Central Nervous System Physiology Primer PDF
Document Details
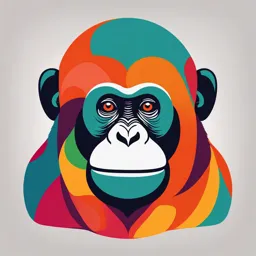
Uploaded by AccomplishedMagic
Stony Brook University
Kelly A. Warren, Ph.D.
Tags
Summary
This document is a primer on CNS physiology, covering the development and organization of the nervous system, the function of cerebrospinal fluid and the blood-brain barrier, and the structure and function of CNS structures. It includes detailed information on the different types of cells in the nervous system, and describes the structure and function of the brain and spinal cord.
Full Transcript
Kelly A. Warren, Ph.D. Department of Physiology and Biophysics BST-T5, Rm. 180 [email protected] CNS Physiology I and II The lecture material is provided in both slides and notes format. The material covered in these lectures can also be referenced in Chapter 9 and 13 of Silverthorn’s Hum...
Kelly A. Warren, Ph.D. Department of Physiology and Biophysics BST-T5, Rm. 180 [email protected] CNS Physiology I and II The lecture material is provided in both slides and notes format. The material covered in these lectures can also be referenced in Chapter 9 and 13 of Silverthorn’s Human Physiology (5th Edition) textbook. Lecture Objectives: 1. 2. 3. 4. Understand the development and organization of the nervous system Describe nervous system cell types and function Understand the function of the cerebrospinal fluid and blood brain barrier Describe the structure and function of CNS structures, from the spinal cord working up towards the cerebral cortex 5. Understand the basic complex functions of the CNS 6. Discuss motor control pathways and execution of gross and/or complex motor movements ORGANIZATION OF THE HUMAN NERVOUS SYSTEM The central nervous system (CNS) is just one component in understanding overall nervous system function. The CNS comprises the brain and the spinal cord and acts in the analysis and integration of sensory and motor information. However, the CNS has extensive communication with sensory systems (which allow for the sensation of both internal and external environment) and motor systems (which act to drive visceral and somatic motor function). These latter two systems are considered the peripheral nervous system (PNS). It is the coordinated actions of both the CNS and PNS together that allows for effective functioning. EVOLUTION OF THE NERVOUS SYSTEM The nervous system provides an organism with the ability to respond to changes in its internal and external environments. The examples below provide insight into an array of neural networks, including those where integrating centers are absent, primitive, or extensive and developed. 2 NERVOUS SYSTEM DEVELOPMENT The initial stages of nervous system development are dependent on a programmed series of mitotic events that initially transition a single-cell zygote into a multicellular structure (the blastula). The structure undergoes a critical process called gastrulation, where the embryo creates a local invagination of a subset of cells. This invagination results in three distinct primitive cell layers including (1) the endoderm, (2) the mesoderm, and (3) the ectoderm. The formation of these layers is crucial in that these layers contain cells that are predetermined to form into specific structures. For example, the endoderm layer will ultimately form the epithelial lining of the GI tract, along with the glands that open up to the lumen of the GI tract. This layer will also lead to the formation of lung, thyroid, and urethral structures. 3 The mesoderm layer will develop into the skeletal system, including both muscle and bone structures, the dermis of the skin, connective tissue, heart, blood, and spleen. The ectoderm layer will form the tissue that covers the entire body surface and also lead to the formation of the central nervous system (crucial in these lectures), cranial and sensory nerves, peripheral ganglia, pigment cells, hair, and lens of the eye. Neurulation is the next critical process in establishing the brain and spinal cord structures in a mammalian embryo. Neurulation involves the folding of the neural plate (comprised of mesoderm) into a neural groove, and ultimately neural tube. Generally by the 23rd day of human development, the overall process of neurulation is complete. This neural tube gives rise to the brain and spinal cord. Three sets of cells are important to the formation of this embryonic structure. (1) Neural precursor cells – dividing neural stem cells that produce precursors with the capacity to give rise to neurons, astrocytes, and oligodendrocytes (CNS cells) (2) Neuroblasts – non-dividing cells that differentiate into neurons (3) Neural crest cells – cells which have migrated away from the neural tube and that will ultimately give rise to a variety of cell types of the PNS, including neurons and glia of the visceral and sensory systems, neurosecretory cells of the adrenal gland, and neurons of the enteric nervous system Later in development (4 weeks), the neural tube will be specialized into three major brain regions including, the forebrain, midbrain, and hindbrain. Within a few weeks these three regions will further subdivide and differentiate. The forebrain will differentiate into the diencephalon and 4 cerebrum. The hindbrain will differentiate into the medulla, pons, and cerebellum. Further along in development, the cerebrum structure of the forebrain will become noticeably enlarged, ultimately leading to the formation grooves in the cerebral surface. The embryonic brain, through each successive subdivision, will be transformed into the adult brain structure. The table below identifies the adult brain derivatives that are the product of the early embryonic brain structures. 