CBI 1: Fundamentals of Chemistry PDF
Document Details
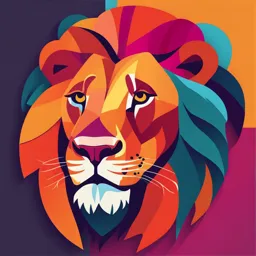
Uploaded by RicherCynicalRealism3520
Tags
Summary
This document provides a general overview of fundamental chemistry concepts. It covers topics such as atomic structure, bonding, and reaction nomenclature. This will be useful for students taking basic chemistry courses.
Full Transcript
CBI 1: Fundamentals of Chemistry Atom – smallest particle with properties of the given element - Contains protons, neutrons and electrons - Z = number of protons - The number of protons determines the number of electrons in an atom and consequently dictates the ways in which a...
CBI 1: Fundamentals of Chemistry Atom – smallest particle with properties of the given element - Contains protons, neutrons and electrons - Z = number of protons - The number of protons determines the number of electrons in an atom and consequently dictates the ways in which atoms of a particular element interact and participate in chemical reactions - A = atomic mass, the number of protons and neutrons - Electrons have 1/2000 mass of a proton, therefore their mass is negligible Unified mass unit: 1/12 mass of a atom of carbon-12 - Also can be in the form of dalton (Da) - e.g. 1H or 1.008 Da Periodic Table – arrangement of elements by atomic number (Z) Ionization energy: energy required to eject an electron outside atom or molecule in its ground state (measure of energy change when electron is removed) Electron Affinity: energy change that occurs when an additional electron is attached to a neutral atom or molecule in its ground state Electronegativity: power of an atom of a given element to attract electrons to itself - Estimated by taking average of ionization energy and electron affinity Atomic radius: distance from center of nucleus to outermost shell of electron in an atom Isotopes: same element different mass number (different number of neutrons) - Carbon has two stable isotopes and 1 unstable one o Carbone 12: stable and most abundance o Carbon 13: stable, used in nuclear magnetic resonance spectroscopy o Carbon 14: unstable - Hydrogen o Hydrogen 1: stable and most abundant isotope o Hydrogen 2: stable, used in labeling studies and kinetics Most elements occur as a mixture of isotopes. Nuclide Symbols Mass number X Atomic number Rutherford-Bohr model: positively charge nucleus surrounded by negatively charged electrons in specific orbits - Not accurate for atoms with more than one electron Quantum mechanical model - Electrons have wave-like properties - Behavior of these waves follow the Schrodinger wave equation (solution of wave equation is wave function) - Wave function describes probability of electron found at a particular point in space in/around the atom - Solving wave equation will give different probability densities depending on energy of the atom Atomic orbitals: regions of space in an atom where there is high probability of finding an electron (described by 4 quantum numbers) Quantum numbers: the size, shape, orientation and properties of the electron - Principal (n) - shell (larger value means higher energy and further away from nucleus) - Orbital (l) - s, p, d, f (0,1,2...) o Orbitals that have same energy can be described as degenerate - Magnetic (m1) - orientation of orbital (e.g. 0 for s orbital, 3 sorts of p orbital : -1, 0,1) - Spin (ms) - follows pauli exclusion principle where electrons in the same orbital spin in opposite directions Orbital occupancy: - Electrons occupy lowest energy orbital first (aufbau principle) - Electrons fill seperate before pairing (Hund’s rule) - No two electrons can have the same set of four quantum numbers (Pauli’s exclusion principle) Madelung Rule: at higher energies the orbitals energies begin to overlap, hence orbital fillings become a bit complicates Lewis Dot Structure: 1) Draw atomic symbol 2) Start filling valence electron shell 3) Don’t make pairs unless you have too 4) Ignore the difference between s and p 5) Bring together two atoms to deduce how electrons could be transferred (ionic bonding) or shared (covalent bonding) to make all the atoms appear to have a full valence shell 6) Match up unpaired electrons to make covalent bonds Ionic Bond – transfer of electron to more electronegative atom - Contains a cation (positively charged) and an anion (negatively charged) Covalent Bond – sharing electrons (less than 1 difference in electronegativity) Polar electronegative bond – electrons not equally shared - The more electronegative atom attracts the electrons in the covalent bond, polarizing the bond Hill notation for chemical formula: - For organic molecules we write the number of carbon atoms, then number of hydrogen atoms and then number of all the other atoms of the various elements in alphabetical orders - Ionic compounds are exceptions: write the positive ion first, then the negative ion - Oxides, acids and hydroxides are also exceptions o Oxides will end in multiples of O, no matter what the alphabetical order o Formula for acids begins with hydrogen atom o Hydroxides end in OH Structural formula – order of chemical groups (writing each chemical group one after another) e.g. CH3CH2CH2I Skeletal formula: - Lines represent bonds between atoms that lie at each end - Beginning or end of a line represents a carbon - Intersection of lines implies carbon atom - Each carbon atom has enough hydrogen atoms to give it a total of four bonds - Hydrogens are not shown they are implicit - Hydrogen atoms attached to non-carbons are shown VSEPR theory: valence, shell, electron, pair, repulsion e.g. linear, trigonal planar, tetrahedral Valence Bond theory - Overlap of atomic orbitals: a covalent bond is interpreted in terms of the overlap of atomic orbitals o Sigma bonds are formed by the end-to-end overlap of s, p, d, f orbitals o Pi-bonds are formed when p, d, f orbitals overlap sideways (above, below, behind or in front of the bond axis - Hybridization: combining the atomic orbitals in a valence shell to make a new set of orbitals that includes hybridized orbitals Molecular Orbital theory: describes molecular orbitals as regions of space where electrons are likely to be