Chapter 12 Section 2.docx
Document Details
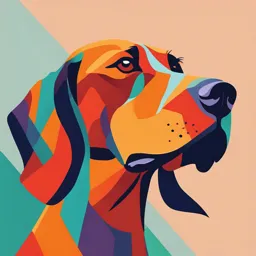
Uploaded by [email protected]
Tags
Full Transcript
As we ascend through the atmosphere, the temperature does not remain constant; it changes in a pattern that is critical for aviators to understand. The vertical temperature profile of the Earth\'s atmosphere is a fundamental concept in aviation meteorology, as it directly affects aircraft performanc...
As we ascend through the atmosphere, the temperature does not remain constant; it changes in a pattern that is critical for aviators to understand. The vertical temperature profile of the Earth\'s atmosphere is a fundamental concept in aviation meteorology, as it directly affects aircraft performance, flight planning, and safety. Starting at the surface, the lowest layer of the atmosphere is the troposphere, which extends upwards to about 8 to 15 kilometers (approximately 26,000 to 49,000 feet) depending on the latitude and season. The troposphere is characterized by a decrease in temperature with altitude, known as the environmental lapse rate. On average, the temperature drops by about 2 degrees Celsius for every 1,000 feet of elevation gain. This lapse rate can vary due to weather conditions and geographic location but is a crucial factor for determining cloud formation, weather patterns, and turbulence. Above the troposphere lies the tropopause, a transitional boundary that marks the end of the troposphere and the beginning of the stratosphere. The tropopause is significant in aviation because it typically represents the upper limit for weather phenomena and the cruising altitude for most commercial airliners. The temperature within the tropopause is relatively stable. In the stratosphere, which extends from the tropopause up to about 50 kilometers (164,000 feet), the temperature profile reverses from the troposphere. Instead of cooling, the temperature starts to increase with altitude. This increase is due to the absorption of ultraviolet (UV) radiation by the ozone layer, which protects life on Earth from harmful solar radiation. The warming effect of the stratosphere has implications for the stability of the air, which is generally much smoother than the air in the troposphere, offering a more comfortable flight experience at these altitudes. The next layer, the mesosphere, sees temperatures decreasing once again with altitude, reaching some of the coldest temperatures in the Earth\'s atmosphere. Following the mesosphere is the thermosphere, where temperatures increase dramatically due to the absorption of highly energetic solar radiation. Understanding these temperature variations is crucial for pilots for several reasons. Aircraft performance, including engine thrust, lift, and fuel efficiency, is influenced by air density, which is affected by temperature. For instance, colder temperatures typically increase air density, which can enhance aircraft performance. However, density altitude, which is pressure altitude adjusted for non-standard temperature, can also mislead pilots about the true performance capability of their aircraft in warmer conditions. Moreover, knowledge of the vertical temperature profile aids pilots in anticipating weather-related phenomena such as icing conditions or thunderstorm activity, which are predominantly found in the troposphere. It also helps in understanding the flight environment, such as why jet streams occur at the tropopause and why smoother flights are often found in the lower stratosphere. In summary, the vertical temperature variations in the atmosphere are a key element of aviation weather that pilots must master to ensure safe and efficient flight operations. Whether it\'s planning for potential weather encounters or calculating aircraft performance, temperature plays a pivotal role in the complex interplay of factors that dictate flight in the Earth\'s atmosphere. Hello everyone, and welcome back to \"Aviation Weather Fundamentals: Mastering Meteorology for Safe Flight.\" If you recall, in our previous lessons, we\'ve begun to unravel the complexities of the atmosphere and how weather patterns emerge. Now, we will delve into a fascinating phenomenon that plays a crucial role in shaping our planet\'s climate and our experience as pilots: the Coriolis force. Today, in this module titled \"Understanding the Atmosphere\", we\'re focusing on the Coriolis force and its effect on air movement, a concept vital for every aspiring commercial pilot to grasp. Understanding the Coriolis effect is instrumental in predicting weather patterns and ensuring safe flight operations. Let\'s dive right in. The Coriolis force is an apparent force that arises from Earth\'s rotation. It affects the movement of objects, such as air masses, making them appear to turn to the right in the Northern Hemisphere and to the left in the Southern Hemisphere. This deflection occurs because the Earth rotates underneath the moving air, creating a difference in velocity that alters the air\'s direction. Imagine standing at the equator; you\'re rotating at about 1,675 kilometers per hour with the Earth. However, if you move northward or southward, the circumference of the Earth shrinks, and your rotational speed changes. Air traveling long distances across latitudes experiences this difference in the Earth\'s rotational speed, leading to the Coriolis effect. This invisible force has a profound impact on global weather patterns and wind. For instance, it helps form the trade winds, westerlies, and polar easterlies, which are essential components of the global circulation system. These large-scale wind patterns are what drive weather systems