Isolation, Nutrition, and Cultivation of Microorganisms PDF
Document Details
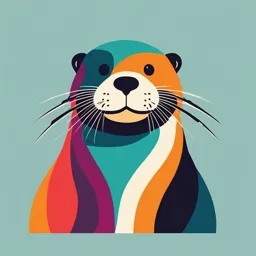
Uploaded by TantalizingOpal
Háskólinn á Akureyri
Tags
Summary
This chapter delves into the isolation, nutrition, and cultivation of microorganisms. It explores the diverse metabolic strategies employed by these organisms, highlighting their ability to utilize various carbon sources. It emphasizes the evolutionary underpinnings of microbial diversity and the importance of microorganisms for maintaining atmospheric CO2 levels.
Full Transcript
Chapter 5 It appears incongruous to delineate metabolism of bacteria from that of fungi, since the two overlap in so many respects and since each group displays, more than any other groups of microorganisms, a kaleidoscopic array of metabolic diversity. – JACKSON W. FOSTER reen plants are photosynt...
Chapter 5 It appears incongruous to delineate metabolism of bacteria from that of fungi, since the two overlap in so many respects and since each group displays, more than any other groups of microorganisms, a kaleidoscopic array of metabolic diversity. – JACKSON W. FOSTER reen plants are photosynthetic, gaining their energy from sunlight and using CO2 as their carbon source. Animals, on the other hand, require preformed organic molecules for both energy and building material. These preformed molecules may be microbial, plant or animal in origin. A plant or an animal species is generally differentiated from any other plant or animal species by observable differences in size, shape, and component structures. Mites to elephants or moss to sequoias are examples of the extremes in size and structure in the animal and plant world. In contrast to plants and animals, the various prokaryotic microorganisms are not markedly different from one another either in size or structure. Microorganisms are generally differentiated from one another by their nutrition, which is the sum of the processes whereby the organism takes in and utilizes food for energy and building material (carbon). Among Bacteria and Archaea, nutritional needs vary from species to species. It is axiomatic that a microorganism in nature can utilize, as nutrient source, any carbon-containing constituent that is or has been a component part of living cells. Many other compounds unrelated to living cells can also be utilized as substrate (source of food) for growth. Among these compounds that microorganisms can actually utilize as carbon/energy sources are carbon monoxide, methane, cyanide, acetic acid, or glucose. Others readily utilize aromatic compounds, such as benzene, that are toxic to most eukaryotes. These marked nutritional differences are referred to collectively as representing “metabolic diversity in the microbial world.” This chapter examines the major nutritional differences that distinguish microorganisms, how different nutritional types can be isolated, and how they may be cultivated and preserved. FUNDAMENTAL ASPECTS OF DIVERSITY Microorganisms live in virtually every environmental niche on Earth. These microbial populations can utilize the naturally occurring organic compounds that fall into these niches as sources of carbon and/or energy. Many industrial chemical synthesis products, such as pesticides, are also metabolized by natural microbial populations. However, it should be noted that some chemically derived compounds are resistant to microbial degradation, and these tend to accumulate in the environment. Evolution has resulted in a seemingly endless array of microbial types with broad metabolic capacities. These organisms differ from one another in their nutritional capabilities and their adaptation to the physical conditions in which they live. Selected Bacteria and Archaea are adapted to 104 CHAPTER 5 growth under wide-ranging physical conditions, including pH (<1 to >11), temperature (<0°C to >100°C), salinity (freshwater to saturated saltwater), or availability of O2 (aerobic to anaerobic). These physical conditions are further imposed on the nature and availability of substrates for growth of a microorganism (form of carbon, nitrogen, or phosphorous). Most bacterial species utilize simple monomeric substrates such as sugars or amino acids as growth substrate, whereas others digest complex proteins and polysaccharides. Although some bacteria, such as Pseudomonas cepacia, can utilize any one of over 100 different carbon-containing compounds for growth, most organisms can utilize only a limited number. Other microbes are restricted to growth on only one or a few chemically related substrates. For example, Methylomonas methanooxidans will grow only when methane or methanol is provided as growth substrate. Collectively, however, microbial populations are capable of growth on the unending variety of substrates encountered in nature. This broad metabolic diversity is essential in maintaining the level of CO2 in the atmosphere. This CO2 is the carbon source for the photosynthetic populations that are the basis for all viable systems on this planet. The Evolution of Metabolic Diversity The presence of diverse nutritional types, as the quote at the beginning of the chapter affirms, is a consequence of the evolutionary role that microbes have assumed. Much of the microbial community has evolved with the ability to mineralize constituents or end products of all biota. The word mineralize is used in this text to emphasize that microorganisms can break down organic molecules to basic constituents (NH4, CO2, SO4–, PO4–, etc.). In the course of microbial evolution, processes of mutation and selection gave rise to populations that are able to utilize all available carbon compounds as their source of carbon and/or energy source. Populations of microbes have also evolved that can obtain energy from light and from inorganic substrates: hydrogen (H2), sulfides, reduced iron, and ammonia. Other organisms evolved that could utilize gaseous carbon sources, such as CO, Milestones Box 5.1 CO2, methane, ethane, or propane. The ability to “fix” atmospheric nitrogen (N2) lent a distinct advantage to selected Bacteria and Archaea occupying environments where little combined nitrogen (NH4+, NO3–, or organic) would be available. The ability to utilize a substrate