found in molecules - Can accurately predict bond lengths and bond energies CBI 2: Fundamentals of Chemistry Moles – number of discrete particles/entities it contains - Avogadro's number: 6.022 * 1023 Molar mass – mass in grams of one mole of a substance (g mol-1) - Number of moles = mass/ molar mass 1 g mol-1 = 1 dalton = 1 Da = 1/12 of the mass of a carbon-12 atom n = m/M n = the amount of substance (in moles) m = the mass of the substance (in grams) M = the molar mass of the substance (in grams per mol) Molar concentration – amount of solute divided by volume of solution - number of moles / volume of solution - molarity: the molar concentration of a solution in terms of the amount per unit volume c=n/V When you substitute n, you get c = m / (Mr*V) Mass concentration – mass of solute divided by volume of solution 1 g/L = 1 g/dm3 Volume concentration – volume of solute divided by volume of solution 1 M = 1 mol / L = 1 mol / dm3 = 1 mol dm-3 c1V1 = c2V2 c1 = Concentration of the stock solution (we have already) V1 = Volume of the stock solution (to be used in the dilution) c2 = Concentration of the dilute solution (that we desire) V2 = Volume of the dilute solution (that we desire) Serial dilution – where the solution generated by one dilution step becomes the solution for the dilution in the next step - Serial dilution can rapidly generate solutions with low concentrations that would require handling of very small volumes were we to try and dilute the stock solution directly Dilution factor: volume of one sample aliquot in the total combined volume of the sample aliquot and the volume of the solvent used to dilute that sample aliquot (e.g. 1:10) Dilution ratio: ratio of the volume of one sample aliquot to the volume of the solvent used to dilute that sample aliquot (e.g. 1:9) Acids and Bases: RATE= k [A] ^order * [B]^order Water: K = rate constant At equilibrium, the rate of association and the rate of dissociation are equal. Therefore we can equate both rate equations to form: This can be rearranged to find the dissociation constant You can assume in equilibrium that h+ and A- are same value and equate it both to H+^2 Ionic product of water: - We get rid of the concentration of water as it is effectively constant and very high in comparison to the concentration of the ions Bronsted Lowry Acids and Bases: Acid – proton donor Base – proton acceptor high ka = low pka and vice versa Strong acid -> high dissociation -> high ka -> low pka Weak Acid -> low dissociation -> low ka -> high pka - Concentration of the undissociated acid and its conjugate base in solution will be equal Strong acid: A substance that completely dissociates to form a hydrogen ion and a conjugate base ion when placed in aqueous solution Weak acid: A substance that only partially dissociates to form a hydrogen ion and a conjugate base ion when placed in aqueous solution [H+] = SQRT (Ka * [HA]) pH: concentration of hydrogen ions in solution Because we know the ionic product of water is a constant value, we can use it to figure out concentration of H+ and OH- ions. Kw = 1 x 10-14 mol2 dm-6 [H+][OH-] = 1 x 10-14 mol2 dm-6 pH + pOH = 14 Buffers: allow biological fluids to remain within a pH range that can be tolerated by other biological molecules present. pH buffer: an aqueous solution that contains a conjugate pair that changes in pH very little when small quantities of acid or base are added PH buffer solution contains a weak acid in excess and conjugate base (and vice versa) - Addition of acid or base to buffer solution will cause little change to the pH - Buffers perform most effectively around their pH unit which is why we need different buffers for different applications - A buffer’s ability to neutralise added acid or base is determined by the concentrations of HA and A– in the solution. The greater the concentrations for a given ratio of [HA] to [A– ], the greater the overall buffer capacity. When [HA] exceeds [A–], the capacity for added base exceeds that of acid How to prepare buffer: - Solution of weak acid (which will partially dissociate in conjugate base) and solution of salt of the same acid (which will completely dissociate added to available conjugate base) The Henderson-Hasselbach Equation: The Henderson-Hasselbalch equation provides a mathematical relationship to calculate the pH of a buffer solution based on the concentration of the weak acid and its conjugate base - if pka =pH then log of ratio of associating acid to dissociating acid is zero, because acid is half dissociated - As the ratio of conjugate base to acid increases, the pH increases **when given two concentrations and unknown variables you can do simultaneous equations ** to find ratio of ionised to unionised part you can use this equation Limitations of henderson-he Factors that affect rate of absorption: - Route of administration: IV (intravenously), oral (tablets) etc - Dosage: concentration - Lipid solubility: Weak acid are absorbed primarily across the stomach Weak base are absorbed primarily across the intestine CBI 3: Biomolecular Bonding Structural isomerism: - Molecules that have the same composition/molecular formula, but differ in the order in which atoms are bonded together Stereoisomerism: molecules that have the same compositional/molecular formula and order in the bonding of their constituent atoms, but these differ in their three-dimensional arrangement in space - E/Z isomerism - Optical isomerism (enantiomer): pair of compounds that have the same compositional/molecular formula and order of bonding of their constituent atoms, but these differ in their three-dimensionl arrangement in space such that the pair are non-superimposable mirror images of each other o Stereocenter – 4 different functional groups attached around center atom o Enantiomers: non-superimposable mirror images of each other ▪ have identical properties when in a symmetric environment, apart from their interaction with plane polarized light - Diastereomers: stereoisomers that differ in the absolute configuration of one or more but not all stereocenters o non-mirror image, non-superimposable o Differ by one stereocenter (epimer) o Differ by more than