5 CELLS OF THE NERVOUS SYSTEM It should be quite apparent that the structures and function of the CNS and PNS cannot exist without distinct cell types acting in a coordinated fashion. Please note, these distinct cell types and structure terminology differ when considering the CNS and PNS. The major functional unit of the nervous system is the neuron. Neurons are excitable cells that receive, integrate, and output information. They come in different forms, including pseudounipolar, bipolar, and multipolar and can be classified as sensory neurons, interneurons, and motor neurons. As you have already learned, neurons consist of a cell body (or soma), axon, and dendritic terminals. The cell bodies of neurons within the CNS are referred to as nuclei, whereas they are referred to as ganglia within the PNS. The axons of neurons within the CNS are referred to as tracts, whereas they are called nerves in the PNS. The number of inputs that a particular neuron may receive will depend completely on its dendritic arborization (number and branching of dendrites). Neurons lacking a large number of dendrites receive information from only a few other cells, while neurons that have quite an abundance of dendrites may be innervated by an extremely large number of neurons. This brings to light two ideas you must become familiar with: convergence and divergence. Convergence – the number of inputs to a single neuron Divergence – the number of targets innervated by one neuron White Matter vs. Gray Matter - The tissues of the CNS are divided into gray and white matter. Gray matter consists of unmyelinated nerve cell bodies, dendrites, and axon terminals. White matter consists of myelinated axons. Glial cells are another cell type of the nervous system. Glial cells outnumber neurons, but do not participate directly in synaptic interactions and electrical signaling. Instead, they perform supportive functions which help define synaptic contacts and maintain the signaling abilities of neurons, while also maintaining an adequate extracellular environment for normal neuronal function. There are three types of glial cells. 6 1. Astrocytes are restricted to the CNS and appear “star-like” in shape. These cells aim to maintain the appropriate chemical environment for neuronal signaling, generally by uptaking into their intracellular space excessive potassium and neurotransmitters that are free in the extracellular and synaptic environment. They have the ability to recycle neurotransmitters and their byproducts of enzyme degradation back to neurons to replenish the supply. 2. Myelinating glia refers to either oligodendrocytes (CNS) or Schwann cells (PNS). These cells wrap myelin around axons of neurons in order to increase the effectiveness and velocity of the transmission of electrical signals. 3. Microglial cells share similar properties to that of macrophages, in that they act as scavengers that remove cellular debris from areas of damaged neuronal tissue. Microglia have also been implicated in immune response pathways, as they also have the ability to secrete signaling molecules (e.g., cytokines) that influence inflammation and mediate a cell’s survival and death. Although not considered a classic glial cell, ependymal cells exist within the CNS and act as a lining between compartments. They are found lining the ventricles, and in some regions of the CNS, are capable of secreting cerebrospinal fluid. 7 BONE AND CONNECTIVE TISSUE SUPPORT The brain and spinal cord are protected from injury by bone and connective tissue support structures. The brain is encased in the skull (or cranium), while the spinal cord is protected by the vertebral column. Connective tissue layers exist between the bone of the cranium/vertebrae and brain/spinal cord to provide additional structural support. These are collectively referred to as the meninges and are composed of three layers. (1) Dura matter is the layer closest to the bone and is composed of tough and fibrous tissue (2) Arachnoid matter is the middle layer and forms a web-like structure (3) Pia matter is a thin membrane that adheres closest to the surface of the brain and spinal cord and contains small arteries that supply the brain with blood The space that exists between the dura matter and the arachnoid membrane is referred to as the subdural space. The space that exists between the arachnoid and pia matter is referred to as the subarachnoid space. CEREBROSPINAL FLUID AND THE VENTRICULAR SYSTEM The brain and spinal cord are further protected from injury through the shock absorption properties of the extracellular fluid environment surrounding them. The extracellular fluid is made up of the blood, interstitial fluid, and cerebrospinal fluid within the brain and spinal cord regions. Where interstitial fluid is located within the pia matter, cerebrospinal fluid is compartmentalized into the cavities of the ventricular system. Cerebrospinal fluid (CSF) is a salty solution that is secreted by specialized ependymal cells within the choroid plexus of the third ventricle. These cells pump sodium and other solutes from the plasma of blood into the ventricles. Due to the solute gradient that exists, water will follow the solute by osmosis into the ventricular space as well. CSF has two major functions within the CNS, to provide physical and chemical protection. 