Ch 14 part 1 apk.docx
Document Details
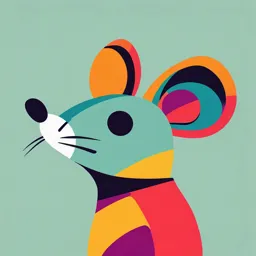
Uploaded by AppreciatedTurkey
Full Transcript
Ch 14 - Blood Vessels and Pressure- Part 1 Physical Laws Governing Blood Flow and Blood Pressure In this section, we will examine how the body gets blood to the tissues that need it. At any given time, certain tissues may need more blood flow than others. The body has a mechanism to redirect blood f...
Ch 14 - Blood Vessels and Pressure- Part 1 Physical Laws Governing Blood Flow and Blood Pressure In this section, we will examine how the body gets blood to the tissues that need it. At any given time, certain tissues may need more blood flow than others. The body has a mechanism to redirect blood from tissues that do not require much blood to tissues that need a lot of it. The body also has built-in mechanisms to adjust to potentially dangerous patterns in blood flow. For example, suppose that you're suffering from of lack of blood flow to the brain. Without a constant supply of oxygen, you will faint. When you faint, you fall over. In a person who is standing up, the heart has to pump blood against gravity to get it to the brain. Once you have fallen over, the heart no longer has to overcome the force of gravity. This makes it easier for blood to get to your brain. This part of the course will require a small amount of physics. At its most basic level, blood flow through blood vessels follows the physical laws describing the blood flow of any liquid through any system of pipes. The flow rate of a Iiquid through these pipes is directly proportional to the difference between the pressures of the two ends of the pipe and inversely proportional to the resistance of the pipe. In layman's terms, blood flow is higher when the pressure difference between the two ends of the tube is higher and when the resistance to blood flow Is lower. This can be expressed mathematically as: Flow = (P1 – P2)/Resistance = size of the pressure gradient divided by the resistance. Based on this equation, the body can increase blood flow in two ways: By Increasing the pressure gradient. By decreasing the resistance. Likewise, the body can decrease blood flow in two ways: By decreasing the pressure gradient. By increasing the resistance. Next, we will separately discuss pressure gradients and resistance in the cardiovascular system. We will then discuss ways that the body can control blood flow. Pressure Gradients in the Cardiovascular System The blood in the vasculature is always moving, so we can infer that there is a pressure gradient moving it from areas of high pressure to areas of low pressure. Liquid moves down a pressure gradient whenever there is a difference in pressure between two locations. Bulk flow is a term that refers to the movement of any substance (including air, liquid, or gas} down its pressure gradient. For example, when you blow up a balloon, you create an area of high pressure. If you open the balloon, air will flow out of the balloon. This is an example of bulk flow. The heart is the primary organ that produces pressure in the vasculature. Mean arterial pressure is greatest in the arteries. It decreases as it flows through the vasculature particularly at the level of the arterioles. It is lowest in the veins as it returns to the heart. The pressure gradient between the arteries and the veins provides the force that drives the movement of blood through the vasculature. Hydrostatic columns Pressure concepts can be conceptualized with a hydrostatic column. Specifically, a hydrostatic column of water demonstrates the concept of hydrostatic pressure, which is the pressure exerted on a fluid due to the force of gravity. Consider two tubes of water with a linking “vessel" between them. If one Jar has more water in it (and, thus, higher hydrostatic pressure), the pressure gradient Is determined by the vertical difference between the tops of the two columns of liquid. The liquid will tend to flow down the pressure gradient from the column with more hydrostatic pressure to the column with less hydrostatic pressure. Eventually, the two columns will equalize, and the net flow of water will be zero. Note that the rate of flow does not depend on the absolute pressure In either column: it depends on the pressure difference between the two columns. In this set of hydrostatic columns, the amount of flow Is the same in both of these sets of columns, even though the absolute pressure Is greater in the set of columns on the left. The pressure Difference, or the pressure gradient, is the same in both columns, so the rate of flow is the same. In a hydrostatic column like the ones illustrated above, the flow of water from one column to another eventually eliminates the pressure gradient. As a result, water flow eventually stops as the system reaches equilibrium. This never happens in our bodies, however, because the heart continuously pumps blood into the arteries and pressure continuously decreases as the blood moves through the vasculature. The pressure gradient for systemic blood flow (P1 – P2) is equal to the mean arterial pressure (MAP) minus the central venous pressure (CVP). That is: The pressure gradient = (p1-p2)= MAP – CVP As we have