one (diastereomer) o To identify how many are possible look for chiral centers (if there are none, then the cis/trans will allow for one) Anomer – specific type of epimer (forms at an anomeric carbon of a cyclic saccharide as a result of acetyl and hemiacetyl formation) ** you can seperate enantiomers using chiral coloum with stationary phase that contains chiral group Or turn them into diastereomers “react racemic mixture with a single enantiomer of a chiral compound” Cahn-Ingold-Prelog rules – R/S Remeber to send the H to the back (lowest priority pointing away from the observer) ** you can seperate enantiomers using chiral coloum with stationary phase that contains chiral group Or turn them into diastereomers “react racemic mixture with a single enantiomer of a chiral compound” Corn Rules: Intramolecular bonding: bonding within molecule Intermolecular bonding: between molecules Dipoles: - Charge is distributed across molecule - Difference in electronegativity can cause polarity of a molecule Permanent dipole interaction: non-symmetric distribution of charge across molecule London dispersion forces: transient dipoles that lead to attraction within and between molecules - electron distribution can induce dipole which causes domino affect to induce dipole on surrounding molecules - Larger molecule = greater LDF Hydrogen Bonds: very electronegative atom attracts electron deficient hydrogen Ionic bond: electrostatic force of attraction between positively and negatively charged groups Melting points of molecules: - Higher melting points has to do with better packing in the solid - More symmetry has increased order which increases melting points CBI 4: Biomolecular Interactions Thermodynamics: the relative energies between reactants and products, and the exchange of energy between system and surroundings - Can determine if a reaction will proceed spontaneously or not but does not tell us anything about the rate of reaction 1) Is the reaction exothermic or endothermic = look at enthalpy 2) Is the reaction spontaneous = look at Gibbs free energy 3) Does the equilibrium lie towards the reactant or products = look at equilibrium constant System – reactants and products in the reaction Surroundings – everything else outside system Boundary – where the two meet ** total energy in an isolated system does not change ** total energy of system and surroundings does not change Thermodynamic temperature: absolute measure of the average total internal energy (Kelvin, K) Heat: energy per mol (kJ mol^-1) Enthalpy: internal energy of a system plus the product of pressure and volume (total amount of energy that a chemical system possesses) - Kinetic energy --> can come in the form of moving electrons, vibration of atoms connected by bonds, rotation and translation of molecules etc. - Chemical potential energy --> covalent or ionic bonds between atoms/ions, intermolecular forces between molecules Bond energy: average amount of energy required (in gas phase and standard conditions) to break 1 mol of all bonds of same type within same chemical species as the bond energy (kJ mol^-1) ** why do we care? Tells us how much energy is required to break them but also how much energy is released when they are formed. By calculating difference between products and reactants we determine if energy is released or taken in. ΔH > 0 = endothermic reaction where products have greater chemical potential energy than reactants, so input of energy is required for reaction to proceed ΔH < 0 = exothermic reaction where reactants have greater chemical potential energy than products, so reactants give out energy in the form of heat Hess’s Law: the total enthalpy change that occurs for a given reaction is independent of the reaction pathway Entropy: a measure of energy dispersal at a given temperature (J mol-1 K-1), measure of energy dispersal at a given temperature ΔS(total) = ΔS(system) + ΔS(surroundings) ΔS >0 indicates that the reaction increases the dispersal of energy in the system ΔS one product (contains all the reactants) 2. Elimination reaction: two or more components (functional groups or atoms) are lost 3. Substitution reaction: one atom or functional group is replaced by another a. SN1: unimolecular nucleophilic substitution reaction i. Rate determining step depends on 1 reactant ii. For tertiary carbocation iii. First Ea: dissociation of leaving group to form carbocation iv. Electrophilic groups are central carbocation are strongly attracted through electrostatic force of attraction v. Second transition state occurs as function group of electrophile and nucleophile interact, before stable product is formed vi. Intermediates that are formed are transition states that represent local energy maximum b. SN2: biomolecular nucleophilic substituition reaction i. Rate determining step depends on 2 species ii. One group becoming attached to central atom, while another is detaching iii. For primary carbocation iv. Nucleophile (like negatively charged atom) is attracted to an electrophile (commonly a carbon attracted to an electrophilic element) v. Reaction causes configuration at carbon center to be inverted vi. Intermediates that are formed are transition states that represent local energy maximum 4. Rearrangement reaction: internal connectivity of atoms in a molecule is changed 5. Redox reaction: electrons are transferred from one reactant to another a. Oxidation is loss of electrons and increase in oxidation state b. Reduction is gain in electrons and decrease in oxidation state Nicotinamide adenine dinucleotide (NAD) is a major redox agent in biological reactions: the nicotinamide ring of NAD+ acts as the hydride/electron acceptor. Flavin adenine dinucleotide (FAD) is a common redox reagent in biological reactions: the ring system of FAD acts as a hydrogen/electron acceptor CBI 5: Structure and Function of Biomolecules Carbohydrates Empirical formula - (CH2O)n Monosaccharide Aldose: end in C=O Ketose: in the middle C=O Fischer projection: horizontal lines towards you, vertical lines away from you Monosaccharide nomenclature: Locate the carbonyl group Draw fischer projection Identify stereocenter furthest away from carbonyl