Atoms, Molecules, and Ions PDF
Document Details
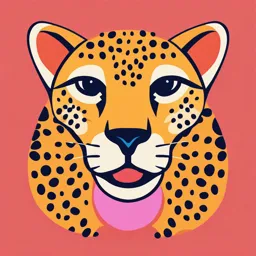
Uploaded by EasierExtraterrestrial
Tags
Related
- PiXL Independence GCSE Chemistry Past Paper PDF
- CSIR NET Unit 1(A) Notes - Structure of Atoms, Molecules & Chemical Bonds PDF
- Edexcel GCSE Chemistry Atomic Structure Notes PDF
- AQA GCSE Chemistry Past Paper - Atomic Structure and the Periodic Table PDF
- Chemistry Atomic Theories Part 1 PDF
- Atoms and Atomic Structure Lesson 2 PDF
Summary
This document is a chapter from a chemistry textbook, introducing the fundamental concepts of atoms, molecules, and ions. It covers the historical background, atomic structure, and nomenclature of chemical compounds.
Full Transcript
Atoms, Molecules, and Ions Colored images of the radioactive emission of radium (Ra). The models show the nuclei of radium and the radioactive decay products—radon (Rn) and an alpha...
Atoms, Molecules, and Ions Colored images of the radioactive emission of radium (Ra). The models show the nuclei of radium and the radioactive decay products—radon (Rn) and an alpha particle, which has two protons and two neutrons. Study of radioactivity helped to advance scientists’ knowledge about atomic structure. Chapter Outline A Look Ahead We begin with a historical perspective of the search for the fundamental units of matter. The modern version of atomic theory was laid by John 2.1 The Atomic Theory Dalton in the nineteenth century, who postulated that elements are composed 2.2 The Structure of the Atom of extremely small particles, called atoms. All atoms of a given element are 2.3 Atomic Number, Mass identical, but they are different from atoms of all other elements. (2.1) Number, and Isotopes We note that, through experimentation, scientists have learned that an atom is composed of three elementary particles: proton, electron, and neutron. The 2.4 The Periodic Table proton has a positive charge, the electron has a negative charge, and the 2.5 Molecules and Ions neutron has no charge. Protons and neutrons are located in a small region at the center of the atom, called the nucleus, while electrons are spread out 2.6 Chemical Formulas about the nucleus at some distance from it. (2.2) 2.7 Naming Compounds We will learn the following ways to identify atoms. Atomic number is the number of protons in a nucleus; atoms of different elements have different 2.8 Introduction to Organic Compounds atomic numbers. Isotopes are atoms of the same element having a different number of neutrons. Mass number is the sum of the number of protons and neutrons in an atom. Because an atom is electrically neutral, the number of protons is equal to the number of electrons in it. (2.3) Next we will see how elements can be grouped together according to their chemical and physical properties in a chart called the periodic table. The periodic table enables us to classify elements (as metals, metalloids, and nonmetals) and correlate their properties in a systematic way. (2.4) We will see that atoms of most elements interact to form compounds, which are classified as molecules or ionic compounds made of positive (cations) and negative (anions) ions. (2.5) We learn to use chemical formulas (molecular and empirical) to represent molecules and ionic compounds and models to represent molecules. (2.6) We learn a set of rules that help us name the inorganic compounds. (2.7) Finally, we will briefly explore the organic world to which we will return in a later chapter. (2.8) Student Interactive Activity S ince ancient times humans have pondered the nature of matter. Our modern ideas of the structure of matter began to take shape in the early nineteenth century with Dalton’s atomic theory. We now know that all matter is made of atoms, molecules, and ions. All of chemistry is concerned in one way or another Animations with these species. Cathode Ray Tube (2.2) Millikan Oil Drop (2.2) Alpha, Beta, and Gamma Rays (2.2) a-Particle Scattering (2.2) Media Player Rutherford’s Experiment (2.2) Formation of an Ionic Compound (2.7) Chapter Summary ARIS Example Practice Problems End of Chapter Problems Quantum Tutors End of Chapter Problems 41 42 Atoms, Molecules, and Ions 2.1 The Atomic Theory In the fifth century b.c. the Greek philosopher Democritus expressed the belief that all matter consists of very small, indivisible particles, which he named atomos (mean- ing uncuttable or indivisible). Although Democritus’ idea was not accepted by many of his contemporaries (notably Plato and Aristotle), somehow it endured. Experimen- tal evidence from early scientific investigations provided support for the notion of “atomism” and gradually gave rise to the modern definitions of elements and com- pounds. In 1808 an English scientist and school teacher, John Dalton,† formulated a precise definition of the indivisible building blocks of matter that we call atoms. Dalton’s work marked the beginning of the modern era of chemistry. The hypoth- eses about the nature of matter on which Dalton’s atomic theory is based can be summarized as follows: 1. Elements are composed of extremely small particles called atoms. 2. All atoms of a given element are identical, having the same size, mass, and chemical properties. The atoms of one element are different from the atoms of all other elements. 3. Compounds are composed of atoms of more than one element. In any compound, the ratio of the numbers of atoms of any two of the elements present is either an integer or a simple fraction. 4. A chemical reaction involves only the separation, combination, or rearrangement of atoms; it does not result in their creation or destruction. Figure 2.1 is a schematic representation of the last three hypotheses. Dalton’s concept of an atom was far more detailed and specific than Democritus’. The second hypothesis states that atoms of one element are different from atoms of all other elements. Dalton made no attempt to describe the structure or composition of atoms—he had no idea what an atom is really like. But he did realize that the different properties shown by elements such as hydrogen and oxygen can be explained by assuming that hydrogen atoms are not the same as oxygen atoms. The third hypothesis suggests that, to form a certain compound, we need not only atoms of the right kinds of elements, but specific numbers of these atoms as well. † John Dalton (1766–1844). English chemist, mathematician, and philosopher. In addition to the atomic theory, he also formulated several gas laws and gave the first detailed description of color blindness, from which he suffered. Dalton was described as an indifferent experimenter, and singularly wanting in the language and power of illustration. His only recreation was lawn bowling on Thursday afternoons. Perhaps it was the sight of those wooden balls that provided him with the idea of the atomic theory. Figure 2.1 (a) According to Dalton’s atomic theory, atoms of the same element are identical, but atoms of one element are different from atoms of other elements. (b) Compound formed from atoms of elements X and Y. In this case, the ratio of the atoms of element X to the atoms of element Y is 2:1. Note that a chemical reaction results only in the rearrangement of atoms, not in their destruction or creation. Atoms of element X Atoms of element Y Compounds of elements X and Y (a) (b) 2.2 The Structure of the Atom 43 This idea is an extension of a law published in 1799 by Joseph Proust,† a French Carbon monoxide chemist. Proust’s law of definite proportions states that different samples of the same compound always contain its constituent elements in the same proportion by mass. O 1 ± ⫽ ±±± ⫽ ± C 1 Thus, if we were to analyze samples of carbon dioxide gas obtained from different sources, we would find in each sample