Case 1 Notes.docx
Document Details
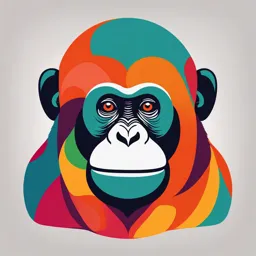
Uploaded by EasedPegasus
Full Transcript
THE STABBING Mechanics of Breathing The lungs do not have muscles that contribute to breathing; the small muscles they contain control the diameter of the airways. Air will only flow from a region of high pressure to a region of low pressure. Inspiration: the pressure in the elastic alveoli is made...
THE STABBING Mechanics of Breathing The lungs do not have muscles that contribute to breathing; the small muscles they contain control the diameter of the airways. Air will only flow from a region of high pressure to a region of low pressure. Inspiration: the pressure in the elastic alveoli is made low (lower than the outside atmospheric pressure) by stretching them by reducing the pressure around them by expanding the chest wall, thus increasing its volume. Air is thus sucked into the lungs Expiration: the pressure in the elastic alveoli is increased (higher than the atmospheric pressure outside) by decreasing the size of the chest, thus decreasing its volume, thereby compressing the gas in the lungs. The changes of volume in the lungs occur through the action of muscles: Intercostal muscles Diaphragm (separates the thoracic and abdominopelvic cavities) Accessory respiratory muscles Inspiration: Inhalation is an active process. Quiet Inspiration (eupnoea): Increase in vertical diameter: Contraction of diaphragm flattens the floor of the thoracic cavity, increasing its volume and so decreasing the intra-alveolar pressure, and drawing air into the lungs. Diaphragmatic contraction accounts for 75% of the air movement in normal breathing at rest. Increase in transverse diameter: Contraction of the external intercostal muscles elevate the ribs. This contributes to around 25% of the volume of air in the lungs at rest. The ribs curve downward as well as forward around the chest wall. They resemble bucket handles. If the ribs are raised, the transverse diameter of the thoracic cavity increases. Increase in anteroposterior diameter: Contraction of the Scalenei muscles causing the first rib to be fixed. This means that all ribs are drawn together and raised toward the first rib. The downward facing ribs are raised at their sternal ends, the anteroposterior diameter of the thoracic cavity is increased, and the sternum is thrust forward. Forced Inspiration: maximum increase in thoracic cavity occurs. Contraction of the accessory muscles assist the external intercostal muscle in elevating the ribs. These muscles increase the speed and amount of rib movement. The accessory muscles include: sternocleidomastoid, Scalenei muscles (anterior and medius), serratus anterior and pectoralis minor. In inspiration: Root of the lung descends and the level of the bifurcation of the trachea may be lowered by as much as two vertebrae. The bronchi elongate and dilate and the alveolar capillaries dilate, thus assisting the pulmonary circulation. Air is drawn into the bronchial tree as a result of the positive atmospheric pressure exerted through the upper part of the respiratory tract and the negative pressure on the outer surface of the lungs brought about by the increased capacity of the thoracic cavity. With expansion of lungs, the elastic tissue in the bronchial walls and connective tissue are stretched. As the diaphragm descends, the costodiaphragmic recess of the pleural cavity opens, and the expanding sharp lower edges of the lungs descend. Expiration: Exhalation is an active or passive. Quiet expiration: largely passive. Elastic rebound/ recoil of the lungs: This phenomenon occurs because of the elastic fibres in the connective tissue of the lungs and because of the surface tension of the film of fluid that lines the alveoli. As water molecules pull together, they also pull on the alveolar walls causing the alveoli to recoil and become smaller. When the muscles of inhalation relax, these elastic components recoil, returning the diaphragm, the rib cage, or both to their original positions. But two factors prevent the lungs from collapsing: surfactant (reduce surface tension) and the interpleural pressure. Forced expiration: active. The internal (and innermost) intercostal muscles and the transversus thoracis muscles depress the ribs and reduce the width and depth of the thoracic cavity. The abdominal muscles: External and internal oblique, transversus abdominis, and rectus abdominis muscles, can assist the internal intercostal muscles in exhalation by compressing the abdomen and