group If the –OH group is on the left side of the stereocenter then its L-isomer or right side is D-isomer Cyclisation: when a carbonyl carbon of an open chain reacts via nucleophilic attack from one of the –OH groups in the chain - Forms a circle: from aldose = hemiacetal, from ketose = hemiketal Cyclisation creates a new stereocenter which forms new anomers, which are identified by α- (hydroxyl group other side) and β- (hydroxyl group same side) - to the substituent on the last carbon How to identify alpha or beta: 1) Identify anomeric carbon (carbon that has o in side chain and connected to main o) 2) Then check if both OH groups are pointing same direction or not Disaccharide: when two monosaccharides react to form a glycosidic bond in a condensation reaction - Naming of bond: α,β-1,2 glycosidic bond. Polysaccharide: long chain Important ones – cellulose (β-D-glucose via β-1,4 glycosidic bonds), starch and glycogen (α-D-glucose connected via α-1,4 glycosidic bonds), only glycogen has (a-1,6 glycosidic bonds) - If you connect 1,6 glycosidic bond to amylose it creates amylopectin - Starch contains both amylose and amylopectin, glycogen is amylopectin Structure: cellulose, chitin Post-translational modification: e.g. ABO blood groups Energy storage: glycogen, starch Lipids: long hydrocarbon chains with a carboxylic acid group at the end - Most importantly known for their amphiphilic properties Fatty acids: No C=C bond is saturated, C=C bond is unsaturated Trans/E opposite side, Cis/Z same side (leads to bend or kink in the chain) ** A fatty acid containing a total of 16 carbon atoms (in its hydrocarbon chain) and zero double bonds would be described as: 16:0 How to state the position of the double bond: 1. The ω- or n- notation starts counting at the methyl group (-CH3): ω-7 or n- 7 2. The IUPAC or C- notation starts counting at the carboxylic acid carbon (- COOH): 16:1(9) or C-9 Triacylglycerol: forms by carboxylic acid from fatty acid reacting with hydroxyl group from glycerol forming ester bond (condensation reaction) - Function: chemical energy storage molecules - The acyl groups (c=o) can different, this determines the exact physiochemical properties it has Hydrophobic tail and hydrophilic head (amphiphilic) Glycerophospholipid: two fatty acids and the remaining OH bond to phosphate that bonds to glycerol to form a phospholipid - Amphilic molecules: hydrophobic tail and hydrophilic head - Can have many head groups, including choline, serine etc - Ester bond between fatty acid and glycerol - Phosphate ester linkage from specific head group to rest of R Sphingolipid: sphingoid backbone (amino acid serine and long chain fatty acyl CoA) and fatty acid and head group - Sphingomyelins contain a phosphate group that is most commonly bound to a choline or ethanolamine group. They are mainly found in myelin sheaths. - Cerebrosides are the simplest sphingolipids and contain a monosaccharide as a head group and are found in the membranes of neurons. - Gangliosides are complex sphingolipids that contain an oligosaccharide as head groups with a minimum of one sialic acid unit; they make up around 6% of the lipids in the brain. Sterol Lipids: has a ring system that is rigid and hydrophobic, while the polar hydroxyl group is hydrophilic Lipid aggregation: - Lipids spontaneously aggregate so that their hydrophobic tails interact and their hydrophilic heads face out towards water - The hydrophilic heads have dipole interactions with surrounding water molecules and ionic interactions with surrounding ions in the solution - This is driven by the hydrophobic effect, where breaking hydrogen bonds in water is energetically unfavorable so the hydrophobic tails face towards each other - This maximizes LDF between fatty acid tails and head groups form electrostatic and hydrogen bonds with water molecules - Therefore the conformation adopted minimizes the repulsion and maximizes favorable interactions Main properties: They are cooperative structures They can extend and fuse with themselves They are self-repairing They are impermeable for ions and polar molecules Membrane fluidity: refers to the viscosity of the lipid bilayer of the cell membrane - Influenced by the types of lipids in the membrane - Less dense packing makes it more fluid, change in melting point and phase transition Influences – e.g. cholesterol and unsaturated fatty acids (typically both increase membrane fluidity) Nucleic Acids: Nucleotides: linear polymers that contain coded information that is transferred from one generation to the next - Nucleoside is without phosphate Guanine and ctyosine have 3 hydrogen bonds between Adenine and Thymine/uracil have 2 hydrogen bonds between Monomeric nucleotides: molecules with other functions like energy metabolism (ATP, GTP), second messenger molecules (cAMP), or substrates for enzymes (ATP, GTP) Proteins: Amino acids: α-carbon atom that is bound to an amino group (-NH2), a carboxylic acid group (-COOH), and a side chain (-R) that determines its individual and specific physicochemical properties. Primary sequence: chain of amino acids linked by peptide bonds Secondary sequence: hydrogen bonds between the backbone carbonyl and nitrogen atoms - This forms β-sheets, α-helices and hairpin bends/β-turns Tertiary sequence: covalent and non-covalent interactions between amino acid subunits - e.g. hydrogen bonds, ionic interactions, dipole interactions, disulfide bonds etc - Gives the protein its overall 3D structure, which is essential in its interactions with other substances Quaternary sequence: multiple polypeptide chains form together **proteins that facilitate correct folding are called chaperones, ensuring that they achieve functional three-dimensional structure In globular proteins, amino acids with hydrophobic side chains are typically situated in the center of the protein in the core - Interactions between hydrophobic side chains is through London forces In globular proteins, amino acids with polar and ionizable side chains are typically situated in the exterior of the protein to interact with the aqueous/cytosol - Interactions between ionizable side chains is through London forces