the same ratio by mass of carbon to oxygen. It stands to reason, then, that if the ratio of the masses of different elements in a given Carbon dioxide compound is fixed, the ratio of the atoms of these elements in the compound also must be constant. O 2 ± ⫽ ±±±±±±± ⫽ ± Dalton’s third hypothesis supports another important law, the law of multiple C 1 proportions. According to the law, if two elements can combine to form more than one compound, the masses of one element that combine with a fixed mass of the other element are in ratios of small whole numbers. Dalton’s theory explains the law of Ratio of oxygen in carbon monoxide to multiple proportions quite simply: Different compounds made up of the same elements oxygen in carbon dioxide: 1:2 differ in the number of atoms of each kind that combine. For example, carbon forms two stable compounds with oxygen, namely, carbon monoxide and carbon dioxide. Figure 2.2 An illustration of the law of multiple proportions. Modern measurement techniques indicate that one atom of carbon combines with one atom of oxygen in carbon monoxide and with two atoms of oxygen in carbon dioxide. Thus, the ratio of oxygen in carbon monoxide to oxygen in carbon dioxide is 1:2. This result is consistent with the law of multiple proportions (Figure 2.2). Dalton’s fourth hypothesis is another way of stating the law of conservation of mass,‡ which is that matter can be neither created nor destroyed. Because matter is made of atoms that are unchanged in a chemical reaction, it follows that mass must be conserved as well. Dalton’s brilliant insight into the nature of matter was the main stimulus for the rapid progress of chemistry during the nineteenth century. Review of Concepts The atoms of elements A (blue) and B (orange) form two compounds shown here. Do these compounds obey the law of multiple proportions? 2.2 The Structure of the Atom On the basis of Dalton’s atomic theory, we can define an atom as the basic unit of an element that can enter into chemical combination. Dalton imagined an atom that was both extremely small and indivisible. However, a series of investigations that began in the 1850s and extended into the twentieth century clearly demonstrated that atoms actually possess internal structure; that is, they are made up of even smaller particles, which are called subatomic particles. This research led to the discovery of three such particles—electrons, protons, and neutrons. † Joseph Louis Proust (1754–1826). French chemist. Proust was the first person to isolate sugar from grapes. ‡ According to Albert Einstein, mass and energy are alternate aspects of a single entity called mass-energy. Chemical reactions usually involve a gain or loss of heat and other forms of energy. Thus, when energy is lost in a reaction, for example, mass is also lost. Except for nuclear reactions (see Chapter 23), however, changes of mass in chemical reactions are too small to detect. Therefore, for all practical purposes mass is conserved. 44 Atoms, Molecules, and Ions Figure 2.3 A cathode ray tube – with an electric field perpendicular to the direction of the cathode rays and an external magnetic A field. The symbols N and S Anode Cathode denote the north and south poles S of the magnet. The cathode rays will strike the end of the tube at B A in the presence of a magnetic field, at C in the presence of an N electric field, and at B when there are no external fields present or when the effects of the electric C field and magnetic field cancel each other. Fluorescent screen + High voltage The Electron In the 1890s, many scientists became caught up in the study of radiation, the emission and transmission of energy through space in the form of waves. Information gained from this research contributed greatly to our understanding of atomic structure. One Animation device used to investigate this phenomenon was a cathode ray tube, the forerunner of Cathode Ray Tube the television tube (Figure 2.3). It is a glass tube from which most of the air has been evacuated. When the two metal plates are connected to a high-voltage source, the negatively charged plate, called the cathode, emits an invisible ray. The cathode ray is drawn to the positively charged plate, called the anode, where it passes through a hole and continues traveling to the other end of the tube. When the ray strikes the specially coated surface, it produces a strong fluorescence, or bright light. In some experiments, two electrically charged plates and a magnet were added to the outside of the cathode ray tube (see Figure 2.3). When the magnetic field is on and the electric field is off, the cathode ray strikes point A. When only the electric field is on, the ray strikes point C. When both the magnetic and the electric fields are off or when they are both on but balanced so that they cancel each other’s influence, the ray strikes point B. According to electromagnetic theory, a moving charged body behaves like a magnet and can interact with electric and magnetic fields through which it passes. Because the cathode ray is attracted by the plate bearing positive charges and repelled by the plate bearing negative charges, it must consist of negatively charged particles. Electrons are normally associated with We know these negatively charged particles as electrons. Figure 2.4 shows the effect atoms. However, they can also be studied individually. of a bar magnet on the cathode ray. An English physicist, J. J. Thomson,† used a cathode ray tube and his knowledge of electromagnetic theory to determine the ratio of electric charge to the mass of an individual electron. The number he came up with was 21.76 3 108 C/g, where C stands for coulomb, which is the unit of electric charge. Thereafter, in a series of Animation experiments carried out between 1908 and 1917, R. A. Millikan‡ succeeded in measur- Millikan Oil Drop ing the charge of the electron with great precision. His work proved that the charge on each electron was exactly the same. In his experiment, Millikan examined the motion of single tiny drops of oil that picked up static charge from ions in the air. He suspended the charged drops in air by applying an electric field and followed their † Joseph John Thomson (1856–1940). British physicist who received the Nobel Prize in Physics in 1906 for discovering the electron. ‡ Robert Andrews Millikan (1868–1953). American physicist who was awarded the Nobel Prize in Physics in 1923 for determining the charge of the electron. 2.2 The Structure of the Atom 45 (a) (b) (c) Figure 2.4 (a) A cathode ray produced in a discharge tube. The ray itself is invisible, but the fluorescence of a zinc sulfide coating on the glass causes it to appear green. (b) The cathode ray is bent downward when a bar magnet is brought toward it. (c) When the polarity of the magnet is reversed, the ray bends in the opposite direction. motions through a microscope (Figure 2.5). Using his knowledge of electrostatics, Millikan found the charge of an electron to be 21.6022 3 10219 C. From these data he calculated the mass of an electron: charge mass of an electron 5 charge/mass 21.6022 3 10219 C 5 21.76 3 108 C/g 5 9.10 3 10228 g This is an exceedingly small mass. Radioactivity In 1895, the German physicist Wilhelm Röntgen† noticed that cathode rays caused glass and metals to emit very unusual rays. This highly energetic radiation penetrated matter, darkened covered photographic plates, and caused a variety of substances to fluoresce. Because these rays could not be deflected by a magnet, they could not contain charged particles as cathode rays do. Röntgen called them X rays because their nature was not known. † Wilhelm Konrad Röntgen (1845–1923). German physicist who received the Nobel Prize in Physics in 1901 for the discovery