Prenatal Development of the Central Nervous System PDF
Document Details
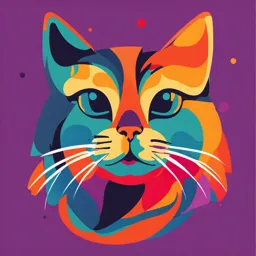
Uploaded by GenuineHarpy2986
University of Guelph
Tags
Summary
This document covers the prenatal development of the central nervous system, highlighting key stages and processes. It discusses the interaction of genes and environment, and different types of stem cells in this developmental process. The document also includes specific examples of disorders related to neuron migration.
Full Transcript
Development of the Central Nervous System Development of the Central Nervous System A. Prenatal Chapter 9 B. Postnatal Key Topics 1. Childhood 2. Adolescence 3. Adulthood C. Disorders of Neurodevelopment...
Development of the Central Nervous System Development of the Central Nervous System A. Prenatal Chapter 9 B. Postnatal Key Topics 1. Childhood 2. Adolescence 3. Adulthood C. Disorders of Neurodevelopment 1. Autism Spectrum Disorder 2. Williams Syndrome Historical View of CNS Development 1. Embryonic Development → 2. Postnatal (Childhood) Development → 3. Adulthood: brain as a stable, “fixed” organ However, we now know that the CNS continues to change throughout our entire life → Brain Plasticity Current, Comprehensive View of CNS Development 1. Embryonic Development → 2. Postnatal (Childhood) Development → 3. Adulthood: brain as a plastic organ Throughout, continued interaction between genes → environment (e.g., environment in utero, postnatal experiences) Genes and Environment: The case of “Genie” Highlights the critical importance of experience, but… Stories about children like Genie seldom give the full story. Prenatal Development of the CNS 23 pairs Gametogenesis (46 chromosomes) through Meiosis One pair shown Egg Sperm Fertilization Zygote Mitotic Divisions 1. Differentiate After (e.g., into muscle cells, liver cells, neurons, glia) formation, cells need to: 2. Migrate to the appropriate location 3. Establish functional connections with other cells 1. Induction of the Neural Plate Embryonic Development 2. Neural Proliferation of the Nervous 3. Migration and Aggregation System 4. Axon Growth and Synapse Formation 5. Neuron Death and Synapse Rearrangement 1. Induction of the Neural Plate (2) Induction initiated From the ectoderm: by chemical signals from the 1. Neural Plate 18 days Mesoderm Ectoderm 2. Neural Groove 21 days 3. Neural Tube a. Central Canal b. Neural Crest 24 days Endoderm Cellular Differentiation: Stem Cells Two properties: 1. Unlimited divisions without differentiation 2. Potential to differentiate into different cell types a. Totipotent b. Pluripotent c. Multipotent d. Unipotent (Totus = all; Pluri = many; Multi = many) COPYRIGHT © 2018 PEARSON Cellular Differentiation: Totipotent Stem Cells 1. Totipotent o Can differentiate in any cell type of the body o Early embryonic cells, up to the stage of the morula (16-32 cell stage, up to human dpf 3) o Before differentiation into 3 germ cell types Cellular Differentiation: Totipotent → Pluripotent Stem Cells 2. Pluripotent o Initial differentiation – cells can still become many, but not all, cell types o From blastula to embryonic disk stage o 3 germ cell types that can only develop into certain classes of cells What is the difference between totipotent and pluripotent stem cells? If you remove a totipotent embryonic cell and move it somewhere else to develop Forms into a new embryo (i.e., identical twins) If you remove a multipotent embryonic cell and move it somewhere else to develop Transplanting cells from different germ layers can also result in the development of tissue types where they would not normally develop (e.g., a chicken with teeth – check out this study) The mesoderm triggers development of the neural plate, so transplanting these mesoderm cells will trigger the development© Thinkstock of an additional neural plate Cellular Differentiation: Pluripotent → Multipotent Stem Cells (3) 3. Multipotent o Cells become more specialized and can only develop into multiple cell types within a class of cells o Neural tube → Neurons and glia of the CNS o Neural crest → Neurons and glia of the PNS → Epinephrine-producing cells of the adrenal medulla → Some connective tissue of the head and face Read more: “The Neural Crest” (Gilbert, 2000). 