forcing the diaphragm upwards. Forcible contraction of the muscles of the anterior abdominal wall. Quadratus lumborum also contracts to pull down the 12th rib. Serratus posterior inferior and the Latissimus dorsi muscles also play a minor role. In expiration: Roots of the lungs ascend. Bifurcation of the trachea ascends. Bronchi shorten and contract. Elastic tissue of the lungs recoils and the lungs become reduced in size. Diaphragm moves upwards. The lower margins of the lung shrink and rise. Control/ Regulation of Respiration Respiratory control has both voluntary and involuntary components: Involuntary: The involuntary respiratory centres regulate the activities of the respiratory muscles involved in quiet inspiration. They control the respiratory volume by adjusting the frequency and depth of pulmonary ventilation. Voluntary: This reflects the activity in the cerebral cortex that affects either: The output of the respiratory centres in the medulla oblongata and pons The motor neurons in the spinal cord that control respiratory muscles. The respiratory centres are three pairs of nuclei (clusters of nerve cell bodies) in the medulla oblongata and pons. The respiratory rhythmicity centres set the basic pace for respiratory movements. Respiratory Centres in the Medulla Oblongata: Dorsal Respiratory Group (DRG) Located in the dorsal portion of the medulla oblongata. DRG controls mostly inspiratory movements and their timing. It controls both quiet and forced inspiration. DRG’s inspiratory centre controls: The phrenic nerve which innervates the diaphragm. The intercostal nerves which innervate the external intercostal muscles. Nerves which innervate the accessory respiratory muscles involved maximal inhalation (scalenei muscles, sternocleidomastoid, serratus anterior and pec minor). Ventral Respiratory Group (VRG) Located in the ventrolateral part of the medulla. VRG mainly causes forced expiration. VRG’s expiratory centre controls: The intercostal nerves which innervate the internal (and innermost) intercostal muscles. Nerves which innervate the accessory respiratory muscles involved in active exhalation (abdominal muscles mainly). VRG’s inspiratory centre aids the DRG during forced inspiration. This centre controls: Nerves which innervate the accessory respiratory muscles involved maximal inhalation (scalenei muscles, sternocleidomastoid, serratus anterior and pec minor). When the respiratory drive for increased pulmonary ventilation becomes greater than normal, respiratory signals spill over into the VRG from the DRG. This activates the inspiratory centre of the VRG, allowing it to innervate the accessory respiratory muscles of forced inspiration. Inspiratory “Ramp” Signal: The signals sent from the respiratory centres to the respiratory muscles occur in bursts of action potentials. In normal respiration: The signals begin weakly and increase steadily in a ramp manner for about 2 seconds, providing the stimulation to the inspiratory muscles (inhalation occurs). Then, the signals cease abruptly for approximately the next 3 seconds, which turns off the excitation of the diaphragm and allows elastic recoil of the lungs and the chest wall to cause expiration (passive exhalation occurs). Next, the inspiratory signal begins again and this cycle repeats again, with expiration occurring in between. Advantage: causes a steady increase in the volume of the lungs during inspiration, rather than inspiratory gasps. Deep breathing: The signals become stronger more quickly. The rate of increase of the ramp signal is faster. Faster breathing: The signals start and cease earlier. The ramps are less than 2 seconds. Respiratory Centres of the Pons: Apneustic centres and Pneumotaxic centres of the pons adjust the output of DRG and VRG. Their activities regulate the respiratory rate and depth of respiration in response to stimuli or other centres in the brain. Stimuli: Impulses from receptors around the body are carried via the vagus or glossopharyngeal nerves to the respiratory centres. Other centres: These include the hypothalamus (deviation in temperature) or the cerebrum. Apneustic centres Located in the lower pons. The Apenuestic centre provides continuous stimulation to the DRG, resulting in a long, deep inhalation. The continuous stimulation builds the ramp signal during quiet inspiration. It coordinates transition between inhalation and exhalation. Under normal conditions, after the 2 seconds, the apneustic centre is inhibited by the pneumotaxic centre on the same side. Pneumotaxic centres