of X rays. Charged plate Oil droplets Figure 2.5 Schematic diagram of Millikan’s oil drop experiment. Atomizer Small hole (⫹) X ray to produce charge on oil droplet Viewing microscope (⫺) Charged plate 46 Atoms, Molecules, and Ions Figure 2.6 Three types of rays emitted by radioactive elements. b rays consist of negatively charged particles (electrons) and are therefore attracted by the positively charged plate. The opposite holds true for a rays— they are positively charged and – are drawn to the negatively α charged plate. Because g rays Lead block have no charges, their path is unaffected by an external electric field. γ β + Radioactive substance Not long after Röntgen’s discovery, Antoine Becquerel,† a professor of physics in Paris, began to study the fluorescent properties of substances. Purely by accident, he found that exposing thickly wrapped photographic plates to a certain uranium compound caused them to darken, even without the stimulation of cathode rays. Like X rays, the rays from the uranium compound were highly energetic and could not be deflected by a magnet, but they differed from X rays because they arose spontane- ously. One of Becquerel’s students, Marie Curie,‡ suggested the name radioactivity to describe this spontaneous emission of particles and/or radiation. Since then, any ele- ment that spontaneously emits radiation is said to be radioactive. Animation Three types of rays are produced by the decay, or breakdown, of radioactive Alpha, Beta, and Gamma Rays substances such as uranium. Two of the three are deflected by oppositely charged metal plates (Figure 2.6). Alpha (a) rays consist of positively charged particles, called a particles, and therefore are deflected by the positively charged plate. Beta (b) rays, or b particles, are electrons and are deflected by the negatively charged plate. The third type of radioactive radiation consists of high-energy rays called gamma (g) Positive charge spread over the entire sphere rays. Like X rays, g rays have no charge and are not affected by an external field. The Proton and the Nucleus – By the early 1900s, two features of atoms had become clear: they contain electrons, – – and they are electrically neutral. To maintain electric neutrality, an atom must contain an equal number of positive and negative charges. Therefore, Thomson proposed that – an atom could be thought of as a uniform, positive sphere of matter in which electrons are embedded like raisins in a cake (Figure 2.7). This so-called “plum-pudding” model – – was the accepted theory for a number of years. – † Antoine Henri Becquerel (1852–1908). French physicist who was awarded the Nobel Prize in Physics in 1903 for discovering radioactivity in uranium. ‡ Figure 2.7 Thomson’s model of Marie (Marya Sklodowska) Curie (1867–1934). Polish-born chemist and physicist. In 1903 she and her the atom, sometimes described French husband, Pierre Curie, were awarded the Nobel Prize in Physics for their work on radioactivity. In as the “plum-pudding” model, 1911, she again received the Nobel prize, this time in chemistry, for her work on the radioactive elements after a traditional English dessert radium and polonium. She is one of only three people to have received two Nobel prizes in science. Despite containing raisins. The electrons her great contribution to science, her nomination to the French Academy of Sciences in 1911 was rejected are embedded in a uniform, by one vote because she was a woman! Her daughter Irene, and son-in-law Frederic Joliot-Curie, shared positively charged sphere. the Nobel Prize in Chemistry in 1935. 2.2 The Structure of the Atom 47 Gold foil Figure 2.8 (a) Rutherford’s experimental design for measuring α –Particle the scattering of a particles by a emitter piece of gold foil. Most of the a particles passed through the gold foil with little or no deflection. A few were deflected at wide angles. Occasionally an a particle was turned back. (b) Magnified view of a particles passing through and Detecting screen Slit being deflected by nuclei. (a) (b) In 1910 the New Zealand physicist Ernest Rutherford,† who had studied with Animation Thomson at Cambridge University, decided to use a particles to probe the structure of a-Particle Scattering atoms. Together with his associate Hans Geiger‡ and an undergraduate named Ernest Media Player Marsden,§ Rutherford carried out a series of experiments using very thin foils of gold Rutherford’s Experiment and other metals as targets for a particles from a radioactive source (Figure 2.8). They observed that the majority of particles penetrated the foil either undeflected or with only a slight deflection. But every now and then an a particle was scattered (or deflected) at a large angle. In some instances, an a particle actually bounced back in the direction from which it had come! This was a most surprising finding, for in Thomson’s model the positive charge of the atom was so diffuse that the positive a particles should have passed through the foil with very little deflection. To quote Rutherford’s initial reaction when told of this discovery: “It was as incredible as if you had fired a 15-inch shell at a piece of tissue paper and it came back and hit you.” Rutherford was later able to explain the results of the a-scattering experiment in terms of a new model for the atom. According to Rutherford, most of the atom must be empty space. This explains why the majority of a particles passed through the gold foil with little or no deflection. The atom’s positive charges, Rutherford proposed, are all concentrated in the nucleus, which is a dense central core within the atom. Whenever an a particle came close to a nucleus in the scattering experiment, it experienced a large repulsive force and therefore a large deflection. Moreover, an a particle traveling directly toward a nucleus would be completely repelled and its direction would be reversed. The positively charged particles in the nucleus are called protons. In separate experiments, it was found that each proton carries the same quantity of charge as an electron and has a mass of 1.67262 3 10224 g—about 1840 times the mass of the oppositely charged electron. At this stage of investigation, scientists perceived the atom as follows: The mass of a nucleus constitutes most of the mass of the entire atom, but the nucleus occupies only about 1/1013 of the volume of the atom. We express atomic (and molecular) A common non-SI unit for atomic length is dimensions in terms of the SI unit called the picometer (pm), where the angstrom (Å; 1 Å = 100 pm). 1 pm 5 1 3 10212 m † Ernest Rutherford (1871–1937). New Zealand physicist. Rutherford did most of his work in England (Manchester and Cambridge Universities). He received the Nobel Prize in Chemistry in 1908 for his investigations into the structure of the atomic nucleus. His often-quoted comment to his students was that “all science is either physics or stamp-collecting.” ‡ Johannes Hans Wilhelm Geiger (1882–1945). German physicist. Geiger’s work focused on the structure of the atomic nucleus and on radioactivity. He invented a device for measuring radiation that is now com- monly called the Geiger counter. § Ernest Marsden (1889–1970). English physicist. It is gratifying to know that at times an undergraduate can assist in winning a Nobel Prize. Marsden went on to contribute significantly to the development of science in New Zealand. 