Flight Mechanics How Aircraft Fly Ae111 PDF
Document Details
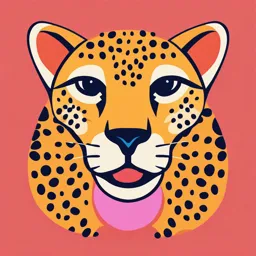
Uploaded by PrettyMoldavite9681
IPSA École d'Ingénieurs
E.Boniol
Tags
Summary
This document provides an introduction to flight mechanics, covering forces on airplanes, lift formulas, and lift coefficients. It includes illustrations and explanations of key concepts.
Full Transcript
Flight Mechanics How aircraft fly Ae111 – Introduction to Aeronautics...
Flight Mechanics How aircraft fly Ae111 – Introduction to Aeronautics E.Boniol 1 Reminder : Forces on the airplane Weight Aerodynamic forces (Lift, Drag) (= Aerodynamic resultant) Lift : Perpendicular to relative wind Drag : Parallel to relative wind Propulsive force (Thrust) ( may not exist (gliders)) Aligned with the engines axis (≈ Parallel to the Fuselage axis) E.Boniol 2 Slides named NOTE are bonus information, not to be learnt. 1 Reminder : Lift formula Unit : N (Force) Direction : Always perpendicular to the relative wind Air Density Wing area Airspeed Lift coefficient E.Boniol 3 Reminder : Lift coefficient At the heart of aerodynamics Dependancies : E.Boniol 4 Slides named NOTE are bonus information, not to be learnt. 2 Lift coefficient and AoA For small angles ( often below 15°) Around 15° (often) : maximum value for the lift coef, called 𝐶 , from which the airplane stalls. Flow separation Here, we shall deal with the Lift and Reality Drag coefficients of the complete aircraft, which are not the same as for an airfoil ! E.Boniol 5 Symmetrical Parabolic Drag polar Total Drag = Parasitic Drag + Induced Drag Zero-lift Drag coef. Lift-Dependant Drag coef. : Oswald factor. E.Boniol 6 Slides named NOTE are bonus information, not to be learnt. 3 Airplane control surfaces Vocabulary E.Boniol 7 Rotation axis Vocabulary Aircraft control is achieved around three axis : E.Boniol 8 Slides named NOTE are bonus information, not to be learnt. 4 Rotation axis & Control surfaces Vocabulary Roll : Ailerons Flight controls are used to control the Pitch : Elevator attitudes (Orientation of the aircraft) Yaw : Rudder E.Boniol 9 Some important angles 𝛼 V : Airspeed 𝜶 : Angle of Attack (Incidence en Français) 𝜷 : Angle of Sideslip (Dérapage en Français) 𝛽 E.Boniol 10 Slides named NOTE are bonus information, not to be learnt. 5 Some important angles Horizon V : Airspeed 𝜶 : Angle of Attack (Incidence en Français) Only if Bank (roll) angle is 0 ! 𝜸 : Flight path angle (fr : Pente) 𝜽 : Pitch attitude (fr : Assiette) E.Boniol 11 NOTE (previous slide) For an airfoil : AoA is often defined as the angle between the Chord and the Airspeed vector (relative wind) 𝛼 For an airplane : AoA is often defined as the angle between the longitudinal (fuselage) axis and the Airspeed vector (relative wind) 𝛼 This does not change much, AoA is just offset between the two cases (through wing structural incidence)… (fr : calage de l’aile, à ne pas confondre avec le mot français incidence, traduction française de AoA) E.Boniol 12 Slides named NOTE are bonus information, not to be learnt. 6 Flight Mechanics equations 𝑽𝒌 : Groundspeed Caution : Here, we consider the aircraft in flight, otherwise, ground reaction must be taken into account In the above formulation, the aircraft is considered as a solid, this is an approximation since : Aircraft structure is flexible CG position may change during flight (Fuel consumption, transfer and slosh) E.Boniol 13 Steady straight and level flight (Considering the Thrust vector is parallel to the Airspeed vector for simplicity) Lift 𝐿 Drag 𝐷 Thrust 𝐹⃗ 𝑚𝑔⃗ Newton’s First Law : Lift = Weight Drag = Thrust E.Boniol 14 Slides named NOTE are bonus information, not to be learnt. 7 Steady straight and level flight Influence of Airspeed V Low airspeed : to achieve , Lift must be increased through 𝐶 , thus increasing the AoA High airspeed : to achieve , There is no need for a big 𝐶 , so a small AoA is enough. But, increasing 𝐶 has a limit, which is 𝐶 , thus we can define what is called the stall speed, which represents the minimum airspeed for an aircraft to sustain straight and level flight : E.Boniol 15 Steady Climb (Considering the Thrust vector is parallel to the Airspeed vector for simplicity) Lift 𝐿 Drag 𝐷 Thrust 𝐹⃗ 𝑚𝑔⃗ There is a component of Weight slowing down the airplane. E.Boniol 16 Slides named NOTE are bonus information, not to be learnt. 