Located in the upper pons. This inhibits the aponeustic centre. It controls the “switch-off” point of the ramp signal, thus limiting inspiration. Centres in the hypothalamus and cerebrum can alter the activity of the pneumotaxic centres, as well as the respiratory rate and depth. Respiratory Reflexes: The activities of the respiratory centres are modified by sensory information from several sources: Chemoreceptors: sensitive to PCO2, pH or PO2 of the blood or cerebrospinal fluid. Baroreceptors: changes in blood pressure. Stretch receptors: respond to changes in volume of the lungs (Hering-Breuer Reflex). Irritating physical/chemical stimuli in the nasal cavity, larynx, or bronchial tree. Other sensations: pain, changes in body temperature, and abnormal visceral sensations. Information from these receptors alters the pattern of respiration. The induced changes are called respiratory reflexes. Chemical Control of Respiration/ Central Chemoreceptors: Excess carbon dioxide or excess hydrogen ions in the blood mainly act directly on the respiratory centre itself, causing greatly increased strength of both the inspiratory and the expiratory motor signals to the respiratory muscles. Oxygen in contrast acts almost entirely on peripheral chemoreceptors located in the carotid and aortic bodies, and these in turn transmit appropriate nervous signals to the respiratory center for control of respiration. Direct Chemical Control of Respiratory Centre: A chemosensitive area is located bilaterally, lying beneath the ventral surface of the medulla. This area is highly sensitive to changes in either blood PCO2 or hydrogen ion concentration, and it in turn excites the other portions of the respiratory centre. Carbon dioxide has little direct effect in stimulating the neurons in the chemosensitive area. Hydrogen ions have a direct effect in stimulating the neurons in the chemosensitive area. However, hydrogen ions cannot pass through the blood-brain barrier. Carbon dioxide can cross the blood-brain barrier. Carbon dioxide crosses the barrier and reacts with the water of the tissues to form carbonic acid, which dissociates into hydrogen and bicarbonate ions; the hydrogen ions then have a direct stimulatory effect on the chemosensitive area in the brain. Therefore, more hydrogen ions are released into the respiratory chemosensitive sensory area of the medulla when the blood carbon dioxide concentration increases than when the blood hydrogen ion concentration increases. For this reason, respiratory centre activity is increased very strongly by changes in blood carbon dioxide. Regulation of Respiration during Exercise: In strenuous exercise the arterial PCO2, pH and PO2 remain almost exactly normal. Therefore, the increased ventilation doesn’t occur as a result of alterations in the arterial PCO2, pH or PO2. It is likely that most of the increase in respiration results from neurogenic signals transmitted directly into the brain stem respiratory centre at the same time that signals go to the body muscles to cause muscle contraction. The onset of exercise, the alveolar ventilation increases instantaneously, without an initial increase in arterial Pco2. In fact, this increase in ventilation is usually great enough so that at first it actually decreases arterial Pco2 below normal. The presumed reason that the ventilation forges ahead of the build-up of blood carbon dioxide is that the brain provides an “anticipatory” stimulation of respiration at the onset of exercise, causing extra alveolar ventilation even before it is needed. Peripheral Chemoreceptors: Located outside the brain. Important for detecting changes in oxygen levels in the blood. Transmit nervous signals to the respiratory centres in the brain. Carotid bodies: found in the carotid arteries. Their nerve fibres pass through the glossopharyngeal nerves and then to the dorsal respiratory area. Aortic bodies: found in the arch of the aorta. Their nerve fibres pass through the vagi, also to the dorsal respiratory area. Baroreceptors: Blood pressure – The carotid and aortic baroreceptors that detect changes in blood pressure have a small effect on respiration. A sudden rise in blood pressure decreases the rate of respiration, and a drop in blood pressure increase the respiratory rate. Stretch Receptors (Hering-Breuer Reflex): Located in the muscular portions of the walls of the bronchi and bronchioles throughout the lungs are stretch receptors that transmit signals through the vagus nerve into the dorsal respiratory group of neurons when