8 Physical protection: Reduction in the weight of the brain through buoyancy, protective padding for head injury Chemical protection: CSF is a different composition than plasma and the choroid plexus epithelial cells are quite selective about which ions and solute can pass. This leads to a solution that has values of potassium, calcium, bicarbonate, and glucose that are lower than observed in plasma. Proton concentrations, however, are typically higher within the CSF. Additionally, the CSF very rarely contains protein and/or blood cells. With regards to chemical protection, the CSF also allows for waste removal from the brain and spinal cord environment through exchange with the interstitial fluid. CSF flows throughout the brain, from the ventricles into the subarachnoid space where it cushions the CNS. In this way, it can surround the entire brain and spinal cord in fluid. Eventually, specialized structures that exist on the arachnoid membrane, called villi, absorb some of the CSF back into the blood, requiring this circulating volume to be replaced continuously (~3 times/day). BLOOD BRAIN BARRIER The capillaries within the brain create a tight meshwork that constitutes the blood-brainbarrier (BBB). This BBB is highly selective to incoming solutes such as ions, hormones, and neuroactive substances, leading to reductions in permeability. Reduced permeability is a basic consequence of these capillaries exhibiting tight junctions that prevent the movement of solutes between cells, thus distinguishing them from capillaries elsewhere in the body. The selective permeability is also a consequence of the transporters that 9 exist within/on these capillaries that allow for the selective movement of nutrients and other useful materials from the blood into the interstitial fluid. Movement also exists in the opposite direction, where the blood becomes the repository for metabolic wastes being excreted from interstitial fluid. The BBB allows for the non-carrier mediated transport of small lipid-soluble molecules, but does not allow for transport of water-soluble substances. This becomes critical when considering drug development and efficacy at CNS sites. THE SPINAL CORD The spinal cord transmits sensory and motor content between the brain and the periphery and consists of four distinct regions including (1) cervical, (2) thoracic, (3) lumbar, and (4) sacral spinal cord. Each of these distinct regions give rise to bilateral spinal nerves. These nerves branch and carry sensory and motor information towards and away from the spinal cord, respectively. Sensory information is carried by sensory afferent neurons whose cell bodies reside in the dorsal root ganglia. From these ganglia, these axons synapse onto interneurons within the dorsal horn gray matter in distinct regions (nuclei) for visceral and somatic information. Motor information is relayed from cell bodies of the gray matter within the ventral horn of the spinal cord. These cell bodies comprise nuclei that relay autonomic motor or somatic motor function, carrying efferent signals to the muscles, organs, and glands. 10 . Additionally, within the spinal cord, axons are segregated by the direction in which they travel. Ascending tracts of white matter (myelinated axons) occupy the dorsal and lateral limits of the spinal cord and transmit sensory information to the brain. Descending tracts of white matter occupy the ventral and interior lateral portions of the spinal cord and relay motor commands from the brain to effectors. The spinal cord can function on its own as well, and serves as an integrating center for simple spinal reflexes as you learned previously. As a reminder, a spinal reflex initiates a response without input from the brain. See figure on following page for reference. 11 THE BRAINSTEM The brainstem is the transition between the brain and the spinal cord and consists of the midbrain (colliculi), pons, and medulla oblongata. 12 The brainstem is the target or source for the cranial nerves that deal with sensory and motor function in the head and neck. It also provides a through-way for all of the ascending sensory tracts from the spinal cord; the sensory tracts for the head and neck (trigeminal system); the descending motor tracts from the forebrain; and the local pathways that link eye movement centers. It is important to recognize that 10 of the 12 cranial nerves originate in the brainstem (olfactory and optic nerves are the exception) and that they consist of different types of fibers including sensory, motor, or a mixture of both. Refer to the table below for more detail. Cranial nerve nuclei, which are either the target or source of the cranial nerves, exist within the brainstem region. With the exception of the cranial nerve nuclei associated with the trigeminal nerve, there is a fairly close correspondence between the location of the cranial nerve nuclei within the midbrain, pons, and medulla and the location of the associated cranial nerves. 