2. Neural Proliferation Neural Proliferation (2) 40 days post-fertilization, the ectodermal tissue of the neural tube forms 3 vesicles Around 50 days, 5 vesicles Neural Proliferation (3) Peak right after closure of the neural tube Most proliferation occurs in the ventricular zone of the neural tube Sequence of development is species-specific, but generally proceeds antero-caudal Regulated by chemical signals from the floor plate (along ventral surface) and roof plate (dorsal surface) of the neural tube 3. Migration Migration and o Glial-mediated Aggregation o Somal translocation Aggregation Migration Radial Tangential Multipolar (both) Guided by chemicals that either attract or repel migrating cells Two Types of Migration These neurons are not fully mature Migrate by: o Somal translocation o Glial-mediated locomotion Aggregation Cell adhesion molecules (CAMs) o On the surface of neurons and glia o Involved in migration, recognition, and adhesion Gap junctions o Formed by connexins arranged in a connexon o Two adjoining connexons form a gap junction Glia-neuron interactions Disorders of Neuron Migration Kallmann Syndrome: Abnormal genitals and dysfunctional sense of smell related to failed migration of neurons secreting sex hormones and coding for odors. Genetic mutation that codes a faulty CAM protein (MacColl et al., 2002). Lissencephaly: “Smooth brain,” caused by defects in the cytoskeleton that neurons need for migration (Kato & Dobyns, 2003). Schizophrenia, Bipolar Disorder, Autism Spectrum Disorder (Fukuda & Yanagi, 2017) and Dyslexia* (Carrion-Castillo et al., 2013; Guidi et al., 2018) 4. Axon Growth and Synapse Formation Begins with the axon growth cone oAt the tip of a growing axon, it extends and retracts filopodia (finger- like processes) 1. Chemoaffinity Hypothesis How do the growth cones 2. Topographic Gradient Hypothesis find their way to their target? Chemoaffinity Hypothesis Target-specific chemical labels → axons grow into the chemical gradient Hypothesis supported by: o In vitro studies (no spatial cues, only chemical) o Discovery of several chemical labels that attract or repel neurons Evidence for the Chemoaffinity Hypothesis: Sperry’s Study of Eye Rotation and Regeneration N.B.: ▪ The frog optic tectum is homologous to the mammalian superior colliculus ▪ Retinal ganglion cell regeneration does not occur in mammals Evidence that Contradicts the Chemoaffinity Hypothesis The Chemoaffinity Hypothesis is not supported by studies showing: 1. Targets transplanted in new positions can become incorrectly innervated. Example: Stirling & Summerbell, 1988 In chicken embryos, motor neurons from the posterior wing were grafted to the anterior part of the wing. Instead of connecting with their target muscles on the posterior wing, they extended both the anterior and posterior with no clear target. 2. The route to the target is often circuitous, rather than linear. Normal wing Grafted wing ?? Revised Chemoaffinity Hypothesis 1. Pioneer growth cones follow CAMs and other guidance molecules (chemical trails) 2. Subsequent growth cones follow the pioneer growth cones via fasciculation Fasciculation = the tendency of growing axons to follow previous axons CAMs = Cell adhesion molecules Fasciculus = bundle Topographic Gradient Hypothesis Two intersecting gradients (up-down and left-right) of chemicals on the originating tissue guide axonal growth from one topographic array (such as a retina) to another (the optic tectum) Hypothesis supported