the lungs become over stretched. When the lungs become overly inflated, the stretch receptors activate an appropriate feedback response that “switches off” the inspiratory ramp and thus stops further inspiration. This is called the Hering- Breuer inflation reflex. This reflex also increases the rate of respiration. Local Regulation of Gas Transport and Alveolar Function: In normal tissues, if the tissue becomes more active Po2 falls and Pco2 rises. The change in partial pressures causes more oxygen to be delivered. The rising Pco2 relaxes smooth muscle in walls of arteries and capillaries, causing vasodilation and so increasing blood flow. In alveolar capillaries, blood is directed where Po2 is relatively high, this occurs because alveolar capillaries constrict when local Po2 is low. In bronchioles, oxygen is directed towards lobules where Pco2 is high, because these lobules are actively engaged in gas exchange. This occurs because smooth muscle in the bronchioles bronchodilate when Pco2 is high. Hypoxia Hypoxia means a lack of oxygen. Causes of hypoxia: Inadequate oxygenation of the blood in the lungs because of extrinsic reasons Deficiency of oxygen in the atmosphere Hypoventilation (neuromuscular disorders) Pulmonary disease Hypoventilation caused by increased airway resistance or decreased pulmonary compliance Abnormal alveolar ventilation – perfusion ratio Diminished respiratory membrane diffusion Venous-to-arterial shunts (“right-to-left cardiac shunts”) Inadequate oxygen transport to the tissues by the blood Anaemia or abnormal haemoglobin General circulatory deficiency Localized circulatory deficiency (peripheral, cerebral, coronary vessels etc) Tissue oedema Inadequate tissue capability of using oxygen Poisoning of cellular oxidation enzymes (cyanide poisoning) Diminished cellular metabolic capacity for using oxygen, because of toxicity, vitamin deficiency, or other factors Effects of Hypoxia on the Body: Acute effects: Drowsiness Lassitude (lack of energy) Mental and muscle fatigue Headache, nausea One of the most important effects of hypoxia is decreased mental proficiency, which decreases judgment, memory, and performance of discrete motor movements. Cyanosis: this means blueness of the skin. Caused as a result of excessive amounts of deoxygenated haemoglobin in the skin blood vessels. This deoxygenated blood has an intense dark blue-purple colour that is transmitted through the skin. Changes in Pleural Pressures Anatomy: There are no attachments between the lung and the walls of the chest cage, except where it is suspended at its hilum from the mediastinum. The lung “floats” in the thoracic cavity, surrounded by a thin layer of pleural fluid that lubricates movement of the lungs within the cavity. Furthermore, continual suction of excess fluid into lymphatic channels maintains a slight suction between the visceral pleural surface of the lung and the parietal pleural surface of the thoracic cavity. Therefore, the lungs are held to the thoracic wall by surface tension as if glued there, except that they are well lubricated and can slide freely as the chest expands and contracts. Pleural Pressure: Pleural pressure is the pressure of the ‘fluid’ in pleural cavity (space between the visceral and parietal pleurae). Normally it is a slightly negative pressure. The normal pleural pressure at the beginning of inspiration = –5 centimetres of water (cm H2O) (-5 cm H2O = -3.68 mmHg). This is the amount of suction required to hold the lungs open to their resting level. Then, during normal inspiration, expansion of the chest cage pulls outward on the lungs with greater force and creates more negative pressure, to around –7.5 cm H2O (= -5.5mmHg). Alveolar Pressure: Alveolar pressure is the pressure of the ‘air’ inside the lung alveoli. When the glottis is open and no air is flowing into or out of the lungs, the pressures in all parts of the respiratory tree, all the way to the alveoli, are equal to atmospheric pressure, which is considered to be zero reference pressure in the airways—that is, 0 cmH2O. To cause inward flow of air into the alveoli during inspiration, the pressure in the alveoli must fall to a value slightly below atmospheric pressure (below 0). The second curve (labelled “alveolar pressure”) demonstrates that during normal inspiration, alveolar pressure decreases to about –1 cm H2O. This slight negative pressure is enough to pull 0.5 liter of air into the lungs in the 2 seconds required for normal quiet inspiration. During normal expiration, opposite pressures