48 Atoms, Molecules, and Ions A typical atomic radius is about 100 pm, whereas the radius of an atomic nucleus is only about 5 3 1023 pm. You can appreciate the relative sizes of an atom and its nucleus by imagining that if an atom were the size of a sports stadium, the volume of its nucleus would be comparable to that of a small marble. Although the protons are confined to the nucleus of the atom, the electrons are conceived of as being spread out about the nucleus at some distance from it. The concept of atomic radius is useful experimentally, but we should not infer that atoms have well-defined boundaries or surfaces. We will learn later that the outer If the size of an atom were expanded to regions of atoms are relatively “fuzzy.” that of this sports stadium, the size of the nucleus would be that of a marble. The Neutron Rutherford’s model of atomic structure left one major problem unsolved. It was known that hydrogen, the simplest atom, contains only one proton and that the helium atom contains two protons. Therefore, the ratio of the mass of a helium atom to that of a hydrogen atom should be 2:1. (Because electrons are much lighter than protons, their contribution to atomic mass can be ignored.) In reality, however, the ratio is 4:1. Rutherford and others postulated that there must be another type of subatomic par- ticle in the atomic nucleus; the proof was provided by another English physicist, James Chadwick,† in 1932. When Chadwick bombarded a thin sheet of beryllium with a particles, a very high-energy radiation similar to g rays was emitted by the metal. Later experiments showed that the rays actually consisted of a third type of subatomic particles, which Chadwick named neutrons, because they proved to be electrically neutral particles having a mass slightly greater than that of protons. The mystery of the mass ratio could now be explained. In the helium nucleus there are two protons and two neutrons, but in the hydrogen nucleus there is only one proton and no neutrons; therefore, the ratio is 4:1. Figure 2.9 shows the location of the elementary particles (protons, neutrons, and electrons) in an atom. There are other subatomic particles, but the electron, the proton, † James Chadwick (1891–1972). British physicist. In 1935 he received the Nobel Prize in Physics for proving the existence of neutrons. Figure 2.9 The protons and neutrons of an atom are packed in an extremely small nucleus. Electrons are shown as “clouds” around the nucleus. Proton Neutron 2.3 Atomic Number, Mass Number, and Isotopes 49 TABLE 2.1 Mass and Charge of Subatomic Particles Charge Particle Mass (g) Coulomb Charge Unit Electron* 9.10938 3 10228 21.6022 3 10219 21 Proton 1.67262 3 10224 11.6022 3 10219 11 Neutron 1.67493 3 10224 0 0 *More refined measurements have given us a more accurate value of an electron’s mass than Millikan’s. and the neutron are the three fundamental components of the atom that are important in chemistry. Table 2.1 shows the masses and charges of these three elementary particles. 2.3 Atomic Number, Mass Number, and Isotopes All atoms can be identified by the number of protons and neutrons they contain. The atomic number (Z) is the number of protons in the nucleus of each atom of an ele- ment. In a neutral atom the number of protons is equal to the number of electrons, so the atomic number also indicates the number of electrons present in the atom. The chemical identity of an atom can be determined solely from its atomic number. For example, the atomic number of fluorine is 9. This means that each fluorine atom has 9 protons and 9 electrons. Or, viewed another way, every atom in the universe that contains 9 protons is correctly named “fluorine.” The mass number (A) is the total number of neutrons and protons present in the nucleus of an atom of an element. Except for the most common form of hydrogen, which has one proton and no neutrons, all atomic nuclei contain both protons and neutrons. In general, the mass number is given by mass number 5 number of protons 1 number of neutrons (2.1) 5 atomic number 1 number of neutrons The number of neutrons in an atom is equal to the difference between the mass num- Protons and neutrons are collectively called nucleons. ber and the atomic number, or (A 2 Z). For example, if the mass number of a par- ticular boron atom is 12 and the atomic number is 5 (indicating 5 protons in the nucleus), then the number of neutrons is 12 2 5 5 7. Note that all three quantities (atomic number, number of neutrons, and mass number) must be positive integers, or whole numbers. Atoms of a given element do not all have the same mass. Most elements have two or more isotopes, atoms that have the same atomic number but different mass numbers. For example, there are three isotopes of hydrogen. One, simply known as hydrogen, has one proton and no neutrons. The deuterium isotope contains one pro- ton and one neutron, and tritium has one proton and two neutrons. The accepted way to denote the atomic number and mass number of an atom of an element (X) is as follows: mass number 8n A ZX atomic number 8n 50 Atoms, Molecules, and Ions Thus, for the isotopes of hydrogen, we write 1 2 3 1H 1H 1H hydrogen deuterium tritium 1 2 3 1H 1H 1H As another example, consider two common isotopes of uranium with mass numbers of 235 and 238, respectively: 235 238 92U 92U The first isotope is used in nuclear reactors and atomic bombs, whereas the second isotope lacks the properties necessary for these applications. With the exception of hydrogen, which has different names for each of its isotopes, isotopes of elements are identified by their mass numbers. Thus, the preceding two isotopes are called uranium-235 (pronounced “uranium two thirty-five”) and uranium-238 (pronounced “uranium two thirty-eight”). The chemical properties of an element are determined primarily by the protons and electrons in its atoms; neutrons do not take part in chemical changes under nor- mal conditions. Therefore, isotopes of the same element have similar chemistries, forming the same types of compounds and displaying similar reactivities. Example 2.1 shows how to calculate the number of protons, neutrons, and elec- trons using atomic numbers and mass numbers. EXAMPLE 2.1 Give the number of protons, neutrons, and electrons in each of the following species: (a) 20 22 11Na, (b) 11Na, (c) 17 O, and (d) carbon-14. Strategy Recall that the superscript denotes the mass number (A) and the subscript denotes the atomic number (Z). Mass number is always greater than atomic number. (The only exception is 11H, where the mass number is equal to the atomic number.) In a case where no subscript is shown, as in parts (c) and (d), the atomic number can be deduced from the element symbol or name. To determine the number of electrons, remember that because atoms are electrically neutral, the number of electrons is equal to the number of protons. Solution (a) The atomic number is 11, so there are 11 protons. The mass number is 20, so the number of neutrons is 20 2 11 5 9. The number of electrons is the same as the number of protons; that is, 11. (b) The atomic number is the same as that in (a), or 11. The mass number is 22, so the number of neutrons is 22 2 11 5 11. The number of electrons is 11. Note that the species in (a) and (b) are chemically similar isotopes of sodium. (c) The atomic number of O (oxygen) is 8, so there are 8 protons. The mass number is 17, so there are 17 2 8 5 9 neutrons. There are 8 electrons. (d) Carbon-14 can also be represented as 14C. The atomic number of carbon is 6, so Similar problems: 2.15, 2.16. there are 14 2 6 5 8 neutrons. The number of electrons is 6. Practice Exercise How many protons, neutrons, and electrons are in the following 63 isotope of copper: Cu? Review of Concepts (a) Name the only element having an isotope that contains no neutrons. (b) Explain why a helium nucleus containing no neutrons is likely to be unstable. 