discussed, mean arterial pressure (MAP) is a weighted average of the end diastolic pressure and the end systolic pressure. It is closer to the end diastolic pressure than to the end systolic pressure because the heart spends more time in diastole (at rest) than in systole (pumping). The central venous pressure (CVP) describes the blood pressure in the vena cava, as it approaches the right atrium of the heart. Central venous pressure is normally very low (about 2 to 3 mm Hg), so we will ignore it for the purposes of this class. If we ignore central venous pressure and assume it is 0, we can conclude that the pressure gradient for systemic blood flow is entirely determined by mean arterial pressure. That is, The pressure gradient equals the Mean arterial pressure. Mean arterial pressure is approximately 85 mm Hg. This is the driving force for blood reaching all of the organs in the body, so it is sometimes called organ perfusion pressure. Recall that the body increases blood flow by increasing the pressure gradient and decreases blood flow by decreasing the pressure gradient. This implies that blood flow increases when mean arterial pressure increases and that blood flow decreases when mean arterial pressure decreases. the pressure gradient is much higher in the systemic circuit than in the pulmonary circuit. This is not a problem, however, because blood flowing through the pulmonary circuit only has to travel a short distance, and the resistance to flow Is much smaller in the pulmonary circuit than In the systemic circuit. As we have noted, blood pressure decreases as blood flows from the arteries, to the arterioles, to the capillaries, to the venules, and finally to the veins. The most significant drop in blood pressure occurs at the level of the arterioles, which have a lot of smooth muscle and exhibit significant resistance to flow. This is a "built-in safety mechanism” that protects the capillaries from "blowing out." Recall that capillaries consist of a single layer of simple squamous epithelium and a small amount of basement membrane, with no smooth muscle at all. If the pressure of blood flowing through the capillaries was too high, the capillary walls would burst. Again, remember the following relationship: Flow = (P1 – P2)/Resistance = The pressure gradient/Resistance The pressure gradient is larger in the systemic circuit than in the pulmonary circuit, but the amount of blood flow is the same in both. We accommodate for the higher pressure gradient in the systemic circuit with a greater amount of resistance-primarily, at the arterioles. Resistance in the Cardiovascular System Recall that blood flow is inversely related to the resistance of the vessels it is flowing through. Resistance of individual blood vessels depends both on their physical dimensions and the properties of the blood flowing through them. Three measurements affecting resistance are as follows: 1 Vessel radius - The radius of a vessel can be altered through vasoconstriction (which increases resistance) or vasodilation (which decreases resistance). Resistance increases when the vessel's radius decreases, and it decreases when the vessel's radius increases. This is the most important factor that determines a vessel's resistance to blood flow. EXAM TIP: Vessel radius is, by far, the most important factor affecting resistance in the cardiovascular system. To illustrate the impact at vessel radius on blood flow, the professor asked students to think about sucking a milkshake through a straw. If the straw is skinny, you'll have to suck really hard because there's a lot of resistance. If the straw is wider, you won't have to suck as hard. 2 Vessel length - Longer vessels have greater resistance because they provide more surface with which the blood can come into contact. This is rarely a factor in the body, however, because vessel length doesn't change much except for instances of rapid growth, such as rapid weight gain. We will largely ignore the impact of vessel length on resistance. 3 Blood viscosity - You probably intuitively know that it's easier to drink a soda than a milkshake through a straw; this is because milkshakes are thicker (more viscous) than sodas. Likewise, thicker blood has more resistance because it does not move as easily through the vasculature. The viscosity of blood Is mostly determined by the concentration of red blood cells and proteins relative to water in the blood. People who take blood thinners do so to reduce the viscosity of the blood, which reduces resistance and thereby reduces blood pressure. These three factors affecting resistance are summarized by a mathematical equation known as Poiseuille's Law. This equation is as follows: Resistance equals (8 * L * Pi) divided by (Pi * radius to the 4th power) In this equation, R is the resistance, L is the tube length, Pi is the viscosity, and r is the internal radius of the tube. The numbers 8 and Pi are constants. Note that vessel length and viscosity are directly related to resistance (and thus inversely related to blood flow), while the radius of the vessel is inversely related to resistance (and thus directly related to blood flow). This law makes it clear that resistance is most impacted