8 Steady Descent (Considering the Thrust vector is parallel to the Airspeed vector for simplicity) Lift 𝐿 Drag 𝐷 Thrust 𝐹⃗ 𝑚𝑔⃗ There is a component of Weight accelerating the airplane. E.Boniol 17 Lift-to-Drag Ratio Let’s consider a total engine failure (no thrust anymore) Lift 𝐿 Let’s consider also the airplane Drag 𝐷 is descending at constant speed with a steady flight path, thus : 𝑚𝑔⃗ Lift-to-Drag ratio : Gliding distance E.Boniol 18 Slides named NOTE are bonus information, not to be learnt. 9 NOTE (previous slide) At constant airspeed (and constant wind), and constant Flight Path Angle : This is called the Lift-to-Drag ratio, and thus represents the distance an airplane can travel, for a certain initial height, after having lost its thrust. There is a maximum value for it, called the Maximum Lift-to-Drag ratio (la Finesse maximale en français), corresponding to a defined AoA value. E.Boniol 19 Pull-up (Ignoring the engines contribution & considering constant Wind) (3) (4) (2) (1) A change is the airplane AoA (2) (through the elevator creating local downward lift (1)) shall induce a higher lift force (3), leading to a change in the groundspeed vector (Newton’s 2nd Law), changing then the airspeed vector, thus the Flight path angle (4) E.Boniol 20 Slides named NOTE are bonus information, not to be learnt. 10 Take-off A pilot does not wait for the lift to be equal to the weight to lift off… The Rotation Rather : When the airplane has gained enough speed, the pilot pulls on his stick to create a down force on the tail, thus lifting the nose. Lifting the nose creates an AoA 𝜶𝒓𝒐𝒕 , that increases the Lift coef. : 𝛼 : AoA due to aircraft rotation (pitch up) Remark : 𝛼 is limited by the airplane geometry (the goal is also to avoid a tail strike) E.Boniol 21 Landing The flare To cushion the landing the pilot needs to get the vertical speed near zero ( Smooth touchdown) Increase the lift coefficient. When close to the ground, Ground effect : Increases the lift Reduces the Induced drag But reduces the stall AoA E.Boniol 22 Slides named NOTE are bonus information, not to be learnt. 11 NOTE (previous slide) From a steady descent, to cushion the landing the pilot needs to get the vertical speed near zero ( Smooth touchdown) Thus, he has to increase the lift coefficient. Just like for the take-off, he performs a flare (lifting the nose up increase the AoA increase the lift vector in order to zero the vertical descent speed) Plus, when close to the ground, the airplane shall be submitted to the Ground effect. The ground effect : Increases the lift (added pressure on the lower surface due to the air being trapped between the wing lower surface and the ground) Reduces the Induced drag (the wing tip vortices formation is prevented by the ground) But reduces the stall AoA E.Boniol 23 Load factor General idea 𝐿𝑜𝑎𝑑 𝑓𝑎𝑐𝑡𝑜𝑟 = 𝐴𝑝𝑝𝑎𝑟𝑒𝑛𝑡 𝑤𝑒𝑖𝑔ℎ𝑡 𝑚𝑔 Positive load factor : feeling of being crushed in the seat (up to the black veil, when the blood leaves the brain) Negative load factor : feeling of being pulled upwards (up to the red veil, when the blood concentrates in the brain) E.Boniol 24 Slides named NOTE are bonus information, not to be learnt. 12 NOTE (previous slide) When an airplane accelerates (changing direction, speed or while turning), which means not being at force equilibrium, the occupants will feel an apparent weight, different from their ‘true’ weight 𝑚𝑔. It must be emphasized that, physically, an acceleration is any change in the speed vector (speed value increase or decrease, or speed direction change) If the airplane accelerates upward : feeling of being crushed in the seat If the airplane accelerates downward : feeling of being pulled up from the seat E.Boniol 25 Load factor Simulation By tilting the cabin, we can use the weight vector to simulate the load factor (only up to a certain limit of course) E.Boniol 26 Slides named NOTE are bonus information, not to be learnt. 