2.4 The Periodic Table 51 2.4 The Periodic Table More than half of the elements known today were discovered between 1800 and 1900. During this period, chemists noted that many elements show strong simi- larities to one another. Recognition of periodic regularities in physical and chemi- cal behavior and the need to organize the large volume of available information about the structure and properties of elemental substances led to the development of the periodic table, a chart in which elements having similar chemical and phys- ical properties are grouped together. Figure 2.10 shows the modern periodic table in which the elements are arranged by atomic number (shown above the element symbol) in horizontal rows called periods and in vertical columns known as groups or families, according to similarities in their chemical properties. Note that ele- ments 112–116 and 118 have recently been synthesized, although they have not yet been named. The elements can be divided into three categories—metals, nonmetals, and met- alloids. A metal is a good conductor of heat and electricity while a nonmetal is usually a poor conductor of heat and electricity. A metalloid has properties that are intermediate between those of metals and nonmetals. Figure 2.10 shows that the 1 18 1A 8A 1 2 2 13 14 15 16 17 H 2A 3A 4A 5A 6A 7A He 3 4 5 6 7 8 9 10 Li Be B C N O F Ne 11 12 13 14 15 16 17 18 3 4 5 6 7 8 9 10 11 12 Na Mg 3B 4B 5B 6B 7B 8B 1B 2B Al Si P S Cl Ar 19 20 21 22 23 24 25 26 27 28 29 30 31 32 33 34 35 36 K Ca Sc Ti V Cr Mn Fe Co Ni Cu Zn Ga Ge As Se Br Kr 37 38 39 40 41 42 43 44 45 46 47 48 49 50 51 52 53 54 Rb Sr Y Zr Nb Mo Tc Ru Rh Pd Ag Cd In Sn Sb Te I Xe 55 56 57 72 73 74 75 76 77 78 79 80 81 82 83 84 85 86 Cs Ba La Hf Ta W Re Os Ir Pt Au Hg Tl Pb Bi Po At Rn 87 88 89 104 105 106 107 108 109 110 111 112 113 114 115 116 (117) 118 Fr Ra Ac Rf Db Sg Bh Hs Mt Ds Rg 58 59 60 61 62 63 64 65 66 67 68 69 70 71 Metals Ce Pr Nd Pm Sm Eu Gd Tb Dy Ho Er Tm Yb Lu 90 91 92 93 94 95 96 97 98 99 100 101 102 103 Metalloids Th Pa U Np Pu Am Cm Bk Cf Es Fm Md No Lr Nonmetals Figure 2.10 The modern periodic table. The elements are arranged according to the atomic numbers above their symbols. With the exception of hydrogen (H), nonmetals appear at the far right of the table. The two rows of metals beneath the main body of the table are conventionally set apart to keep the table from being too wide. Actually, cerium (Ce) should follow lanthanum (La), and thorium (Th) should come right after actinium (Ac). The 1–18 group designation has been recommended by the International Union of Pure and Applied Chemistry (IUPAC) but is not yet in wide use. In this text, we use the standard U.S. notation for group numbers (1A–8A and 1B–8B). No names have yet been assigned to elements 112–116, and 118. Element 117 has not yet been synthesized. CHEMISTRY in Action Distribution of Elements on Earth and in Living Systems T he majority of elements are naturally occurring. How are these elements distributed on Earth, and which are essential to living systems? elements, we should keep in mind that (1) the elements are not evenly distributed throughout Earth’s crust, and (2) most ele- ments occur in combined forms. These facts provide the basis Earth’s crust extends from the surface to a depth of about for most methods of obtaining pure elements from their com- 40 km (about 25 mi). Because of technical difficulties, scientists pounds, as we will see in later chapters. have not been able to study the inner portions of Earth as easily The accompanying table lists the essential elements in the as the crust. Nevertheless, it is believed that there is a solid core human body. Of special interest are the trace elements, such as consisting mostly of iron at the center of Earth. Surrounding the iron (Fe), copper (Cu), zinc (Zn), iodine (I), and cobalt (Co), core is a layer called the mantle, which consists of hot fluid which together make up about 0.1 percent of the body’s mass. containing iron, carbon, silicon, and sulfur. These elements are necessary for biological functions such as Of the 83 elements that are found in nature, 12 make up growth, transport of oxygen for metabolism, and defense 99.7 percent of Earth’s crust by mass. They are, in decreasing against disease. There is a delicate balance in the amounts of order of natural abundance, oxygen (O), silicon (Si), aluminum these elements in our bodies. Too much or too little over an (Al), iron (Fe), calcium (Ca), magnesium (Mg), sodium (Na), extended period of time can lead to serious illness, retardation, potassium (K), titanium (Ti), hydrogen (H), phosphorus (P), or even death. and manganese (Mn). In discussing the natural abundance of the Mantle Essential Elements in the Human Body Crust Element Percent by Mass* Element Percent by Mass* Oxygen 65 Sodium 0.1 Carbon 18 Magnesium 0.05 Core Hydrogen 10 Iron ,0.05 Nitrogen 3 Cobalt ,0.05 Calcium 1.6 Copper ,0.05 Phosphorus 1.2 Zinc ,0.05 Potassium 0.2 Iodine ,0.05 Sulfur 0.2 Selenium ,0.01 2900 km 3480 km Chlorine 0.2 Fluorine ,0.01 Structure of Earth’s interior. * Percent by mass gives the mass of the element in grams present in a 100-g sample. (a) Natural abundance of the elements All others 5.3% in percent by mass. For example, oxy- Magnesium 2.8% gen’s abundance is 45.5 percent. This means that in a 100-g sample of Earth’s Calcium 4.7% Oxygen All others 1.2% crust there are, on the average, 45.5 g 45.5% Oxygen of the element oxygen. (b) Abundance 65% Phosphorus 1.2% Iron 6.2% of elements in the human body in per- Calcium 1.6% cent by mass. Nitrogen 3% Silicon Aluminum 8.3% Carbon 27.2% Hydrogen 10% 18% (a) (b) 52 2.5 Molecules and Ions 53 majority of known elements are metals; only 17 elements are nonmetals, and 8 ele- ments are metalloids. From left to right across any period, the physical and chemical properties of the elements change gradually from metallic to nonmetallic. Elements are often referred to collectively by their periodic table group number (Group 1A, Group 2A, and so on). However, for convenience, some element groups have been given special names. The Group 1A elements (Li, Na, K, Rb, Cs, and Fr) are called alkali metals, and the Group 2A elements (Be, Mg, Ca, Sr, Ba, and Ra) are called alkaline earth metals. Elements in Group 7A (F, Cl, Br, I, and At) are known as halogens, and elements in Group 8A (He, Ne, Ar, Kr, Xe, and Rn) are called noble gases, or rare gases. The periodic table is a handy tool that correlates the properties of the elements in a systematic way and helps us to make predictions about chemical behavior. We will take a closer look at this keystone of chemistry in Chapter 8. The Chemistry in Action essay on p. 52 describes the distribution of the elements on Earth and in the human body. Review of Concepts In viewing the periodic table, do chemical properties change more markedly across a period or down a group? 2.5 Molecules and Ions Of all the elements, only the six noble gases in Group 8A of the periodic table (He, Ne, Ar, Kr, Xe, and Rn) exist in nature as single atoms. For this reason, they are called monatomic (meaning a single atom) gases. Most matter is composed of molecules or ions formed by atoms. Molecules A molecule is an aggregate of at least two atoms in a definite arrangement held We will discuss the nature of chemical bonds in Chapters 9 and 10. together by chemical forces (also called chemical bonds). A molecule may contain atoms of the same element or atoms of two or more elements joined in a fixed ratio, in accordance with the law of definite proportions stated in Section 2.1. Thus, a mol- ecule is not necessarily a compound, which, by definition, is made up of two or more elements (see Section 1.4). Hydrogen gas, for example, is a pure element, but it con- sists of molecules made up of two H atoms each. Water, on the other hand, is a molecular compound that contains hydrogen and oxygen in a ratio of two H atoms and one O atom. Like atoms, molecules are electrically neutral. The hydrogen molecule, symbolized as H2, is called a diatomic molecule because 1A H 2A 3A 4A 5A 6A 7A 8A it contains only two atoms. Other elements that normally exist as diatomic molecules N O F Cl are nitrogen (N2) and oxygen (O2), as well as the Group 7A elements—fluorine (F2), Br I chlorine (Cl2), bromine (Br2), and iodine (I2). Of course, a diatomic molecule can contain atoms of different elements. Examples are hydrogen chloride (HCl) and car- bon monoxide (CO). Elements that exist as diatomic molecules. The vast majority of molecules contain more than two atoms. They can be atoms of the same element, as in ozone (O3), which is made up of three atoms of oxygen, or they can be combinations of two or more different elements. Molecules containing more than two atoms are called polyatomic molecules. Like ozone, water (H2O) and ammonia (NH3) are polyatomic molecules. 