occur: The alveolar pressure rises to about +1 cm H2O, and this forces the 0.5 litre of inspired air out of the lungs during the 2 to 3 seconds of expiration. Transpulmonary Pressure/ Transpleural Pressure : This is the difference between the alveolar pressure and the pleural pressure. It is also a measure of the elastic forces in the lungs that tend to collapse the lungs at each instant of respiration, called the recoil pressure. Work of Breathing Depends on: Tidal volume: increased tidal volume = more work done by the lungs Respiratory frequency: increased frequency = more work done by the lungs Lung compliance: increased compliance = less work done by the lungs Airways resistance: increased resistance = more work done by the lungs Increased work of breathing, leading to fatigue, is one cause of respiratory failure. Airways Resistance $\mathbf{Airflow = \ }\frac{\mathbf{\mathrm{\Delta}P}}{\mathbf{\text{Airways\ \ Resistance}}}$ ΔP= Palv− Patm $\mathbf{Airways\ Resistance\ \propto \ }\frac{\mathbf{\text{length}}}{\mathbf{\text{radius}}^{\mathbf{4}}}$ The longer the airway, the greater the airways resistance. The narrower the airway, the greater the airways resistance. Compliance Compliance: indication of a lung’s expandability, or how easily the lungs expand and contract. The lower the compliance, the greater the force required to fill and empty the lungs. The greater the compliance, the easier it is to fill and empty the lungs. Factors affecting compliance include the following: Elastic forces of the lung tissue Elastic forces caused by surface tension of the fluid that lines the inside walls of the alveoli and other lung air spaces. Surfactant: This is a surface active agent in water, which means that it greatly reduces the surface tension of water. It is secreted by special surfactant-secreting epithelial cells called type II alveolar epithelial cells, which constitute about 10% of the surface area of the alveoli. Surfactant is a complex mixture of several phospholipids, proteins, and ions. Dead Space The amount of ‘fresh air’ reaching the alveoli/ gas exchange areas of the lung (alveolar ventilation) is less than the amount of fresh air entering the airways at the mouth and nose (pulmonary ventilation). Alveolar ventilation will vary with breathing pattern For the same amount of pulmonary ventilation, slow deep breathing gives more alveolar ventilation than fast rapid breathing (see example below). Alveolar ventilation is important for gas exchange, and so the composition of arterial blood. Pneumothorax A pneumothorax is a collection of air between the visceral and parietal pleura causing a real rather than potential pleural space. Under normal conditions, the pressure within the intrapleural space is negative with respect to the atmosphere and with respect to alveolar gas. If any connection is made with atmospheric pressure and the pleural cavity, gas will flow into the intrapleural space, increasing its pressure to atmospheric pressure. Lung partially collapses due to the elastic recoil pressure. Clinical presentation: sudden onset of unilateral pleuritic pain or progressively increasing breathlessness. If the pneumothorax enlarges, the patient becomes more breathless and may develop pallor and tachycardia. There are 4 types of pneumothoraxes: Primary Spontaneous Pneumothorax Most common type of pneumothorax. Caused by rupture of a small subpleural emphysematous bulla or pulmonary bleb (usually apical); thought to be due to congenital defects in the connective tissue. This is a weakness and out-pouching of the lung tissue, which can rupture. This introduces air into the pleural space, causing the lung to start collapsing. As the lung collapses, the hole formed by the ruptured bleb seals, preventing more air from entering the intrapleural cavity. Tall, thin, young men (20-40 years old) with no underlying disease usually affected. In patients over 40 years, the usual cause is underlying COPD. Male/female ratio = 5:1 Both lungs are affected with equal frequency. Rarely causes significant physiological disturbance. People who have had one spontaneous pneumothorax are at higher risk of recurrence. Risk factors: smoking, tall stature and presence of apical subpleural blebs. Treatment: Pleurodesis (needle aspiration and chest drain) to fuse visceral and parietal pluera by medical (bleomycin/talc) or surgical (abrasion of pleural lining). Secondary Spontaneous Pneumothorax Much more deadly. Caused by respiratory diseases that damage lung structure: most commonly COPD and