shift, altering the aerodynamic forces and moments on the airfoil (H2). Simulations allow for the observation of these shifts, which is essential in predicting and ensuring stable flight characteristics. When the center of pressure moves significantly with changes in angle of attack, it can impact the controllability of the aircraft (H3). A stable design will have minimal center of pressure movement, whereas a design that allows for a large movement might offer more maneuverability but at the cost of stability. Simulation makes it possible to quantify these effects and to strike a balance that meets the desired flight characteristics. In conclusion, simulation is an indispensable tool in the field of aerodynamics. It bridges the gap between theoretical design and practical application by providing a platform to test and improve airfoil designs. With the ability to simulate complex fluid dynamics and analyze the resulting aerodynamic forces and moments, engineers can refine airfoil shapes to optimize performance, ensure stability, and ultimately contribute to the advancement of aircraft design and technology.