54 Atoms, Molecules, and Ions Ions An ion is an atom or a group of atoms that has a net positive or negative charge. The number of positively charged protons in the nucleus of an atom remains the same during ordinary chemical changes (called chemical reactions), but negatively charged electrons may be lost or gained. The loss of one or more electrons from a neutral In Chapter 8, we will see why atoms of atom results in a cation, an ion with a net positive charge. For example, a sodium different elements gain (or lose) a specific number of electrons. atom (Na) can readily lose an electron to become a sodium cation, which is repre- sented by Na1: Na Atom Na1 Ion 11 protons 11 protons 11 electrons 10 electrons On the other hand, an anion is an ion whose net charge is negative due to an increase in the number of electrons. A chlorine atom (Cl), for instance, can gain an electron to become the chloride ion Cl2: Cl Atom Cl2 Ion 17 protons 17 protons 17 electrons 18 electrons Sodium chloride (NaCl), ordinary table salt, is called an ionic compound because it is formed from cations and anions. An atom can lose or gain more than one electron. Examples of ions formed by the loss or gain of more than one electron are Mg21, Fe31, S22, and N32. These ions, as well as Na1 and Cl2, are called monatomic ions because they contain only one atom. Figure 2.11 shows the charges of a number of monatomic ions. With very few exceptions, metals tend to form cations and nonmetals form anions. In addition, two or more atoms can combine to form an ion that has a net posi- tive or net negative charge. Polyatomic ions such as OH2 (hydroxide ion), CN2 (cyanide ion), and NH14 (ammonium ion) are ions containing more than one atom. 1 18 1A 8A 2 13 14 15 16 17 2A 3A 4A 5A 6A 7A Li+ C4– N3– O2– F– Na+ Mg2+ 3 4 5 6 7 8 9 10 11 12 Al3+ P3– S2– Cl– 3B 4B 5B 6B 7B 8B 1B 2B Cr 2+ Mn2+ Fe2+ Co2+ Ni2+ Cu+ K+ Ca2+ Zn2+ Se2– Br– Cr 3+ Mn3+ Fe3+ Co3+ Ni3+ Cu2+ Sn2+ Rb+ Sr2+ Ag+ Cd2+ Te2– I– Sn4+ Au+ Hg2+ 2 Pb2+ Cs+ Ba2+ Au3+ Hg2+ Pb4+ Figure 2.11 Common monatomic ions arranged according to their positions in the periodic table. Note that the Hg221 ion contains two atoms. 2.6 Chemical Formulas 55 2.6 Chemical Formulas Chemists use chemical formulas to express the composition of molecules and ionic compounds in terms of chemical symbols. By composition we mean not only the ele- ments present but also the ratios in which the atoms are combined. Here we are concerned with two types of formulas: molecular formulas and empirical formulas. Molecular Formulas A molecular formula shows the exact number of atoms of each element in the small- est unit of a substance. In our discussion of molecules, each example was given with its molecular formula in parentheses. Thus, H2 is the molecular formula for hydrogen, O2 is oxygen, O3 is ozone, and H2O is water. The subscript numeral indicates the number of atoms of an element present. There is no subscript for O in H2O because there is only one atom of oxygen in a molecule of water, and so the number “one” is omitted from the formula. Note that oxygen (O2) and ozone (O3) are allotropes of oxygen. An allotrope is one of two or more distinct forms of an element. Two allo- tropic forms of the element carbon—diamond and graphite—are dramatically different not only in properties but also in their relative cost. Molecular Models Molecules are too small for us to observe directly. An effective means of visualizing them is by the use of molecular models. Two standard types of molecular models are currently in use: ball-and-stick models and space-filling models (Figure 2.12). In ball- and-stick model kits, the atoms are wooden or plastic balls with holes in them. Sticks or springs are used to represent chemical bonds. The angles they form between atoms approximate the bond angles in actual molecules. With the exception of the H atom, the balls are all the same size and each type of atom is represented by a specific color. See back endpaper for color codes for In space-filling models, atoms are represented by truncated balls held together by snap atoms. Hydrogen Water Ammonia Methane Molecular H2 H2O NH3 CH4 formula H W Structural H±H H±O±H H±N±H H±C±H formula W W H H Ball-and-stick model Space-filling model Figure 2.12 Molecular and structural formulas and molecular models of four common molecules. 56 Atoms, Molecules, and Ions fasteners, so that the bonds are not visible. The balls are proportional in size to atoms. The first step toward building a molecular model is writing the structural formula, which shows how atoms are bonded to one another in a molecule. For example, it is known that each of the two H atoms is bonded to an O atom in the water molecule. Therefore, the structural formula of water is H}O}H. A line connecting the two atomic symbols represents a chemical bond. Ball-and-stick models show the three-dimensional arrangement of atoms clearly, and they are fairly easy to construct. However, the balls are not proportional to the size of atoms. Furthermore, the sticks greatly exaggerate the space between atoms in a molecule. Space-filling models are more accurate because they show the variation in atomic size. Their drawbacks are that they are time-consuming to put together and they do not show the three-dimensional positions of atoms very well. We will use both models extensively in this text. Empirical Formulas The molecular formula of hydrogen peroxide, a substance used as an antiseptic and as a bleaching agent for textiles and hair, is H2O2. This formula indicates that each hydrogen peroxide molecule consists of two hydrogen atoms and two oxygen atoms. The ratio of hydrogen to oxygen atoms in this molecule is 2:2 or 1:1. The empirical formula of hydrogen peroxide is HO. Thus, the empirical formula tells us which elements are pres- ent and the simplest whole-number ratio of their atoms, but not necessarily the actual number of atoms in a given molecule. As another example, consider the compound hydrazine (N2H4), which is used as a rocket fuel. The empirical formula of hydrazine is H2O2 NH2. Although the ratio of nitrogen to hydrogen is 1:2 in both the molecular formula (N2H4) and the empirical formula (NH2), only the molecular formula tells us the actual number of N atoms (two) and H atoms (four) present in a hydrazine molecule. The word “empirical” means “derived from Empirical formulas are the simplest chemical formulas; they are written by reduc- experiment.” As we will see in Chapter 3, empirical formulas are determined ing the subscripts in the molecular formulas to the smallest possible whole numbers. experimentally. Molecular formulas are the true formulas of molecules. If we know the molecular formula, we also know the empirical formula, but the reverse is not true. Why, then, do chemists bother with empirical formulas? As we will see in Chapter 3, when chem- ists analyze an unknown compound, the first step is usually the determination of the compound’s empirical formula. With additional information, it is possible to deduce the molecular formula. For many molecules, the molecular formula and the empirical formula are one and the same. Some examples are water (H2O), ammonia (NH3), carbon dioxide (CO2), and methane (CH4). Examples 2.2 and 2.3 deal with writing molecular formulas from molecular mod- els and writing empirical formulas from molecular formulas. EXAMPLE 2.2 H Write the molecular formula of methanol, an organic solvent and antifreeze, from its C ball-and-stick model, shown in the margin. O Solution Refer to the labels (also see back endpapers). There are four H atoms, one C atom, and one O atom. Therefore, the molecular formula is CH4O. However, the standard way of writing the molecular formula for methanol is CH3OH because it shows Methanol how the atoms are joined in the molecule. Similar problems: 2.47, 2.48. Practice Exercise Write the molecular formula of chloroform, which is used as a solvent and a cleansing agent. The ball-and-stick model of chloroform is shown in the margin on p. 57. 