and as salt bridges (i.e. electrostatic interactions). Protein purification: 1. Lysis: releasing proteins by disrupting/destroying plasma membrane 2. Isolate specific protein a. Size-exclusion chromatography: seperates via size b. sample applied to column that contains beads with pores, small molecules penetrate. c. Order of elution: from large to small d. Ion-exchange chromatography: amino acids separating by charge (occurs due amino acid side chain) by interacting with beads via ionic forces i. Isoelectric point: the pH where protein has overall net charge of zero ii. Due to their charge, the proteins can interact via ionic forces with beads that have been modified with a charged group iii. Molecules with same charge as the matrix will flow through column iv. Proteins of interest can be eluted by changing the pH or using high ion concentrations that replace the protein from the beads e. Affinity Chromatography: specific amino acids or whole protein have tags. These tags load onto a column and interact with column matrix, non-tagged items will flow through. Protein then eluted with high concentrations of small molecules that interact with column matrix or tag itself. f. SDS- PAGE: separate molecules by size i. Biomolecules are given negative charge ii. Detergent denatures the proteins, due to its sulfate head group, and gives each protein an overall negative charge iii. Current is applied, proteins move towards positively charged anode iv. Larger molecules take longer to travel through polymer mesh while smaller ones can pass quickly through v. Proteins colored with blue dye, which makes them appear more visible vi. To determine size of bands, a marker is applied that contains proteins of known sizes Bacterium were modified to express protein with His-tag 1. Affinity chromatography with Ni-NTA column 2. SDS page Both methods were done to separate the protein of interest from the rest 3D Protein structure: 1. Protein function 2. Protein interaction with other ligands 3. Drug design and development 4. Disease mechanisms 5. Application in other fields SEC-purified His-tagged protein --> functional assays for protein characterisation --> protein structure determination = x-ray crystallography, cryo-electron microscopy, NMR -- > 3D protein structure CBI 6: Enzymes and Kinetics of Biocatalysis Enzymes: catalyze biochemical reactions by lowering the energy required to reach the transition state Enzymes bind specific substrates at their active site: - The substrate binds to the active site of the enzyme, forming a reaction intermediate called the enzyme-substrate complex - This interaction between the substrate and the functional group in the active site of the enzyme lowers the activation energy by providing an alternative reaction pathway - Following the formation of the enzyme-substrate complex, the reaction mechanism will give rise to product and free enzyme - The enzyme is released and ready to bind more substrate Classification of Enzymes: - At low substrate concentration: rate of reaction is directly proportional to concentration of substrate (first-order kinetics) - At high substrate concentration: rate of reaction is unaffected by increase in substrate concentration (zero-order kinetics) - The maximum velocity of the enzyme = all enzyme molecules are occupied, reaction equilibrium is reached The Michaelis-Menten equation: k1 = rate constant for the formation of enzyme-substrate (ES) complex from the enzyme (E) and the substrate (S). k-1 = rate constant for the reverse reaction, the dissociation of the enzyme- substrate complex (ES) to form enzyme (E) and substrate (S). k2 = rate constant for the formation of product (P) from the enzyme-substrate complex (ES). This is also commonly known as kcat. **Km is specifc to each enzyme and independent of enzyme concentration ** low Km means low dissociation constant which represents high affinity of the enzyme to its substrate - Km is the substrate concentration at half of Vmax The Lineweaver-Burk plot: - Bc Vmax can never be reached only approached, this means we must find another way to find Km kcat = k2: max number of chemical conversions of substrate molecules per second that a single catalytic site will execute for a given enzyme that is saturated by its substrate. - the smaller the KM and the larger the kcat, the more efficient an enzyme will be KM The Michaelis-Menten constant, defined as the substrate concentration at half the maximum velocity (um) Vmax The rate of reaction when the enzyme is saturated with substrate is the maximum rate of reaction (mol/min) Kcat A measure of the number of substrate molecules "turned over" by enzyme per second. Cooperativity: substrate binding changes substrate binding affinity - Non-cooperative: substrate binding has no effect on substrate binding affinity - Positive cooperativity: positive effect on substrate binding affinity - Negative cooperativity: negative effect on substrate binding affinity e.g. oxygen binding to hemoglobin (4 subunit protein) increases its binding affinity for oxygen (haemoglobin exhibits positive cooperativity - sigmoid curve when fraction of occupied sites is plotted vs pO2) e.g. myoglobin (monomeric) is non-cooperative (hyperbolic curve when fraction of occupied sites is plotted vs pO2). Reversible inhibitor: Competitive inhibitor Inhibitor resembles substrate and binds to active site Degree of inhibition depends of concentration of substrate The effects of competitive inhibitor can be overcome by sufficient substrate Km is increased, Vmax remains the same (more substrate is needed to obtain same reaction rate) Uncompetitive inhibitor Binds to ES complex to prevent release of product Cannot be overcome by addition of sufficient substrate Vmax is reduced, Km is reduced by the same amount ES is depleted by this inhibitor so due to reaction equilibrium, K1 increases and more substrate binds to enzyme If K1 increases, Km decrease because lower substrate concentration is required to form half the maximal concentration of ES Non-competitive inhibitor Binds at another site, does not prevent substrate molecule from binding Vmax is decrease, Km is unchanged bc inhibitor lowers