Chapter 12.pdf Intracellular Organization and Protein Sorting
Document Details
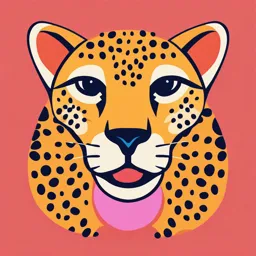
Uploaded by CalmFairy
جامعة البترا-الأردن & كلية الطب-جامعة الأزهر-مصر
Tags
Related
- Molecular Biology of the Cell Chapter 12 Intracellular Organization and Protein Sorting (Part 2) PDF
- Chapter 12 Intracellular Compartments and Protein Sorting Biology PDF
- Organelle Organization (PDF)
- Organelle Organization PDF
- Intracellular Organization and Protein Sorting PDF
- Intracellular Organization and Protein Sorting PDF
Summary
This document details the intracellular organization and protein sorting pathways in eukaryotic cells. It discusses various methods of protein transport, including protein translocation, gated transport, and vesicular transport, and includes diagrams to illustrate these processes.
Full Transcript
Chapter 12 Intracellular Organization and Protein Sorting Copyright © 2022 W. W. Norton & Company, Inc. The major intracellular compartments common to eukaryotic cells. The nucleus contains the genome. Cytoplasm consists of the cytosol and the cytoplasmic organelles suspended in it. The...
Chapter 12 Intracellular Organization and Protein Sorting Copyright © 2022 W. W. Norton & Company, Inc. The major intracellular compartments common to eukaryotic cells. The nucleus contains the genome. Cytoplasm consists of the cytosol and the cytoplasmic organelles suspended in it. The cytosol constitutes a little more than half the total volume of the cell, and it is the main site of protein synthesis and degradation. The endoplasmic reticulum (ER): performs most of the cell’s intermediary metabolism. About half the total area of the membrane in a eukaryotic cell encloses the labyrinthine spaces of ER rough ER.& smooth ER. Golgi apparatus, disc-like compartments called Golgi cisternae, receives lipids and proteins from the ER and dispatches them to various destinations, usually covalently modifying them en route. Lysosomes contain digestive enzymes that degrade defunct intracellular organelles, Mitochondria and chloroplasts generate most of the ATP that cells use to drive reactions requiring an input of free energy. Peroxisomes are small vesicular compartments that contain enzymes used in various oxidative reactions. Each membrane-enclosed organelle performs the same set of basic functions in all cell types. These organelles vary in abundance and can have additional properties that differ from cell type to cell type. Road map of protein traffic Proteins Can Move Between Compartments in Different Ways Nearly all proteins, (except a few inside mitochondria and plastids), begin their synthesis on ribosomes in the cytosol. The final location of each protein depends on its amino acid sequence, which can contain one or more sorting signals that direct its delivery to different parts of the cell. The sorting signals in the transported protein are recognized by complementary sorting receptors that mediate movement between compartments. Proteins that do not have any sorting signals remain in the cytosol as permanent residents. Trafficking ways of proteins There are four fundamentally different ways a protein is moved from one compartment to another. 1. In protein translocation, transmembrane protein translocators : Directly transport specific proteins from the cytosol into a topologically distinct space: either the other side of a membrane or within the lipid bilayer in the case of integral membrane proteins. The transported protein molecule usually must unfold to snake through the translocator. The initial transport of selected proteins from the cytosol into the ER lumen, the ER membrane, or mitochondria occurs in this way. 2. In gated transport, proteins and RNA molecules: Move between the cytosol and the nucleus through nuclear pore complexes in the nuclear envelope. The nuclear pore complexes function as selective gates that support the active transport of specific macromolecules and macromolecular assemblies between the two topologically equivalent spaces. 3. In vesicular transport, membrane-enclosed transport intermediates: Small, spherical transport vesicles, elongated tubules, or larger, irregularly shaped fragments of organelles—ferry proteins from one topologically equivalent compartment to another. The transport intermediate becomes loaded with a cargo of molecules derived from the lumen and membrane of the originating compartment as it buds and pinches off. At the destination compartment, the transport intermediate fuses with the compartment’s enclosing membrane to discharge its cargo. 4. In engulfment, such as autophagy: Double membrane sheets wrap around portions of the cytoplasm often including fragments of organelles or even entire organelles. This membrane structure then seals by membrane fusion to enclose a separate compartment, the autophagosome. The re-formation of the nuclear envelope after mitosis follows a conceptually similar process. ER tubes and sheets wrap around decondensing chromosomes and then fuse laterally with one another to form a sealed double-membrane envelope only traversed by the nuclear pores. Sorting Signals and Sorting Receptors Sorting signals are usually composed of amino acid side chains in a protein and come in two general varieties: signal sequence and batch sequence. 1. Signal patch: a specific three-dimensional arrangement of amino acids, Ex: nuclear and vesicular transport. 