13 The medulla is a region of the brainstem that sits directly above the spinal cord and contains both gray and white matter. White matter includes ascending somatosensory tracts that bring sensory information to the brain and descending corticospinal tracts that convey motor information from the cerebrum to spinal cord. Gray matter within the medulla makes up brainstem nuclei that are responsible for involuntary functions including swallowing, vomiting, and cardiorespiratory activity. The pons sits above the medullary region and below the midbrain and serves as a relay station for information being transferred between the cerebellum and brain (through peduncle tracts). It also has an impact on neural cardiorespiratory control in collaboration with the medullary region. The midbrain lies between the lower brainstem and the diencephalon. The superior and inferior colliculi exist on the dorsal surface of the midbrain and function in the control of eye movement, and relay signals for auditory and visual reflexes. THE DIENCEPHALON The diencephalon sits between the brainstem and the cerebrum. It is comprised of two main structures, the hypothalamus and thalamus, and two endocrine structures, the pineal gland and pituitary gland. The hypothalamus is a very small structure yet allows for homeostasis of a diversity of functions. These include the control of blood flow, the regulation of energy metabolism, the regulation of reproductive activity, and the coordination of responses to threatening stimuli/conditions (stress response). 14 Hypothalamic circuits receive both sensory and contextual information and compare them against biological set points to activate appropriate and relevant visceral motor, neuroendocrine, and somatic motor effector systems to restore homeostasis. A more detailed list of the functions of the hypothalamus is provided in the table below. 15 The thalamus is composed of nuclei that serve as a relay station for most sensory information. This includes sensory information from the ears, eyes, and spinal cord. Most sensory information from the lower parts of the CNS passes through the thalamus and the information has the ability to be modified within the nuclei. The thalamus also contains association nuclei which function to receive information from and project back to the cerebral cortex. Endocrine functions of the diencephalon: The diencephalon also contains the anterior and posterior pituitary structures which are responsible for the release of hormones into the blood. The posterior pituitary secretes hormones that are synthesized in the hypothalamus and transported down the tubuloinfundibular stalk to the posterior pituitary. These hormones, as you will learn later in this course, are vasopressin and oxytocin. The anterior pituitary receives hypophysiotropic hormones (hormones from the hypothalamus) through the portal median eminence (lacks BBB) which act to stimulate the synthesis and release of hormones from the anterior pituitary. The anterior pituitary gland is the perfect example of how control loops regulate endocrine function. The communication between the hypothalamus, the anterior pituitary, and the target organs exists through negative feedback loops in which the target organ can negatively influence the hypothalamus and pituitary release and the pituitary can negatively influence release from the hypothalamus. The pineal gland is an additional endocrine structure within the diencephalon which acts to secrete melatonin into the bloodstream to regulate circadian rhythm. The melatonin within the pineal gland is synthesized from serotonin as daylight comes to an end. The pineal gland works in close coordination with the suprachiasmatic nuclei of the hypothalamus to regulate sleep-wake cycles. THE CEREBELLUM The cerebellum, located below the base of the skull and above the brainstem, has a primary role in movement coordination. The cerebellum receives sensory information and coordinates the execution of fine motor movements. The cerebellum also has a function in relation to the 16 vestibular system, where it acts to receive information from somatic receptors in the periphery of the body (propioceptors) and from receptors within the inner ear to modify movement and position of the body in space. More specifically, the following regions of the cerebellum have the following implications: Vestibulocerebellum – control of balance and eye movement Pontocerebellum – planning and initiation of movement Spinocerebellum – synergy (rate, force, range, and direction of movement) THE CEREBRAL CORTEX The cerebral cortex is divided into two hemispheres that are connected by a structure called the corpus callosum. This structure is composed of axons that promote interhemispheric communication. It is the largest white matter structure in the brain, being composed of 200-250 million axonal projections. Like the spinal cord, the cerebral cortex consists of regions of both gray and white matter. Gray matter within the cerebrum is divided into three regions: 1. Cerebral cortex – gray matter exists in the outer layers of the cerebral cortex, where axonal projections from these cell bodies dive down into the deepest cortical layers to form white matter 2. Basal ganglia – a region involved in the control of movement 3. Limbic system – a primitive cerebrum, the limbic system acts to link higher cognitive function with emotional responses. Regions of the limbic system include the amygdala, cingulate gyrus, and hippocampus. The mature cerebrum has a distinct convoluted appearance that allows for maximal cerebral surface area, reflecting the overall capabilities of the cortical structure. This surface is characterized by sulci (grooves) and gyri (protrusions). 