concentration of available functional enzyme but does not affect the binding of the substrate Axis titles: x-axis = 1/substrate concentration (units 1/mM) y-axis = 1/V, where V is initial velocity (units mins/mM) - In general, warm conditions = higher KE = optimal enzyme activity - However high temperatures can inactivate enzymes bc molecules vibrate and twist that non-covalent bonds breaj - Proteins loses secondary and/or tertiary structure and becomes inactive Cofactors (non-protein chemical or metal compounds that enzymes require in order to function) Allosteric regulation of enzymes: - Allosteric binding causes conformational change in the enzyme that can result in any type of inhibition - Allosteric effects can also result in activation of an enzyme - There can be a number of allosteric site on the enzyme - Can alter both Vmax and Km - Allosteric effects in multi-subunit proteins: At low substrate concentration, the reaction rate increases as substrate concentration increases. After the substrate binds to the first active site of the enzyme, there is a change in the enzyme’s quaternary structure such that the other sites become more likely to bind substrate, so the reaction speeds up. Once all the sites are saturated with substrate the reaction rate reaches a plateau. What affects activity of an enzyme (recap): - PH - Temperature - Inhibitors - Covalent modifications (like phosphorylation of critical amino acid side chain can either activate or inactivate an enzyme) CBI 7: Glycolysis and Gluconeogenesis C6H12O6 + 6 O2→ 6 CO2 + 6 H2O Glycolysis: the breakdown of glucose. It is the first step in glucose metabolism and results in the production of pyruvate, NADH, H+ ions, ATP and water. Glucose + 2 NAD+ + 2 ADP + 2 Pi → 2 Pyruvate + 2 NADH + 2 H+ + 2 ATP + 2 H2O Step 1: trapping glucose and preparing it to be cleaved - investment phase: Steps in glycolysis that require the input of energy, provided by the hydrolysis of ATP 1.1 Glucose to glucose-6-phosphate - Phosphorylation is catalyzed by hexokinase o Hexokinase contains active and inactive forms depending on glucose conc - Reaction inhibited by glucose-6-phosphate (negative feedback) - G6P cannot exit cell, advantage to not produce more G6P so glucose can escape the cell and go where needed - ATP to ADP - Reaction is irreversible (glucose cannot leave cell now) - bc it cannot diffuse out by facilitated diffusion o This is because the phosphate has a negative charge which prevents it from diffusing through the membrane 1.2 glucose-6-phosphate to fructose-6-phosphate - This isomerism creates a resonance structure which has more stability, and makes the beta bond more reactive - This leads to break of C-C bond due to beta bond reactivity which is needed to cleave glucose in step 2 - It allows production of two/three carbon metabolites rather than one/two and one/four 1.3 fructose-6-phosphate to fructose-1,6-bisphosphate - Second phosphorylation in the first carbon - ATP to ADP - Irreversible - Catalyzed by allosteric enzyme phosphofructokinase (AMP is its heterotopic activator) - Can be inhibited by citrate: high levels of citrate signal that enough energy is present in the cell, thus it acts as an allosteric inhibitor for the phosphofructokinase Step 2: Lysis into 2 C3 units 2.1 fructose-1,6-bisphosphate to dihydroxyacetone phosphate and glyceraldehyde-3- phosphate - Reversible reaction - Catalyzed by enzyme aldolase 2.2 dihydroxyacetone phosphate to glyceraldehyde-3-phosphate - From a ketose carb to an aldose carb - Reversible reaction - As glyceraldehyde is used up in the following steps, the equilibrium shifts to the right - From now on all reactions will take place twice per glucose molecule Step 3: The payoff phase – because it produces energy in form of ATP and NADH ** these reactions all take place twice 3.1 gylceraldehyde-3-phosphate to 1,3bisphosphogylcerate - contains high energy intermediate form - First reaction step very high negative G, while second step very endergonic - Using the enzyme, the low energy intermediate is avoided (by formation of thioester intermediate) and reaction is catalyzed 3.2 1,3bisphosphoglycerate to 3-phosphoglycerate - ADP to ATP - This kind of reaction is known as substrate level phosphorylation as the phosphate donor has a very high phosphorylation potential 3.3 3-phosphogylcerate --> pyruvate - First phosphate gets moved to second position - Second step enolase catalyzes a dehydration reaction - Third step substrate level phosphorylation occurs, ADP to ATP (irreversible) Substrate level phosphorlyation: transfer of phosphate group from a molecule to ADP to form ATP Depending on presence of oxygen, pyruvate can be converted into different substances Use of extracellular acidification rate (ECAR) to explain glycolysis: - The change in ECAR on the addition of glucose is a measure of glycolysis. - The change in ECAR on the addition of oligomycin (i.e. oxidative phosphorylation is inhibited) is a measure of the total glycolytic capacity of the cell. - The difference between ECAR after the addition of glucose and the addition of Oligomycin is a measure of spare glycolytic capacity. - There is a basal ECAR which is basal pH that does not change with time and is a measure of non-glycolytic acidification. This is the pH of the extracellular fluid before the addition of glucose, and can be verified by the addition of 2- deoxyglucose (cannot undergo glycolysis) after the addition of glucose and oligomycin. Gluconeogenesis: Gluconeogenesis is the production of glucose from oxaloacetate. It is a mechanism to avoid hypoglycemia/low blood glucose. Energy is required to generate glucose. 