2. Signal sequence: a linear sequence of about 5–10 predominantly hydrophobic amino acids. Ex: protein translocation: The linear signal sequences for protein translocation are often found at the N-terminus of the polypeptide chain. These N-terminal signal sequences are usually removed from the finished protein by specialized signal peptidases once the sorting process is complete. Examples of signal sequences that direct proteins to different intracellular locations. Other types of signal sequences are not removed and remain part of the final mature protein. Proteins destined for mitochondria have signal sequences that include positively charged amino acids The signal for protein import into the nucleus is composed primarily of positively charged amino acids. Proteins for peroxisomes have a signal sequence of three characteristic amino acids at their C- terminus. A sorting signal for any particular destination needs to be sufficiently distinctive from all other sequences to permit its selective recognition by the appropriate sorting receptor. The Endoplasmic Reticulum (ER) The membrane of the ER typically constitutes more than half of the total membrane of an average animal cell. The ER is organized into a netlike labyrinth (irregular) of branching tubules and flattened sacs that extend throughout the cytosol. The tubules and sacs interconnect, and their membrane is continuous with the outer nuclear membrane. This membrane system encloses a single internal space, called the ER lumen, which is continuous with the space between the inner and outer nuclear membranes. The ER often occupies more than 10% of the total cell volume. The Endoplasmic Reticulum (ER) The ER has a central role in the biosynthesis of both lipids and proteins, and the ER lumen stores intracellular Ca2+ that is mobilized in many cell signaling responses. The ER membrane is the site of production of many of the transmembrane proteins and lipids of the cell’s organelles, including the ER itself, the Golgi apparatus, lysosomes, endosomes, secretory vesicles, peroxisomes, and the plasma membrane. The ER membrane is also the site at which most of the lipids for mitochondrial and plastid membranes are made. In addition, almost all of the proteins that will be secreted to the cell exterior— plus those destined for the lumen of the ER, Golgi apparatus, or lysosomes—are initially delivered to the ER lumen. The ER is Structurally and Functionally Diverse Functional specialization entails dramatic changes in the proportional abundance of different parts of the ER. These changes are observed as characteristically different types of ER membrane in different types of cells; rough ER and smooth ER. The rough appearance is due to the abundance of ribosomes bound to the surface of this part of the ER. The smooth ER lack ribosomes and are dedicated to other ER functions such as the biosynthesis and metabolism of lipids. All cells have both rough and smooth ER, but their relative abundance can vary enormously in specialized cells. Most secreted proteins are synthesized by the ribosomes that stud the surface of the rough ER. Cells specialized to secrete vast amounts of protein are packed with an abundance of rough ER. ex, antibody-secreting plasma cells and insulin-secreting β cells also contain expanded rough ER. A type of smooth ER found in all cells is called transitional ER, from which transport vesicles carrying newly synthesized proteins and lipids bud off for transport to the Golgi apparatus. ER in most eukaryotic cells is to sequester Ca2+ from the cytosol. The release of Ca2+ into the cytosol from the ER, and its subsequent reuptake, occur in many rapid responses to extracellular signals. The ER can be specialized in regions that make intimate contacts with other organelles, most notably the mitochondria, plastids, endosomes, and the plasma membrane. These organelle contact sites are enriched for proteins involved in the communication or transport of key metabolites between the juxtaposed membranes. Contact of ER with the plasma membrane modulates levels of plasma membrane phosphoinositides, which are lipids that participate in numerous signaling pathways The experimental basis for the Signal Sequences Discovery Signal sequences (and the signal sequence strategy of protein sorting) were discovered in secreted water- soluble proteins that are first translocated across the ER membrane. The signal hypothesis was formulated to explain these observations. According to this model, the mRNA for the secretory protein codes for a protein that is bigger than the protein that is eventually secreted. It was proposed that the extra polypeptide is a signal sequence that directs the secreted protein to the ER membrane. After the signal sequence has served its function, it is cleaved off by a signal peptidase in the ER membrane before the polypeptide chain has been completed. A Signal-Recognition Particle (SRP) Directs the ER Signal Sequence to a Specific Receptor at the ER The ER signal sequence is guided to the ER membrane by at least two components: a signal-recognition particle (SRP), which binds to the signal sequence, and an SRP receptor in the ER membrane. SRP is a large complex; in animal cells, it consists of six different polypeptide chains bound to a single RNA molecule. ER signal sequences vary greatly in amino acid sequence, How can SRP bind specifically to so many different sequences? The signal-recognition particle (SRP). SRP is a hinged rodlike structure that can wrap along the large ribosomal subunit. The end of SRP that contains the signal sequence–binding pocket is positioned near the ribosomal tunnel through which newly made polypeptides emerge. This allows SRP to engage a signal sequence as it emerges from the ribosome. Once SRP engages a signal sequence, the other end of SRP can bind at the interface between the large and small ribosomal subunits. This is the same site where translation elongation factors bind, so a ribosome engaged by SRP will translate proteins more slowly than normal. Slower translation presumably gives the ribosome enough time to bind to the ER membrane before completion of the polypeptide chain, thereby ensuring that the protein is not released into the