better than others, or a substrate under physical conditions that others could not, gave a survival advantage. It also gave rise to a broad array of microbial species with small but significant differences in their nutrient requirements and tolerance to physical conditions. Basically, survival advantage has been the key during the four billion years of evolution and a major factor in generating microbial diversity (Box 5.1). Nutritional Types of Bacteria Bacteria can be divided into four major groups based on their source of carbon and their source of energy (the nutrition and characteristics of the Archaea is discussed in Chapter 18). All microorganisms must have a source of carbon to provide building blocks, and they require a source of energy to drive the reactions involved in the synthesis of cell components. The group distinctions are based on the source of carbon and the source of energy, that is, the use of CO2 versus organic compounds as carbon source and light or chemicals as energy source. Before discussing the characteristics of the individual groups, some features of bacterial metabolism should be considered. The ratio of carbon/hydrogen/oxygen in a cell is 1-2-1, respectively, and an organism growing with CO2 as carbon source would need a source of H+ to reduce CO2to CH2O. This source of H+ is called the electron donor; for example, H2S, NH4+, H2O can all serve as a source of hydrogen (electron donor), as the electron would carry along the proton. An organism that utilizes reduced substrates such as methane (CH4) as carbon source must contain an electron acceptor (O2) to oxidize the substrate to the CH2O level. Most microorganisms (and animals) utilize substrates such as glucose (C6H12O6) that have a C/H/O ratio equivalent to that in a cell. Although the categories presented here are not all inclusive, they do provide a framework for separating The Unique Character of Bacteria and Archaea Bacterial and archaeal species possess a significant number of unique capabilities not found anywhere among the eukaryotes.They: • Fix atmospheric nitrogen (N2) • Synthesize vitamin B12 • Use inorganic energy sources NH4, H2S, H2, Fe2+ • Photosynthesize without chlorophyll • Utilize inorganic (CO2, NO3–, SO42–, So, Fe3+) terminal electron acceptor as an alternative to O2 • Have extensive capacity for anaerobic growth • Use H2S, H2, or organics as electron donor in photosynthesis • Grow at temperatures in excess of 80°C ISOLATION, NUTRITION, AND CULTIVATION OF MICROORGANISMS microbes into reasonable and concise nutritional units. These groups are: • Photoautotroph (from photo meaning “light,” auto meaning “self,” and troph meaning “feeding”): Photoautotrophs use light as their source of energy and CO2 as their source of carbon. An obligate photoautotroph is an organism that will grow only in the presence of both light and CO2. Obligate photoautotrophs use inorganics such as H2O, H2, or H2S as electron donor to reduce CO2 to cellular carbon (CH2O). • Photoheterotroph (from hetero meaning “different”): Photoheterotrophs grow by photosynthesis if provided with an electron donor (H2 or organic) for reductive assimilation of CO2. Commonly, organisms in this group require selected growth factors, such as B-vitamins, and several species will grow on organic substrates if oxygen is available. They may also utilize light as an energy source while assimilating organic compounds from the environment as growth substrate. • Chemoautotroph (from chemo meaning “chemical”): Chemoautotrophs utilize reduced inorganic substrates for both the reductive assimilation of CO2 and as a source of energy. The oxidation of H2, NH3, NO2–, H2S, or Fe2+ supplies energy for these microorganisms. Aerobic chemoautotrophs utilize O2 as terminal electron acceptor, and some of the anaerobic Archaea can utilize inorganic sulfur as terminal electron acceptor (see Chapter 18). • Chemoheterotroph: Chemoheterotrophs are microorganisms that assimilate organic substrates as source of both carbon and energy. This may be a single substrate such as glucose or succinate, or the sources of carbon and energy may be different. For example, the sulfate reducers use H2 for energy but require an organic substrate for cellular biosynthesis. The vast majority of the microbes that have been studied are chemoheterotrophs. This brief outline affirms that the various bacterial and archaeal species may have significant differences in their nutritional requirements. Bacteria representing each of these categories are presented in Figure 5.1. The ability of some organisms to fit into more than one of the preceding groupings is discussed in Box 5.2. Under laboratory conditions, microorganisms grow in an artificial environment generally not equivalent to that encountered in nature. A microorganism living in soil, water, or the intestinal tract of a warm-blooded animal survives there because its nutritional requirements are met. These requirements may be simple or they may be complex. If we wish to take a microorganism from an environmental niche and grow it in the laboratory, we must somehow mimic the conditions that permitted it to survive in the environment. These would include both physical conditions (pH, temperature, osmotic pressure, light) and substrate availability. A laboratory medium must provide nutrients that are essential for growth. The following section examines in some detail how to prepare a growth medium. Both of these phototrophic organisms were grown using light as their energy source, but with different carbon sources . . . . . . the photoautotroph used CO2 . (A) These chemotrophic organisms were grown using different energy and carbon sources . . . . . . the chemoheterotroph used glucose as both energy and carbon source. . . . the chemoautotroph used sulfide, S2–, as energy source and CO2 as carbon source. . . . the photoheterotroph used acetate. (B) 105 (C) Figure 5.1 Nutritional types Colonies of microorganisms representing the major nutritional groups: (A) Anabaena viriabilis, a photoautotroph; (B) Rhodomicrobium sp., a photoheterotroph; (C) Thiobacillus perometabolis, a chemoautotroph; and (D) Micrococcus luteus, a chemoheterotroph. A, courtesy of Robert Tabita; and B–D, courtesy of Jerome Perry. (D)