13 Load factor Definition The force equation is : (for symmetrical flight) By rearranging the terms : We get the apparent weight (which a function of acceleration) : Then, by definition of the Load factor 𝑛: Finally, the force equation is rewritten : E.Boniol 27 Load factor Let us consider, from steady horizontal flight, that the pilot acts on his stick, leading to a lift increment or decrement. When considering Thrust always compensates Drag, the force equation will then be written as : E.Boniol 28 Slides named NOTE are bonus information, not to be learnt. 14 NOTE (previous slide) When n > 1, it means the lift is greater than ‘real’ weight 𝑚𝑔 ( pull up, the pilot feels crushed in his seat) When n = 1, it means the lift equals the weight (steady horizontal flight, the pilot feels its weight just like on the ground 1 G) When n < 1, it means the lift is smaller than ‘real’ weight 𝑚𝑔 ( push on the stick, the pilot feels lighter in his seat) When n = 0, it means the lift is zero, the airplane is in free fall ( Zero-G flight, the pilot feels weightless) When n < 0, it means the lift and weight are in the same direction (the pilot feels pulled upwards) E.Boniol 29 Load factor Stall speed Let us consider, from steady horizontal flight, that the pilot pulls on his stick, leading to a lift increment. The force equation will then be written as : With n > 1. From this force equation, we can deduce the stall speed corresponding to this load factor n (the speed at which we need to reach 𝐶 to sustain the apparent weight) : E.Boniol 30 Slides named NOTE are bonus information, not to be learnt. 15 NOTE (previous slide) We can therefore see that the stall speed increases when the load factor increases (thus when the lift is forced to increase by the pilot… it is just like having to sustain a higher weight). For example, if the airplane is close to its ‘1G’ stall speed, performing a pull-up would make the airplane stall. We remind that : n is the value we consider when speaking about ‘G’ forces (1G, 2G, etc.). E.Boniol 31 The Turn To turn (to alter the trajectory of the airplane), we need a force. This force shall be directed towards the center of the turn (Centripetal force) Due to this centripetal force, an acceleration is felt by the airplane occupants in the opposite direction ( Centrifugal force, see Lecture 1). To create such a force, the lift vector is used : by banking the airplane, the lift vector is tilted to create the Centripetal force through its ‘horizontal’ component E.Boniol 32 Slides named NOTE are bonus information, not to be learnt. 16 The Turn So if the pilot banks the airplane (deflecting his stick to the desired direction), the horizontal component of the lift vector creates the centripetal force : Lift vertical Lift 𝐿 component Lift 𝐿 Lift horizontal component Centripetal force 𝑚𝑔⃗ 𝑚𝑔⃗ BUT if the process is stopped there, the vertical component of lift does not compensate the weight anymore : Lift 𝐿 The Resultant force (= 𝐿𝑖𝑓𝑡 + 𝑊𝑒𝑖𝑔ℎ𝑡) has a centripetal BUT also a downward component. 𝑅 = 𝐿 + 𝑚𝑔⃗ 𝐿 If the pilot only banks the airplane, it will go on a dive. 𝑚𝑔⃗ E.Boniol 33 The Turn To still sustain the weight, the pilot has to increase the total lift vector (pulling on his stick, increasing the lift coef.), thus increasing the load factor : Lift 𝐿 Lift 𝐿 (increased) 𝐿 𝑚𝑔⃗ 𝐿 is the vertical component of Lift, thus : 𝑚𝑔⃗ However, to make a balanced turn, we need : Lift 𝐿 𝐿 (increased) Leading to : 𝑅 = 𝐿 + 𝑚𝑔⃗ 𝑚𝑔⃗ E.Boniol 34 Slides named NOTE are bonus information, not to be learnt. 