cytosol. The signal-recognition particle (SRP). SRP exposes a binding site for an SRP receptor. The binding of SRP to its receptor brings the SRP–ribosome complex to an unoccupied protein translocator in the ER membrane. The part of SRP bound near the ribosomal tunnel moves to a different site, allowing the translocator to occupy this position. SRP and SRP receptor are then released, and protein synthesis resumes at full speed. The translocator, which is now tightly bound to the translating ribosome, transfers the growing polypeptide chain across the membrane Free and membrane-bound polyribosomes. Membrane-bound ribosomes, attached to the cytosolic side of the ER membrane, are engaged in the synthesis of proteins that are being concurrently translocated across the ER membrane. Free ribosomes, unattached to any membrane, synthesize all other proteins encoded by the nuclear genome. Many ribosomes can engage with a single mRNA molecule, to form a polyribosome. If the mRNA encodes a protein with an ER signal sequence, the polyribosome becomes attached to the ER membrane, directed there by the signal sequences on multiple growing polypeptide chains. The individual ribosomes associated with such an mRNA molecule can return to the cytosol when they finish translation and intermix with the pool of free ribosomes. The mRNA itself, remains attached to the ER membrane by a changing population of ribosomes, each transiently Membrane-bound and free ribosomes are structurally held at the membrane by the translocator and functionally identical, they differ only in the proteins they are making at any given time. A signal sequence opens the Sec61 translocator. The translocator: a water-filled channel across the membrane through which the polypeptide chain passes. The core of the translocator, called the Sec61 complex, is built from three subunits that are highly conserved from bacteria to eukaryotic cells. The Sec61 translocator only opens for proteins containing a signal sequence. The ability of the Sec61 translocator to recognize signal sequences provides a proofreading step to ensure that only proteins truly intended for the ER are allowed to enter. Co-translational and post-translational protein translocation. Ribosomes bind to the ER membrane during co-translational translocation Cytosolic ribosomes complete the synthesis of a protein and release it prior to post-translational translocation. The released protein is kept unfolded in the cytosol by chaperones that dissociate before the protein is translocated across the membrane. In both cases, the protein is directed to the ER by an ER signal sequence. Post-Translocation Post-translational translocation: proteins are completely synthesized in the cytosol as precursors before they are imported into the ER, demonstrating that translocation does not always require ongoing translation. Post-translational protein translocation is more common across the yeast ER membrane and the evolutionarily related bacterial plasma membrane. In both cases, the Sec61 translocator (called SecY in bacteria) is used as the translocator; its narrow channel means that precursors can only be translocated as unfolded polypeptides. Precursor proteins do not fold after their initial synthesis in the cytosol. They interact with other cytosolic proteins that prevent precursor folding or aggregation before they engage the Sec61 translocator. These interacting proteins typically are general chaperone proteins, such as those of the hsp70 family, and must dissociate as the unfolded polypeptide is threaded through the translocator. Co-translational translocation The signal peptide of a precursor directly engages the Sec61 translocator to open the channel. To pull proteins into the ER lumen, eukaryotic cells use accessory proteins called Sec62 and Sec63 that associate with the Sec61 translocator and position an hsp70-like chaperone protein (called BiP, for binding protein) adjacent to the lumenal opening of the translocation channel. BiP has a high affinity for unfolded polypeptide chains, and it binds tightly to an imported protein chain as soon as it emerges from the Sec61 translocator in the ER lumen. Tight binding by BiP prevents the protein chain from sliding backwards, favoring more of the chain to emerge into the lumen where it can bind another molecule of BiP. ATP hydrolysis by BiP causes it to release the polypeptide, making it available to bind again to any newly emerged segments of the translocating polypeptide. This energy-driven cycle of binding and release serves as a molecular ratchet that provides the driving force for protein import after a precursor has initially inserted into the Sec61 translocator. Three ways in which protein translocation can be driven through structurally similar translocators 1. Co-translational translocation: The ribosome is brought to the membrane by the SRP and SRP receptor and then engages with the Sec61 translocator. The growing polypeptide chain is threaded across the membrane as it is made. No additional energy is needed, as the only path available to the growing chain is to cross the membrane. 2. Post-translational translocation in eukaryotic cells: requires an additional complex composed of Sec62 and Sec63 proteins. This complex is attached to the Sec61 translocator and positions BiP molecules where they can bind to the translocating chain as it emerges from the translocator in the lumen of the ER. ATP-driven cycles of BiP binding and release pull the protein into the lumen. 3. Post-translational translocation in bacteria. The completed polypeptide chain is fed from the cytosolic side into the bacterial homolog of the Sec61 SecA is found exclusively in bacteria. translocator (called SecY) in the plasma membrane by the SecA ATPase. Sec62–Sec63 complex is found exclusively in eukaryotic cells.