across continents and oceans. On your screen now, you\'ll see a depiction of the Coriolis effect in action. Notice how air moving from high-pressure areas in higher latitudes spirals outwards and is deflected, while air moving towards low-pressure zones in lower latitudes spirals inwards and is also deflected. This effect creates swirling weather patterns such as cyclones and anticyclones. It\'s important to underline that the Coriolis effect varies with latitude. It is strongest near the poles and weakest at the equator. In fact, right on the equator, the Coriolis force is not felt at all, offering unique challenges and considerations for pilots operating in these regions. As pilots, understanding the Coriolis force helps us predict wind direction and speed, which are crucial for navigational purposes and flight planning. For example, planning a route across the Atlantic involves accounting for the westerlies that could either impede or facilitate our flight, depending on their direction and our intended route. Furthermore, comprehending the Coriolis effect allows us to understand jet streams, the narrow bands of strong winds high in the atmosphere that significantly influence flight time, fuel consumption, and overall safety. In contrast, at different latitudes, the limitations of the Coriolis force become apparent. For flights near the equator, where the Coriolis effect is minimal, wind patterns are influenced more by thermal processes and local geography than by Earth\'s rotation. To summarize, the Coriolis effect plays a central role in the movement of air masses and the weather patterns we observe. This knowledge aids us greatly in safe navigation and precise flight planning. As we continue on our journey through atmospheric science, keep in mind the omnipresence of the Coriolis force and the impact it has on everything from the smallest breeze to the most powerful storm systems. Next, we\'ll be moving on to an insightful reading on pressure systems and flight safety, where you\'ll learn about how high and low-pressure systems can affect your flying experience. It\'s crucial to review this material as it builds on what we've covered and sets the stage for safely piloting an aircraft through diverse weather conditions. Thank you for your attention, and I look forward to exploring pressure systems with you in our next lesson. Until then, clear skies and safe flying! Air pressure systems are a fundamental aspect of meteorology that have a direct impact on aviation and flight safety. These systems are defined by the atmospheric pressure within a certain area and are categorized as either high-pressure systems (anticyclones) or low-pressure systems (cyclones). Understanding these systems is crucial for pilots, as they can significantly affect flight conditions, aircraft performance, and safety. High-pressure systems are areas where the atmospheric pressure is higher than the surrounding environment. Air within a high-pressure system tends to descend and spread out as it reaches the ground, leading to generally stable and clear weather conditions. Skies are often clear or partly cloudy, and wind speeds are usually moderate. For pilots, high-pressure systems can lead to favorable flying conditions; however, they must be mindful of potential issues such as frost in cold weather or haze that can reduce visibility. Low-pressure systems, on the other hand, are characterized by atmospheric pressure that is lower than that of the surrounding areas. Air flows towards the low-pressure zone and, due to the Coriolis effect, spirals inward and upward. This upward movement of air can lead to the formation of clouds and precipitation, making low-pressure systems associated with more unsettled weather conditions, including storms, strong winds, and heavy rain. For aviation, these systems pose numerous hazards. Pilots must navigate turbulence, wind shear, and poor visibility---all of which can challenge even the most experienced aviators. When flying near or through a low-pressure system, pilots must be particularly vigilant. Weather briefings and forecasts become essential tools in planning a safe flight path. In-flight, pilots may need to alter course to avoid the worst of the weather or make the decision to delay or reroute a flight for safety. Pressure systems also influence wind patterns, which are another critical factor in aviation safety. In high-pressure systems, winds move clockwise and outward in the Northern Hemisphere, and counterclockwise in the Southern Hemisphere. The reverse is true for low-pressure systems, where winds move counterclockwise and inward in the Northern Hemisphere and clockwise in the Southern Hemisphere. Understanding these wind patterns helps pilots anticipate wind direction and speed for takeoffs, landings, and en-route flight planning. Additionally, the transition zones between high and low-pressure systems, known as pressure gradients, are areas where the wind speeds can increase significantly. Pilots need to be aware of these gradients as they can lead to challenging flying conditions, particularly if the gradient is steep, indicating a rapid change in pressure over a short distance. In conclusion, pressure systems play a critical role in determining weather patterns and, by extension, flight safety. Pilots must have a thorough understanding of how these systems work, how to interpret meteorological information related to them, and how to apply this knowledge to make informed decisions during flight planning and execution. By doing so, they can minimize the risks associated with adverse weather conditions and ensure the safety of their aircraft and passengers.