17 The Turn Overall process To make an airplane perform a balanced turn, the pilot need : To tilt his stick in the desired direction to create the centripetal force through the lift vector To pull on his stick to have the vertical component of lift still compensating the weight By doing so, the pilot will increase the load factor, which can be expressed in terms of the bank angle to have a balanced turn : It is very important to understand that this load factor is created and controlled by the pilot : if he just banks the airplane, it will go on a dive. E.Boniol 35 The Turn Load factor : Application With the elegant formula found above, we can give some example for several values of bank angle : 30° bank angle : the load factor increment is barely felt, this is suitable for a standard commercial flight. 60° bank angle : the occupants shall feel twice heavier, this may be encountered in some light airplanes. 85° bank angle : the needed load factor is way too big to be sustained by a human body during a long period. This kind of turn may also be limited by the airplane structure. E.Boniol 36 Slides named NOTE are bonus information, not to be learnt. 18 NOTE (previous slide) Let us remind the importance of the pilot here : During the turn, the pilot needs to pull the stick to prevent sideslip development and dive The turn load factor is the load factor the pilot must put through his stick so that lift still compensates weight for a given bank (roll) angle Remark : considering this with the stall speed formula with respect to the load factor found above means that an airplane is more likely to stall during a steep turn… E.Boniol 37 Flaps and Slats Flaps are aerodynamic surfaces that can be deployed at the wing trailing edge Slats are aerodynamic surfaces that can be deployed at the wing leading edge Both mean to increase the wing camber and effective surface (increasing the lift & drag), which can be very useful when flying at low airspeed (when Taking-off and Landing) E.Boniol 38 Slides named NOTE are bonus information, not to be learnt. 19 NOTE (previous slide) Remark : a big camber can induce flow separation (see Lecture 7), thus letting some airflow from the lower surface join the upper surface tend to mitigate this phenomenon by re-energizing the soon-to-revert Boundary Layer (Slats, Fowler and Double slotted flaps are « detached » from the main wing to let the lower surface airflow join the upper surface) E.Boniol 39 Flaps and slats Effects on Aerodynamic Coefficients Slats enable to delay the stall (letting lower surface airflow join the upper surface) Flaps generally increase the lift coefficient (higher camber) But due to the higher camber and wing surface, the drag is also drastically increased. This can be well suited to reduce the groundspeed before landing. E.Boniol 40 Slides named NOTE are bonus information, not to be learnt. 20 Spoilers Located on the wing upper surface, their primary goal is to force flow separation, thus creating tremendous pressure drag. They can be used : On ground, after touchdown : to increase the braking efficiency by destroying the lift, ‘pinning the airplane to the ground’ to help the airplane stop through this added pressure drag During flight (with a much smaller deflection) : To slow down the airplane To mitigate the adverse yaw during a turn (see in Aero 4) E.Boniol 41 Aircraft Centering In reality, the airplane is not a point, but a solid. Forces, when at different points on the solid, create Torques (rotation forces) Lift & Drag : at Center of Pressure ‘Fixed’ Thrust : at Center of Thrust (engines) Fixed Weight : at Center of Gravity Adjustable Adjust the CG position to balance Lift & Drag torques : Aircraft Centering E.Boniol 42 Slides named NOTE are bonus information, not to be learnt. 21 NOTE (previous slide) Thus, the 4 main forces seen above are not applied at the same location (the Weight is applied at the CG, the Lift & Drag at the Center of Pressure and the Thrust at the Center of Thrust (Engines)). Having those points in very different locations may induce torques (‘rotative forces’ on the aircraft). The positions of the Center of Pressure and the Center of Thrust are ‘fixed’. Thus, before a flight, it is very important to set the CG at a precise location, in order for the airplane to be flyable. This is the goal of aircraft centering (the CG must be within the Weight VS CG envelope) (to be seen in Aero 3) E.Boniol 43 Introduction to Flight Dynamics As indicated in the name, Flight Dynamic is the study of the aircraft motion through time. It shall be seen in details in Aero 4. It can be very useful to know the effects of the flight controls, the behaviour of the airplane after a disturbance, and building flight simulators. E.Boniol 44 Slides named NOTE are bonus information, not to be learnt. 