by the radius of the vessel. Note that the radius is taken to the fourth power in the equation above. This means that if the radius of the vessel is decreased by half, resistance increases 2 to the 4th power, or 16-fold! Even small changes in the radius of the vessel produce large changes in resistance. Thus far, we have only considered the resistance in a single vessel. In reality, however, blood flows through a network of thousands of vessels as it makes its way from the heart through the systemic circuit. Total peripheral resistance (TPR), or the resistance of the entire vascular network, is determined by combining the resistance of the individual vessels in it. (Note that TPR is also referred to as total vascular resistance, or TVR.) The cardiovascular system is all interconnected, so on increased resistance in any one vessel will increase the overall resistance for the entire system. The implications are as follows: Vasoconstriction of any blood vessel increases the resistance in that vessel and thus increases total peripheral resistance. Vasodilation of any blood vessel decreases the resistance in that vessel and thus decreases total peripheral resistance. Note that total peripheral resistance will not change when offsetting changes in resistance occur. For example, if peripheral resistance in one area increases but peripheral resistance in another area decreases, total peripheral resistance does not change. This is how the body controls blood flow to the various organs. Peripheral resistance might decrease in the area of an organ that really needs blood flow (for example, through vasodilation). At the same time, peripheral resistance might increase in the area of an organ that does not need blood flow (for example, through vasoconstriction). Putting It All Together: Determining Cardiac Output Recall that the general equation for the flow of a liquid through a tube is as follows: Flow = the pressure gradient/resistance In the cardiovascular system, the relevant flow coming out of the heart is the cardiac output (CO), the difference in pressure is determined by the mean arterial pressure (MAP), and the resistance is equal to the total peripheral resistance (TPR). This relationship is described as follows: Cardiac Output = mean arterial pressure / total peripheral resistance Review of the Vasculature Recall that blood flows from arteries, to arterioles, to capillaries, to venules, and to veins. Arteries have thicker walls because they have a thicker layer of smooth muscle cells. Veins have valves, which promote the unidirectional flow of low-pressure blood bock to the heart. Arterioles are the areas of most resistance; they reduce blood pressure and blood velocity, "slowing things down" to protect the capillaries and to provide enough time for gas exchange to occur. Arteries as Pressure Reservoirs Arteries serve as a pressure reservoir. They are able to store pressure due to their elastic walls, which are composed of smooth muscle tissue and the connective tissue elastin. They ore stiff enough to serve as supports but flexible enough to expand and store pressure. Blood vessels expand when the pressure inside them increases, and they contract when the pressure inside them falls. The degree of expansion or contraction depends on the pressure difference between the inside and outside of the vessel, which is known as distending pressure (or transmural pressure). Compliance is a measure of the ease with which a vessel can expand or contract. Compliance is measured as a change in volume over change in distending pressure: Compliance = change in volume/change in distending pressure For example, a balloon with high compliance will expand easily, whereas a balloon with low compliance will be much harder to blow up. If you try to make it easier to blow a balloon up by stretching it out with your hands before blowing into it, you're trying to make the balloon more compliant. (You might contrast the rubbery balloons, which are sometimes not compliant, with foil-looking balloons, like the kinds you might see already blown up at grocery stores. These balloons hove no elastic recoil, so they are highly compliant and will increase in volume easily when you blow into them.) We could graphically demonstrate the relationship between the volume of blood in a vessel and distending pressure in a diagram such as the one to the right. Note thot blood volume increases as transmural pressure increases. The slope of the line between two points on this curve (such as A and ) represents the compliance of the vessel (the change in volume divided by the change in distending pressure). A steeper slope between the 2 points means greater compliance, which indicates that the vessel expands easily (i.e., the change in volume is high) due to a given change in distending pressure. Arteries hove low compliance, which means that they recoil when they are stretched out. Low compliance means that the change in volume divided by the change in distending pressure is low. This means that small changes in volume result in significant changes in pressure and that large changes in pressure result in small changes in volume. This is what allows them to be effective pressure reservoirs.