17 Lobes and functional areas of the cerebral cortex: The cerebral cortex is specialized into somatic, visual, auditory, olfactory, and gustatory sensory areas for perception; motor areas that direct movement; and association areas that integrate information. Each hemisphere of the cerebral cortex is divided into four lobes: frontal, parietal, temporal, and occipital. These lobes are associated with specialized functions. The frontal lobe coordinates information from other association areas, controls behavior, and is involved in executive functions The parietal lobe contains the primary somatosensory cortex and sensory association area and is involved in processing sensory information from the skin, musculoskeletal system, viscera, and taste buds The temporal lobe contains auditory association areas and the auditory cortex, involved in hearing and understanding language The occipital lobe contains the visual association area and the visual cortex and is involved in vision Cerebral lateralization: The two hemispheres that make up the brain are not completely identical in structure or function; each lobe has special functions not shared by the matching lobe on the opposite side. This concept is called cerebral lateralization and some functions that appear to display hemispheric 18 dominance include language and verbal skills, spatial visualization skills, and emotion. The figure below depicts some executive functions that are limited to one hemisphere. NEUROTRANSMITTER AND NEUROMODULATORY SYSTEMS IN THE BRAIN The brain functions using a variety of neurotransmitter and neuromodulatory chemicals. Prominent neurotransmitter systems include the glutamatergic and GABAergic systems which promote CNS excitation and inhibition, respectively. Additional neuromodulators including noradrenaline, serotonin, dopamine, and acetylcholine also act on CNS structures and modulate function. Norepinephrine (NE) – The source of NE is the locus coeruleus of the pontine brainstem structure and these projections innervate areas of the cerebral cortex, thalamus, olfactory bulb, cerebellum, midbrain, and spinal cord. The functions that are affected by NE include attention, arousal, sleep-wake cycles, learning and memory, anxiety, pain, and mood. Serotonin (5-HT) – The raphe nuclei of the brainstem are the source of the cell bodies of serotonergic neurons and these project throughout cortical limbic structures and also to the spinal cord. Serotonergic transmission has implications in sleep-wake and circadian cycles, emotion, pain, and locomotion. 19 Dopamine – Dopaminergic nuclei exist within the substantia nigra of the midbrain and project towards cortical and limbic structures. These projections are implicated in motor control and reward circuitry of the basal ganglia circuits. Acetylcholine – Cholinergic nuclei exist at the base of the cerebrum, pons, and midbrain and project throughout the cerebrum, hippocampus, and thalamus. These projections impact arousal centers, learning and memory, and sensory information integration in the thalamus. 20 COMPLEX BRAIN FUNCTIONS Arousal: Our CNS must fluctuate between different states of arousal, leading to different levels of consciousness. The reticular activating system, a diffuse collection of neurons in the reticular formation of the brainstem, keeps our nervous system activated and sleep-wake cycles in order through the activation of noradrenergic (NE) and cholinergic (ACh) neurotransmission to higher brain structures. Sleep: Although there are many hypotheses associated with why sleep is required, it appears that most theories hinge on the idea that sleep provides our nervous system and body a recovery period in which energy can be conserved and restored. Sleep has also been demonstrated to be crucial in the consolidation of short-term memories into long-term memories. Sleep is an unconscious state, that although characterized as a rest phase, exhibits marked neural activity that at some points exceed that observed during waking states. With that being said, sleep is divided into four distinct stages that can be classified by somatic changes and EEG responses. As an individual moves from awake to sleep states, they complete a transition through rapid-eye-movement (REM), transition, and slow-wave-sleep (SWS) states. Each of these phases is characterized by a successive decrease in the frequency of EEG waves that exhibit increasing amplitude. Individuals cycle many times through each sleep stage throughout the duration of the night. 