22 Stability General notions To play on the airplane stability properties, we have two ways : Passive stabilizing techniques : airplane geometry (not using electronics to increase the stability) Active stabilizing systems (with a computer, to make some aircraft like fighter jets controllable) But a too stable plane will be felt like very heavy and not responsive when piloting, and for a not stable enough plane, loss of control can occure for the tinest control input… There is always a compromise between Stability and Maneuverability E.Boniol 45 Stability Yaw axis After some disturbance from zero sideslip angle, the vertical tailplane shall face the relative wind with a non-zero AoA. Thus, it will create a lift force (sideways), tending to restore the zero sideslip configuration. Restoring Yawing moment 𝛼 Remark : this effect is reversed if you put this vertical surface at the front (diverging yawing moment, no interest) E.Boniol 46 Slides named NOTE are bonus information, not to be learnt. 23 Stability Pitch axis After some pitch up disturbance, the horizontal tailplane shall face the relative wind with a non-zero AoA. Thus, it will create a lift force (upward), tending to restore the original AoA. Restoring Pitching moment 𝛼 Having lifting surfaces at the back increase Remark : this effect is reversed if you the stability. put this horizontal surface at the front (diverging pitching moment, This is why rockets and darts have their sometimes targeted for a fighter jet) aerodynamic surfaces at the back / bottom. E.Boniol 47 Stability Pitch axis For a civilian airliner, stability is privilieged : the pitch control surfaces shall be located at the back (Horizontal tailplane) For a fighter jet, maneuverability is privileged : the pitch control surfaces may be located at the front (Canards, like on the Rafale) (not always the case) E.Boniol 48 Slides named NOTE are bonus information, not to be learnt. 24 Stability Roll axis After a non-volontary banking of the airplane, it will start to dive (meaning having a transversal component of airspeed) Due to the presence of the fuselage that the transversal airflow must get around, the wings will face different local AoA. For a low wing design : Divergent rolling moment For a high wing design : Restoring rolling moment. Thus : Low wing airplanes are naturally unstable in roll High wing airplanes are naturally stable in roll E.Boniol 49 NOTE (previous slide) For a high wing design : The leading wing (on the left), will face a increased local AoA, contrary to the trailing wing, leading to a restoring rolling moment. For a low wing design : The leading wing (on the left), will face a decreased local AoA, contrary to the trailing wing, leading to a divergent rolling moment. E.Boniol 50 Slides named NOTE are bonus information, not to be learnt. 25 Pendulum effect : WRONG Sometimes, the stability of high wing design is explained by the fact the CG (Center of Gravity) is below the Center of Lift. Thus the lift should act as the wire of a pendulum, bringing the airplane back to its initial position. This is WRONG… It is the same mistake as saying that a rocket should be stable with the thrust vector above the CG. (see Lecture 6) Contrary to a pendulum, which is fixed at some point, the lift vector does not point towards any ‘fixed’ point. The reason for the stability of high wing devices is purely aerodynamic (presence of the fuselage) E.Boniol 51 Stability Roll axis Dihedral angle When banking of the airplane, it develops Sideslip (transversal component of airspeed) Increased local AoA Positive dihedral angle (wing angled upwards) : restoring rolling moment Increases passive roll stability Effect reversed with a negative dihedral angle (wing angled downward) E.Boniol 52 Slides named NOTE are bonus information, not to be learnt. 