by: o Maintenance of topographic integrity © 2001 Allyn & Bacon Evidence for the Topographic Gradient Hypothesis: Topographic Integrity in the Frog Retina Chemoaffinity Hypothesis? So which hypothesis is Topographic Gradient Hypothesis? correct? Both! Synapse Formation Synapse formation = Synaptogenesis Requires neuron-neuron “talk” Glial cells (astrocytes) are key for synaptogenesis o In vitro studies: Neurons cultured with astrocytes form 7 times as many synapses as those without astrocytes. o In vivo studies: Studies with KO mice similarly demonstrate the importance for synapse-promoting and inhibiting signals secreted by astrocytes 5. Neuron Death and Synapse Rearrangement There is an overproduction of neurons during proliferation – about 50% more than actually Functions of needed. The “surplus” neurons die. Neuronal 1. Neurons that make incorrect Cell Death connections die o Developing neurons are very promiscuous o Make lots of connections; not all are essential 2. New neurons make more focused synapses o Cell death increases the overall accuracy of synaptic connections Processes of Cell Death 1. Apoptosis o Active cell death o A “clean” process 2. Necrosis o Passive cell death o A “dirty” process What causes neurons to die? 1. Genetically programmed (some neurons) 2. Competition for target-supplied neurotrophins o Nerve growth factor: the first neurotrophin to be isolated (Levi- Montalcini,1952). Neurotrophins Nerve growth factor (NGF) Brain derived neurotrophic factor (BDNF) Glial cell-line derived neurotrophic factor (GDNF) Neurotrophin 3 (NT-3) Neurotrophin 4/5 (NT-4/5) Ciliary neurotrophic factor (CNTF) Fibroblast growth factor (bFGF) 1. They promote neuronal growth Functions of 2. They promote neuronal survival Neurotrophins 3. They can function as axon guidance molecules 4. They can stimulate synaptogenesis 5. Their absence can trigger apoptosis 1. Uthman, E. (1999). 9-Week Human Embryo from Ectopic Pregnancy. Wikimedia Commons. https://upload.wikimedia.org/wikipedia/commons/5/53/9- Week_Human_Embryo_from_Ectopic_Pregnancy.jpg 2. Parrot, P. (1996). Jeanne Calment (1875-1997). In: Holdsworth, M. (2008). Dialogues Cardiovasc. Sci., 13: 190. 3. Wiley family (1950s). Irene Wiley with her two children… ABC News. https://web.archive.org/web/20140924173405/http://abcnews.go.com/US/popup?id=4870528&page=4 Image 4. Garmon, L. (Writer, Director) (1994, Oct 18). The Secret of the Wild Child (S21, E12) [TV Series episode]. In P. Apsell (Executive Producer), NOVA. Public Broadcasting Service. https://en.wikipedia.org/wiki/File:Genie_(feral_child).jpg References 5. Zeiss Microscopy (2014). Confocal microscopy of Drosophila brain and neuronal structures with ZEISS LSM 780. Flickr. https://www.flickr.com/photos/zeissmicro/12190929525 6. Zeiss Microscopy (2014). Zebrafish embryo, multiview light-sheet fluorescence microscopy. Flickr. https://www.flickr.com/photos/zeissmicro/12206703494 7. Metscher, B. (2011). Chick embryo immunostained for acetylated α-tubulin, contrasted with peroxidase- mediated reduced silver deposition. In: Metscher & Müller (2011). Dev. Dyn., 240: 2303. https://doi.org/10.1002/dvdy.22733 8. Gómez, M. B. et al. (2019). What Is the Purpose of Gastrulation in Embryonic Development? InviTRA. https://www.invitra.com/en/gastrulation/ 9. Gray, H. (1918). Anatomy of the Human Body, 20th edition. Lea & Febriger: Philadelphia/New York City. 10. Wells, T. et al. (2011). Front. Mol. Neur., 4, https://doi.org/10.3389/fnmol.2011.00006 11. Nadarajah, B. & Parnavelas, J. G. (2002. Nature Rev. Neur., 3: 425-6. https://doi.org/10.1038/nrn845 12. Pérez, V. et al. (2013). BMC Vet. Res., 9: 156. http://dx.doi.org/10.1186/1746-6148-9-156 13. Allen, N. J. and Lyons, D. A. (2018). Science, 362: 182. https://doi.org/10.1126/science.aat0473 14. Guerin et al. (2006). Int. J. Dev. Biol., 50: 669. https://doi.org/10.1387/ijdb.062159mg