21 Circadian Function: Circadian rhythm (cycling throughout the day) is critical in a number of our physiological variables and activities. For example, sleep and wake states are dictated by circadian cycles. Other things that are modified by circadian influence are cardiovascular activity, temperature, endocrine function, energy/metabolism, and locomotor activity. These events are a result of the entrainment to external light cues via the retinal ganglion cells (photoentrainment) and an endogenous biological clock that resides in the suprachiasmatic nuclei of the hypothalamus. Together, internal and external cues combine to influence the duration of our circadian day and the physiological variables that should be modified throughout the course of it. Emotion: The limbic system consists of a network of brain structures that dictate emotional responses. The limbic system structures include the prefrontal cortex, amygdala, hippocampus, thalamus, hypothalamus, olfactory bulb, parahippocampal gyrus, and basal ganglia. The limbic system integrates information received by the cerebral cortex (sensory stimuli) and ultimately may act on centers within the hypothalamus and brainstem to initiate responses that include somatic motor responses, 22 autonomic activation, endocrine secretion, and immune system activation. Motivation: Motivation is defined in your textbook as internal signals that shape voluntary behaviors, including those such as eating, drinking, sexual interaction. Motivational states generally include an increased drive in which they create an increased state of CNS arousal or alertness, create goal-oriented behavior, and are capable of encouraging detrimental behaviors to achieve goals. Motivation is a consequence of both limbic (discussed above) and basal ganglia circuitry. The basal ganglia structure most heavily implicated in motivational behavior consists of the GABAergic and dopaminergic cell populations within the ventral tegmental area (VTA). Learning and Memory: Memory is split into two broad categories. The first category makes the distinction between the type of memory that is being made/stored. This includes procedural memory and declarative memory. Procedural memory (reflexive/implicit) – related to knowledge of rules of actions and procedures, which can become automatic with repetition Declarative memory (explicit) – involves explicit information about facts (remembering a telephone number or name) and includes what we know consciously 23 Memory can also be split into short-term and long-term categories. Short-term memory – includes recent events from a few seconds up to several hours Long-term memory – includes information that is kept for longer periods, up to a whole lifetime Working memory – a form of short-term memory that is processed in the prefrontal lobes, allowing one to put pieces of information together to complete a task…for example, working memory allows one to remember and repeat a telephone number The inability to remember is called amnesia and it can occur as retrograde or anterograde. Retrograde amnesia – the inability to remember information previously acquired Anterograde amnesia – the inability to remember newly acquired information Learning is the acquisition of new knowledge and can be classified into two broad types: associative and non-associative. Associative learning – occurs when two stimuli are associated with each other (think Pavlov and the bell) Non-associative learning – a change in behavior that takes place after repeated exposure to a single stimulus (e.g., sensitization and habituation). Sensitization is the exposure to a noxious or intense stimulus that creates and enhanced response upon subsequent exposure. Habituation is the decrease in response to a stimulus that is repeated over and over. 24 Speech and Language: Speech and language capabilities are both lateralized and localized. Lateralization refers to the knowledge that known speech centers responsible for understanding language and producing speech are restricted to the left hemisphere. Localization refers to the idea that the ability to understand language and produce speech are defined into distinct regions of the frontal and temporal lobes. For example, the ability to understand spoken word is a result of the function of Wernicke’s area within the temporal lobe, while the ability to generate speech is a result of the function of Broca’s area within the frontal lobe. Damage to either Wernicke’s or Broca’s areas will result in a condition called aphasia. Receptive aphasia (Wernicke’s aphasia) is the result of damage to Wernicke’s area, where an individual is no longer capable of understanding and making sense of sensory input. Expressive aphasia (Broca’s aphasia) is the result of damage to Broca’s area where an individual is no longer able to speak or write in normal syntax. A third type of aphasia, conductive aphasia, results from damage to the axons that connect these two speech regions. 25 THE INTEGRATED CONTROL OF BODY MOVEMENT Movement is classified into three basic categories 1. Reflex movements – least complex and normally processed within the spinal cord, although higher brain centers may participate. An example is the knee jerk reflex diagramed below. Postural reflexes – movements which allow for the maintenance of body posture and position in space, requiring input from sensory systems including the vestibular, visual, and muscle receptors. 