26 NOTE (previous slide) When banking of the airplane, it develops Sideslip (meaning having a transversal component of airspeed But, with a positive dihedral angle (wing angled upwards), the leading wing will face the transversal component with a higher angle of attack than the trailing wing, leading to a restoring rolling moment (passive roll stability). This effect is reversed with a negative dihedral angle (wing angled downward) E.Boniol 53 Stability Roll axis Dihedral angle : Examples A320 Low wing : unstable (made here to easy the maintenance of the engines) For a passenger aircraft, we need to increase its stability Positive dihedral angle ATR 72 High wing : stable For a passenger aircraft, we need to keep the stability, but keeping some manoeuverability Zero dihedral angle A400M High wing : stable For a military aircraft, we need to lower the stability, to have more manoeuverability Negative dihedral angle E.Boniol 54 Slides named NOTE are bonus information, not to be learnt. 27 Airplane proper modes What is it ? An airplane can have an oscillatory trajectory, just like a mass attached to a spring. Just like for a mass-spring system, it is useful to study the proper modes of a system. Proper-modes : Oscillations of the system when submitted to instantaneous disturbances from rest. For these proper modes, we are interested in knowing the frequency of the oscillations, and the damping / stability (Does the airplane go back to rest after some time or not ?) E.Boniol 55 Airplane proper modes Low frequency mode Longitudinal : Phugoid Slow exchanges between Kinetic and Potential energy. (this mode can have a period of several minutes for big airplanes) See https://www.youtube.com/watch?v=9DnSG4H2UjE&t=78s&ab_channel=GoldSealFlightTraining E.Boniol 56 Slides named NOTE are bonus information, not to be learnt. 28 Airplane proper modes High frequency modes Longitudinal : Short period mode Aircraft response after an instantaneous pitch disturbance (vertical wind gust for example). See https://www.youtube.com/watch?v=1O7ZqBS0_B8&ab_channel=EricJohnson Lateral : Dutch roll Oscillation on the roll and yaw axis with a 90° phase shift, may be the result of a yaw disturbance (inadvertent brief rudder input for example) See https://www.youtube.com/watch?v=NzarS6JucIs&ab_channel=TotalTrainingSupport E.Boniol 57 Airplane proper modes Low frequency mode When the airplane enters a proper oscillation, the pilot may want to correct, counteracting these oscillations. When Aircraft motion period close to human reaction time, there may be : Pilot Induced Oscillations Divergent : up to structural failure Use of indirect control laws : EFCS (Electronic Flight Control System) American Airlines 587 (Aircrash S13 E4) For example, a Yaw damper system may be used to prevent structural damage due to excessive control forces. E.Boniol 58 Slides named NOTE are bonus information, not to be learnt. 29 NOTE (previous slide) When the airplane enters a proper oscillation, the pilot may want to correct, counteracting these oscillations. But, the pilot actions may aggravate the situation, especially if the oscillation frequency is near the human reaction time. Thus, instead of mitigating the oscillations, the pilot may make them grow (resonance). This is often the case for Dutch roll : in November 2001, the copilot of an Airbus A300 caused a structural failure of the vertical tailplane when trying to counteract Dutch roll, encountered after having crossed wing tip vortices from a preceding aircraft This justifies the use of indirect control laws, through an EFCS (Electronic Flight Control System) : a computer that will mitigate the pilot actions when they endanger the aircraft. E.Boniol 59 Any question ? To go further… E.Boniol 60 Slides named NOTE are bonus information, not to be learnt. 30