2 Pyruvate + 4ATP + 2GTP + 2NADH + 6H2O --> Glucose + 2NAD+ + 4ADP + 2GDP + 6Pi + 2H+ Occurs in: liver and adrenal cortex (kidney) An alternative set of enzymes is used to bypass the high gibbs free energy in the unidirectional reaction of glycolysis. Glycolysis Step 1.1 (last step of gluconeogenesis) - Reverse glucose-6-phosphate to glucose - Catalysed by glucose-6-phosphotase, a membrane bound protein in the ER - This reaction takes place in the ER lumen Glycolysis Step 1.3 - Reverse fructose-1,6-bisphosphate to fructose-6-phosphate - Catalyzed by fructose-1,6-bisphosphotase Last step of glycolysis step 3.3 - catalyzed by pyruvate carboxylase (PC) and phosphoenolpyruvate carboxykinase (PEP-CK) - Pyruvate --> oxaloacetate (this reaction takes place in mitochondrial matrix) - Oxaloacetate --> malate (so that it can be transported into cytoplasm - In cytoplasm malate converted back to oxaloacetate and then decarboxylated and phosphorylated by phosphoenolpyruvate carboxykinase Alternative GNG precursors: - Lactate - Glucogenic amino acids - Glycerol Control of blood glucose concentration: Normoglycemia - The condition in which there is a normal concentration of glucose in the blood, essential for proper metabolic functioning Hypoglycemia - The condition in which there is a concentration of glucose in the blood lower than the range considered normal Hyperglycemia - The condition in which there is a concentration of glucose in the blood higher than the range considered normal After a meal, blood plasma glucose levels increase and then decrease as glucose is subsequently up taken by different cells/organs. The glucose taken up by cells is used as substrate for glycolysis. Glycolysis is a catabolic pathway that is switched on when we have high levels of glucose in our blood or need it for other processes. When glucose levels are low glycolysis is switched off, so if glucose is still needed other processes like (gluconeogenesis) are switched on. This allows glucose to be produced and released to the blood to maintain concentration within normal range. Fast regulation of glucose - Inhibiting or activating the enzymes involved in glycolysis and gluconeogenesis Slow regulation of glucose - When blood glucose levels fall, glucagon is released form alpha cells in pancreas - Glucagon activated gluconeogenesis and the conversion of stored glycogen to into glucose - When blood glucose levels are high, beta cells of pancreas release insulin that activates glycolysis - Slow regulation involves hormonal changes. These hormones work by altering expression of the genes that code for the enzymes in glycolysis and gluconeogenesis. Thus this regulation is much slower Biologically relevance: In-vivo: in the naturally occurring conditions and environment Ex-vivo: not in the naturally occuring conditions and environment Entner-Doudoroff Pathway: alternative pathway to glycolysis that is held by many bacteria 1. Hexokinase – requires ATP 2. G6P dehydrogenase – requires NADP+. Forms a cyclic metabolite, 6- phosphogluconolactone 3. 6-phosphogluconolactonase (a hydrolase – so + H2O) then opens ring to give 6-P- gluconate 4. 6-phosphogluconate dehydratase – requires water 5. KDPG aldolase: gives 2/3 carbon metabolites **first two steps are in humans as part of pentose phosphate pathway It yields only 1 ATP molecule per glucose because there is only 1 glyceraldheyde-3- phosphate per glucose CBI 8: Krebs Cycle and Oxidative Phosphorylation Oxidative Decarboxylation: Reaction that simultaneously reduces a compound and removes carbon dioxide. An example is the conversion of pyruvate to acetyl-CoA that reduces NAD+ to NADH while releasing one molecule of CO2. Pyruvate + NAD+ + CoA -> Acetyl-CoA + NADH + CO2 + H+ ** pyruvate is converted into acetyl-CoA by pyruvate dehydrogenase TCA/Krebs Cycle: Acetyl-CoA + 3NAD+ + FAD + ADP + Pi + 2H2O -> CoA + 3NADH + FADH2 + 3H+ + ATP + 2CO2 1) C4 and C2 molecules form together to make C6 a. This reaction is driven by hydrolysis of phosphodiester bond 2) Isocitrate is formed by dehydration step followed by hydration step 3) Oxidative decarboxylation which forms NADH and CO2 4) Oxidative decarboxylation released NADH and CO2 5) Energy transfer reaction a. Hydrolysis of energy rich phosphodiester powers substrate level phosphorlyation b. Formation of ATP (when high energy needed) or GTP 6) Oxidation to fumerate a. FAD covalently bound to enzyme b. Enzyme is embedded in the inner mitochondrial membrane and part of ETC 7) Hydration reaction (stereospecific) 8) Oxidation reaction which is unfavourable with high Gibbs free energy a. Driven by the use of its product NADH – consists of adenine, two ribose sugars, a diphosphate group and a nicotimadine group - Protons and electrons are added to nicotimadine ring when NAD+ is reduced to NADH - Example of a co-enzyme - Electron carrier - Involved in oxidative phosphorylation - NADH is a reducing agent in biological reactions FAD – comprised of ADP unit that is connected via a sugar moiety to an isoalloxazine ring. This ring is the active electron carrier part and can accept two electrons and protons. Acetyl-CoA: formed by beta-oxidation of a fatty acid Beta-oxidation: breakdown of fatty acids into acetyl-CoA Ketone bodies: - Under conditions of starvation, acetyl-CoA may be converted into ketone bodies to act as alternative fuel source - Too high concentration of ketone bodies can be life-threatening as it accumulates in the blood which leads to drop in pH. The result is an ketoacidosis that can impair tissue function and the central nervous system. - Ketoacidosis is a lowering of blood pH caused by the excessive production of ketone bodies Four enzyme complexes involved in ETC: - Complex I: NADH-Q oxidoreductase - Complex II: Succinate-Q reductase - Complex III: Q-cytochrome c oxidoreductase - Complex IV: Cytochrome c oxidase Ubiquinone: can be reduced by two electrons, highly hydrophobic and thus shuttles electrons through the inner mitochondrial membrane - Redox-active molecule, can transport electrons as part of ETC - a small molecule - Restricted to mitochondrial inner membrane by hydrophobic tail - Transports 2 electrons - Contributes directly to proton gradient - Transports electrons from complex I/II to III Cytochrome C: small water-soluble protein with a heme center and a iron inside the protein. Cyt C can carry one electron at a time. - Redox-active molecule, can transport electrons as part of ETC - A protein - Uses Fe bound to heme prosthetic group, transport 1 electron - Doesn’t contribute directly to proton gradient - Transports electrons from complex III to IV ETC: A series of enzyme complexes that perform redox reactions, coupling