2.6 Chemical Formulas 57 EXAMPLE 2.3 Cl Write the empirical formulas for the following molecules: (a) acetylene (C2H2), which is H used in welding torches; (b) glucose (C6H12O6), a substance known as blood sugar; and (c) nitrous oxide (N2O), a gas that is used as an anesthetic gas (“laughing gas”) and as C an aerosol propellant for whipped creams. Strategy Recall that to write the empirical formula, the subscripts in the molecular formula must be converted to the smallest possible whole numbers. Solution Chloroform (a) There are two carbon atoms and two hydrogen atoms in acetylene. Dividing the subscripts by 2, we obtain the empirical formula CH. (b) In glucose there are 6 carbon atoms, 12 hydrogen atoms, and 6 oxygen atoms. Dividing the subscripts by 6, we obtain the empirical formula CH2O. Note that if we had divided the subscripts by 3, we would have obtained the formula C2H4O2. Although the ratio of carbon to hydrogen to oxygen atoms in C2H4O2 is the same as that in C6H12O6 (1:2:1), C2H4O2 is not the simplest formula because its subscripts are not in the smallest whole-number ratio. (c) Because the subscripts in N2O are already the smallest possible whole numbers, the empirical formula for nitrous oxide is the same as its molecular formula. Similar problems: 2.45, 2.46. Practice Exercise Write the empirical formula for caffeine (C8H10N4O2), a stimulant found in tea and coffee. Formula of Ionic Compounds The formulas of ionic compounds are usually the same as their empirical formulas because ionic compounds do not consist of discrete molecular units. For example, a solid sample of sodium chloride (NaCl) consists of equal numbers of Na1 and Cl2 ions arranged in a three-dimensional network (Figure 2.13). In such a compound there is a 1:1 ratio of cations to anions so that the compound is electrically neutral. As you can see in Figure 2.13, no Na1 ion in NaCl is associated with just one particular Cl2 ion. In fact, each Na1 ion is equally held by six surrounding Cl2 ions and vice versa. Thus, NaCl is the empirical formula for sodium chloride. In other ionic compounds, the actual structure may be different, but the arrangement of cations and anions is such that the compounds are all electrically neutral. Note that the charges on the cation and anion are not shown in the formula for an ionic compound. Sodium metal reacting with chlorine gas to form sodium chloride. (a) (b) (c) Figure 2.13 (a) Structure of solid NaCl. (b) In reality, the cations are in contact with the anions. In both (a) and (b), the smaller spheres represent Na1 ions and the larger spheres, Cl2 ions. (c) Crystals of NaCl. 58 Atoms, Molecules, and Ions For ionic compounds to be electrically neutral, the sum of the charges on the cation and anion in each formula unit must be zero. If the charges on the cation and anion are numerically different, we apply the following rule to make the formula electrically neu- tral: The subscript of the cation is numerically equal to the charge on the anion, and the subscript of the anion is numerically equal to the charge on the cation. If the charges are numerically equal, then no subscripts are necessary. This rule follows from the fact that because the formulas of ionic compounds are usually empirical formulas, the sub- scripts must always be reduced to the smallest ratios. Let us consider some examples. Refer to Figure 2.11 for charges of cations Potassium Bromide. The potassium cation K1 and the bromine anion Br2 com- and anions. bine to form the ionic compound potassium bromide. The sum of the charges is 11 1 (21) 5 0, so no subscripts are necessary. The formula is KBr. Zinc Iodide. The zinc cation Zn21 and the iodine anion I2 combine to form zinc iodide. The sum of the charges of one Zn21 ion and one I2 ion is 12 1 (21) 5 11. To make the charges add up to zero we multiply the 21 charge of the anion by 2 and add the subscript “2” to the symbol for iodine. Therefore the formula for zinc iodide is ZnI2. Aluminum Oxide. The cation is Al31 and the oxygen anion is O22. The follow- ing diagram helps us determine the subscripts for the compound formed by the cation and the anion: Al 3 ⫹ O2⫺ Al2 O3 Note that in each of the above three exam- The sum of the charges is 2(13) 1 3(22) 5 0. Thus, the formula for aluminum ples, the subscripts are in the smallest ratios. oxide is Al2O3. EXAMPLE 2.4 Write the formula of magnesium nitride, containing the Mg21 and N32 ions. Strategy Our guide for writing formulas for ionic compounds is electrical neutrality; that is, the total charge on the cation(s) must be equal to the total charge on the anion(s). Because the charges on the Mg21 and N32 ions are not equal, we know the formula cannot be MgN. Instead, we write the formula as MgxNy, where x and y are subscripts to be determined. Solution To satisfy electrical neutrality, the following relationship must hold: (12)x 1 (23)y 5 0 Solving, we obtain x/y 5 3/2. Setting x 5 3 and y 5 2, we write When magnesium burns in air, it forms both magnesium oxide and magnesium nitride. Mg 2 ⫹ N 3 ⫺ Mg3 N2 Check The subscripts are reduced to the smallest whole number ratio of the atoms Similar problems: 2.43, 2.44. because the chemical formula of an ionic compound is usually its empirical formula. Practice Exercise Write the formulas of the following ionic compounds: (a) chromium sulfate (containing the Cr31 and SO22 4 ions) and (b) titanium oxide (containing the Ti 41 and O22 ions). 2.7 Naming Compounds 59 Review of Concepts Match each of the diagrams shown here with the following ionic compounds: Al2O3, LiH, Na2S, Mg(NO3)2. (Green spheres represent cations and red spheres represent anions.) (a) (b) (c) (d) 2.7 Naming Compounds When chemistry was a young science and the number of known compounds was small, it was possible to memorize their names. Many of the names were derived from their physical appearance, properties, origin, or application—for example, milk of magnesia, laughing gas, limestone, caustic soda, lye, washing soda, and baking soda. Today the number of known compounds is well over 20 million. Fortunately, it is not necessary to memorize their names. Over the years chemists have devised a clear system for naming chemical substances. The rules are accepted worldwide, facilitating communication among chemists and providing a useful way of labeling an overwhelming variety of substances. Mastering these rules now will prove benefi- cial almost immediately as we proceed with our study of chemistry. To begin our discussion of chemical nomenclature, the naming of chemical com- pounds, we must first distinguish between inorganic and organic