2. Voluntary movements – complex behaviors that require integration at the level of the cerebral cortex and are initiated at will 3. Rhythmic movements – movements requiring both reflex and voluntary movements (e.g., running and walking), generally initiated by higher brain structures but sustained through spinal reflex circuits (called central pattern generators) The CNS integrates movement on three levels 1. The spinal cord – integrates spinal reflexes and contains central pattern generators capable of sustaining rhythmic activity 2. The brainstem and cerebellum – integrates vestibular and ocular input to control postural movements 3. The cerebral cortex and basal ganglia – integrate and control complex voluntary motor movements The interaction of these systems is best depicted in the figure below, where postural reflexes and spinal reflexes do not require integration within the higher cortical centers. However, it is possible for sensory input to reach higher cortical sensory centers where it can be used to plan voluntary movement behaviors. 26 Voluntary movements require the coordination between the cerebral cortex, cerebellum, and basal ganglia. This is described in the figure below, where the control of voluntary movement is divided into three distinct steps. 1. Planning movement 2. Initiating movement 3. Executing movement With this process, sensory feedback allows the brain to correct for any deviation between the planned movement and the actual movement 27 Initiation of movement: The Corticospinal Tract Axons from neurons existing in the motor regions of the cortex extend down to the spinal cord where they synapse onto somatomotor neurons. These tracts come in two forms. Lateral corticospinal tract – The corticospinal tracts originate from neurons in the primary motor cortex, precentral gyrus, and frontal lobe. These axons descend through 28 the cerebral hemisphere, integrate with information of the basal ganglia and thalamic circuits, and proceed to descend down toward the midbrain. Within the midbrain, lateral corticospinal tracts decussate (cross the midline at the pyramids). The axons descend down toward the spinal cord to synapse onto somatic motor neurons in the anterior horn that innervate skeletal effector muscles. It is important to consider that because of the decussation in the medullary region of the brainstem, the movements of the right side of the body are generally governed by the opposing hemisphere (left). Anterior corticospinal tract – This tract includes a small bundle of axons that transcend from the cerebral cortex to the spinal cord in an uncrossed fashion. These axons synapse onto second order neurons within the spinal cord. 29 STUDY GUIDE QUESTIONS CNS PHYSIOLOGY I AND II 1. What is the role of neural networks and why would one argue that the functional unit of the nervous system should be changed from an individual neuron to a neural network? 2. Compare and contrast the nervous systems of lower organisms (jelly fish and worms) and higher organisms (humans). What do these systems ultimately serve the purpose of doing? 3. What is the difference between gray and white matter? What cellular structures do these refer to and how are they termed differently between the CNS and PNS? 4. Draw a figure of the layers of protection for the brain and spinal cord. While drawing these layers, address the distribution of CSF throughout the brain and spinal cord. 5. What is the purpose of the BBB and how is it formed? 6. What cells are responsible the secretion of CSF into ventricles and how is its composition different from that observed in plasma? 7. Define the function and structure of neurons within the CNS and PNS. 8. Define the function of the glial cells within the CNS and PNS. 9. Draw a transected spinal cord, labeling the entry of sensory information and the nuclei they synapse onto. Additionally, draw the cell bodies (nuclei) of the areas where motor information exits the spinal cord. 10. Define the primary structure and function of each component of the CNS, and how they have been subdivided. 11. With regards to cranial nerves from the midbrain, specify which are motor, sensory, or mixed. 12. Where will you find gray matter within the CNS and what do these regions have implications for? 13. What is the purpose of the corpus callosum? 14. What does cerebral lateralization mean? 15. What modulatory systems are important in regulating complex functions in the CNS and where are their nuclei (cell bodies of origin) located? What do they ultimately have an influence on? 16. Comment on the requirements and stages of sleep and how specific CNS systems may govern how our body handles sleep-wake cycles. 17. Describe the limbic system and how it integrates information to generate emotional and visceral responses. 18. Distinguish between short-term and long-term memory, and also contrast the two different forms of memory (implicit vs. explicit). 19. How is language understood and generated within the CNS? Is there a hemispheric dominance? 30 20. Describe the three types of movement that can be initiated? With regards to voluntary movements, what primary neural pathways do these follow to initiate action at effector muscles. Diagram it from the primary motor cortex all the way down to the effector muscle of a quadriceps. 31