the transfer of electrons - from electron donors (NADH and FADH2) to the terminal electron acceptor (oxygen) - to the movement of hydrogen ions across the mitochondrial inner membrane into the mitochondrial intermembrane space. - NADH binds to complex I, it gets oxidised to NAD+ and proton and 2e - The two electrons formed are transported via internal metal clusters to ubiquinone which takes up two protons from the matrix to be reduced - This electron transfer allows complex I to transfer 4 protons from the matrix into intermembrane space - Complex II catalyses reaction from succinate to fumerate in kreb cycle, which leads to reduction in FAD - FAD covalently bound to enzyme complex where it transfers two electrons and protons to complex II - Electrons and protons are taken up by ubiquinone to form QH2 - Ubiquinone gives the electrons to complex III, this leads to release of 4 protons to intermembrane space, while another 2 protons are taken from matrix - The electrons are then transferred to cytochrome C (located in intermembrane space), two cytochrome c molecules are necessary to take both electrons from ubiquinone. - Cytochrome C shuttles the electrons to complex IV (happens 4 times) o Cyt C uses iron atom, coordinated as part of a haem complex, which can alter redox state from Fe(II) to Fe(III) - Complex IV catalyzes the reduction of molecular oxygen to water by taking up four protons from the matrix, it also pumps 4 protons across inner mitochondrial membrane - These protons generates proton gradient Proton-motive force: the energy that is created by a chemical gradient due to the difference in concentration of protons on each side, and a charge gradient due to the positive charge of these protons Proton motive force = chemical gradient + charge gradient ATP synthase: ATP synthase allows protons to flow through its channel, facilitating the rotation of its c subunit, which catalyzes ATP synthesis during conformational changes. - Proton flows through the intermembrane channel from the a subunit to the c subunit - Proton neutralizes the charge of glutamate inside the C unit due to the high pH - C unit rotates clockwise - Low pH on matrix side leads to release of protons in the matrix - The loose state in the beta subunit allows ADP and phosphate to bind to catalytic side for ATP synthesis - T state supports formation of ATP by tightly binding ATP - Open (o) state, amino acids rearrange in the active side so ATP can leave the enzyme - When this happens the conformational stage of all the three beta subunits changes. The loose conformation becomes tight, catalysing the formation of ATP, the tight conformation becomes open, being able to release ATP and the open conformation becomes loose, ready to bind ADP and inorganic phosphate. - Another rotation again causes the conformational change, closing the catalytic cycle of the subunits. Thus, the role of the proton gradient is not to drive the reaction but to release ATP from the synthase. ** rotation of the c ring leads to 120 degree rotation of gamma unit Malate/aspartate shuttle: NADH reduction, net yield of 2.5 ATP Glycerol phosphate shuttle: FADH reduction, net yield of 1.5 ATP Inhibitors: Oligomycin – inhibits ATP synthase - It decreases electron flow and therefore decreases mitochondrial respiration, in an ATP-linked fashion - ATP synthase utilises the chemiosmotic gradient across the inner membrane of - mitochondria to power ATP production. - The chemiosmotic gradient is produced/maintained by the complexes of the ETC. - Inhibition of ATP synthase prevents ATP formation from the passage of H+ ions - across the inner mitochondrial membrane and therefore the ETC does not yield ATP - in this way. - The processes in glycolysis, oxidative decarboxylation and Krebs/TCA cycle would be unaffected, although build-up of OXPHOS substrate would likely reduce TCA - throughput. - To maintain adequate ATP production, cells would likely increase glycolysis and fermentation of pyruvate, yielding lactate. Rotenone + Antimycin A – inhibits complex I and III - Leads to complete shutdown of ETC and hence mitochondrial respiration, it allows you to measure the rate of non-mitochondrial respiration 2-deoxy-D-glucose (an analog of D-glucose) - Hexokinase phosphorylates 2-deoxy-D-glucose in a similar way to D-glucose - The phosphorylated product is effectively trapped in the cell - 2-DG inhibits the production of glucose-6-phosphate in glycolysis and therefore reduces glycolytic flux and production of pyruvate - Processes in oxidative decarboxylation, Krebs/TCA cycle, and OXPHOS would be unaffected - The reduced input of pyruvate from glycolysis would reduce the production of acetyl-CoA and subsequent processes unless derived from another source Carbonyl cyanide-4-(trifluoromethoxy)phenylhydrazone (FCCP; an ionophore) - Complexes in the ETC utilize NADH and FADH2 to transport H+ into the intermembrane space as ATP synthase requires a chemiosmotic gradient across the inner mitochondrial membrane to be generated maintained - As a charged species, H+ cannot freely move across the lipid bilayers without assistance - FCCP as an ionophore has suitable physicochemical properties to enable H+ to move down the chemiosmotic gradient by facilitating its movement across the lipid bilayer of the inner mitochondrial membrane (and other membranes) - The chemiosmotic gradient cannot be maintained sufficiently well by the complexes in the ETC as FCCP transports ions from the intermembrane space to the matrix and therefore no ATP is produced by ion transport through ATP synthase if FCCP present. - Processes providing substrates for OXPHOS will likely increase but with no increase in ETC-related ATP production Warburg Effect: tendency of cancer cells to engage in aerobic glycolysis, even in the presence of sufficient oxygen. Cancer cells preferentially use glycolysis to break down glucose for energy, producing lactate as a byproduct, rather than relying primarily on oxidative phosphorylation. - This is for rapid ATP synthesis, to support rapid cell proliferation - Acidifying the tumor microenvironment Use in clinical treatment --> glycolytic inhibitors or PET imaging