compounds. Organic compounds contain carbon, usually in combination with elements such as hydrogen, oxygen, nitrogen, and sulfur. All other compounds are classified as inorganic com- pounds. For convenience, some carbon-containing compounds, such as carbon mon- oxide (CO), carbon dioxide (CO2), carbon disulfide (CS2), compounds containing the cyanide group (CN2), and carbonate (CO322) and bicarbonate (HCO32) groups are considered to be inorganic compounds. Section 2.8 gives a brief introduction to organic compounds. To organize and simplify our venture into naming compounds, we can divide For names and symbols of the elements, see front end papers. inorganic compounds into four categories: ionic compounds, molecular compounds, acids and bases, and hydrates. Ionic Compounds In Section 2.5 we learned that ionic compounds are made up of cations (positive ions) 1A 2A 3A 4A 5A 6A 7A 8A and anions (negative ions). With the important exception of the ammonium ion, NH1 4, Li N O F Na Mg Al S Cl all cations of interest to us are derived from metal atoms. Metal cations take their K Ca Br Rb Sr I names from the elements. For example, Cs Ba Element Name of Cation The most reactive metals (green) and the 1 Na sodium Na sodium ion (or sodium cation) most reactive nonmetals (blue) combine to form ionic compounds. K potassium K1 potassium ion (or potassium cation) Mg magnesium Mg21 magnesium ion (or magnesium cation) Media Player Formation of an Ionic Compound Al aluminum Al31 aluminum ion (or aluminum cation) Many ionic compounds are binary compounds, or compounds formed from just two elements. For binary compounds, the first element named is the metal cation, followed by the nonmetallic anion. Thus, NaCl is sodium chloride. The anion is named 60 Atoms, Molecules, and Ions TABLE 2.2 The “-ide” Nomenclature of Some Common Monatomic Anions According to Their Positions in the Periodic Table Group 4A Group 5A Group 6A Group 7A C carbide (C42)* N nitride (N32) O oxide (O22) F fluoride (F2) Si silicide (Si42) P phosphide (P32) S sulfide (S22) Cl chloride (Cl2) Se selenide (Se22) Br bromide (Br2) Te telluride (Te22) I iodide (I2) *The word “carbide” is also used for the anion C22 2. by taking the first part of the element name (chlorine) and adding “-ide.” Potassium bromide (KBr), zinc iodide (ZnI2), and aluminum oxide (Al2O3) are also binary com- pounds. Table 2.2 shows the “-ide” nomenclature of some common monatomic anions according to their positions in the periodic table. The “-ide” ending is also used for certain anion groups containing different ele- ments, such as hydroxide (OH2) and cyanide (CN2). Thus, the compounds LiOH and KCN are named lithium hydroxide and potassium cyanide, respectively. These and a number of other such ionic substances are called ternary compounds, meaning com- pounds consisting of three elements. Table 2.3 lists alphabetically the names of a number of common cations and anions. Certain metals, especially the transition metals, can form more than one type of cat- 3B 4B 5B 6B 7B 8B 1B 2B ion. Take iron as an example. Iron can form two cations: Fe21 and Fe31. An older nomen- clature system that is still in limited use assigns the ending “-ous” to the cation with fewer positive charges and the ending “-ic” to the cation with more positive charges: The transition metals are the elements in Fe21 ferrous ion Groups 1B and 3B–8B (see Figure 2.10). Fe31 ferric ion The names of the compounds that these iron ions form with chlorine would thus be FeCl2 ferrous chloride FeCl3 ferric chloride This method of naming ions has some distinct limitations. First, the “-ous” and “-ic” suffixes do not provide information regarding the actual charges of the two cations involved. Thus, the ferric ion is Fe31, but the cation of copper named cupric has the formula Cu21. In addition, the “-ous” and “-ic” designations provide names for only two different elemental cations. Some metallic elements can assume three or more different positive charges in compounds. Therefore, it has become increasingly common to designate different cations with Roman numerals. This is called the FeCl2 (left) and FeCl3 (right). Stock† system. In this system, the Roman numeral I indicates one positive charge, II means two positive charges, and so on. For example, manganese (Mn) atoms can assume several different positive charges: Keep in mind that the Roman numerals Mn21: MnO manganese(II) oxide refer to the charges on the metal cations. Mn31: Mn2O3 manganese(III) oxide Mn41: MnO2 manganese(IV) oxide These names are pronounced “manganese-two oxide,” “manganese-three oxide,” and “manganese-four oxide.” Using the Stock system, we denote the ferrous ion and the † Alfred E. Stock (1876–1946). German chemist. Stock did most of his research in the synthesis and char- acterization of boron, beryllium, and silicon compounds. He was the first scientist to explore the dangers of mercury poisoning. 2.7 Naming Compounds 61 TABLE 2.3 Names and Formulas of Some Common Inorganic Cations and Anions Cation Anion aluminum (Al31) bromide (Br2) ammonium (NH14) carbonate (CO22 3 ) barium (Ba21) chlorate (ClO2 3) 2 cadmium (Cd21) chloride (Cl ) calcium (Ca21) chromate (CrO22 4 ) cesium (Cs1) cyanide (CN2) chromium(III) or chromic (Cr31) dichromate (Cr2O22 7 ) cobalt(II) or cobaltous (Co21) dihydrogen phosphate (H2PO2 4) copper(I) or cuprous (Cu1) fluoride (F2) copper(II) or cupric (Cu21) hydride (H2) hydrogen (H1) hydrogen carbonate or bicarbonate (HCO2 3) iron(II) or ferrous (Fe21) hydrogen phosphate (HPO4 ) 22 iron(III) or ferric (Fe31) hydrogen sulfate or bisulfate (HSO2 4) lead(II) or plumbous (Pb21) hydroxide (OH2) lithium (Li1) iodide (I2) magnesium (Mg21) nitrate (NO2 3) manganese(II) or manganous (Mn21) nitride (N32) mercury(I) or mercurous (Hg212 )* nitrite (NO22) mercury(II) or mercuric (Hg21) oxide (O22) potassium (K1) permanganate (MnO2 4) rubidium (Rb1) peroxide (O22 2 ) silver (Ag1) phosphate (PO32 4 ) sodium (Na1) sulfate (SO224 ) strontium (Sr21) sulfide (S22) tin(II) or stannous (Sn21) sulfite (SO22 3 ) zinc (Zn21) thiocyanate (SCN2) *Mercury(I) exists as a pair as shown. ferric ion as iron(II) and iron(III), respectively; ferrous chloride becomes iron(II) chloride; and ferric chloride is called iron(III) chloride. In keeping with modern prac- tice, we will favor the Stock system of naming compounds in this textbook. Examples 2.5 and 2.6 illustrate how to name ionic compounds and write formulas for ionic compounds based on the information given in Figure 2.11 and Tables 2.2 and 2.3. EXAMPLE 2.5 Name the following compounds: (a) Cu(NO3)2, (b) KH2PO4, and (c) NH4ClO3. Strategy Note that the compounds in (a) and (b) contain both metal and nonmetal atoms, so we expect them to be ionic compounds. There are no metal atoms in (c) but there is an ammonium group, which bears a positive charge. So NH4ClO3 is also an (Continued) 62 Atoms, Molecules, and Ions ionic compound. Our reference for the names of cations and anions is Table 2.3. Keep in mind that if a metal atom can form cations of different charges (see Figure 2.11), we need to use the Stock system. Solution (a) The nitrate ion (NO23 ) bears one negative charge, so the copper ion must have two positive charges. Because copper forms both Cu1 and Cu21 ions, we need to use the Stock system and call the compound copper(II) nitrate. (b) The cation is K1 and the anion is H2PO2 4 (dihydrogen phosphate). Because potassium only forms one type of ion (K1), there is no need to use potassium(I) in the name. The compound is potassium dihydrogen phosphate. (c) The cation is NH1 2 4 (ammonium ion) and the anion is ClO3. The compound is Similar problems: 2.57(b), (e), (f). ammonium chlorate. Practice Exercise Name the following compounds: (a) PbO and (b) Li2SO3.