asthma, fibrotic or infective diseases (pneumonia), rarely inherited diseases (eg. Marfan’s and cystic fibrosis). Incidence increases with age (peaks in males aged 15-30 years) and severity of underlying disease. Usually occurs in males >55 years. ICU patients with lung disease are at particular risk for SSP due to high presses (barotrauma) and alveolar distention (volutrauma) associated with mechanical ventilation. Protective ventilation strategies using low pressure, limited volume ventilation reduce this risk. Traumatic Pneumothorax Blunt (road traffic accident) or penetrating (stab wounds, fractured ribs) chest trauma. Gas may enter the intrapleural space either from the atmosphere through a hole in the chest wall or from alveoli through a hole in the lung. The flow of air two way. Therapeutic procedures such as line insertion or thoracic surgery are common causes. Traumatic Pneumothorax can usually be associated with haemothorax. Tension Pneumothorax Most common during mechanical ventilation/following traumatic pneumothorax. The flow of air is one way (from lung into the pleural cavity) upon inspiration. Upon inspiration, the air from the atmosphere enters the pleural cavity (from the stab wound) down the pressure gradient. Upon expiration, the air can’t escape from the pleural cavity and remains trapped there because the pleural pressure doesn’t increase above the atmospheric pressure. Every inspiration results in a buildup of air and pressure (tension). Specific symptoms: cyanosis, severe breathlessness. Treatment: needle aspiration and chest drain. Clinical Features of Pneumothorax A spontaneous pneumothorax will present with chest pain and breathlessness. The pain is usually of sudden onset, localized to the affected side and made worse on inspiration. Dyspnoea (labored breathing) is partly produced by the difficulty in taking a deep breath, but is also dependent on the size of the pneumothorax and the presence of underlying lung disease. The most consistent finding is a reduction in breath sounds on the affected side. Movement of the chest wall may be reduced. The percussion note will be resonant. Diagnosis Examination of the chest with a stethoscope reveals decreased or absent breath sounds over the affected lung. The diagnosis is confirmed by chest x-ray. An x-ray can illustrate the collapse of the lung as extra black space, indicating the presence of air, will be seen in the x-ray around the lung. In tension pneumothorax, the lung shrivels up away from the affected side and the mediastinum (trachea and other components) will shift towards the unaffected side – trachea displacement. The combination of absent breath sounds and resonant percussion is diagnostic of pneumothorax. Physical Examinations Inspection: Patient looks distressed and is sweating. Dyspnoea (SOB) may be apparent. Cyanosis, depending on degree of respiratory inadequacy. Palpation: Palpation may show affected side moves less than normal side. (air in intrapleural space allows ribcage to expand outwards) Percussion: Affected side sounds hyperresonant (sound similar to percussion of puffed cheeks). Auscultation: Breath sounds on the affected side are diminished. This is because less gas enters the collapsed lung during inspiration, air in intrapleural space acts as a barrier to the transmission of sounds from lungs to the chest wall. Trachea displaced away from side of collapsed lung in tension pneumonia. Pulse Examination Tachycardia common, pulse rate >135 beats per minute suggests tension pneumothorax. Pulsus paradoxicus (slow pulse on inspiration) suggests severe pneumothorax Monitoring reveals hypotension and desaturation (less oxygen being carried in the blood) Investigations Chest X-Ray - confirms the diagnosis. Clear line of visceral pleura with absence of peripheral lung markings beyond it Trachea and mediastinum may also be deviated away from affected side Standard erect posterior anterior films are usually adequate. A: Airways; B: Breathing and Bones; C: Cardiac; D: Diaphragm; E: Everything else Arterial Blood gases will show hypoxia (insufficient oxygen supply to body). More disturbed in SP - less reserve in the presence of pre-existing lung disease. CT scan not used in routine, but may be helpful to differentiate a large bulla from a pnuemothorax, may detect localised pnuemothoraces. Treatment Pneumothorax is graded depending on the degree of lung collapse, and treatment is administered accordingly. Immediate management involves supplemental oxygen to reduce hypoxia. A tension pnuemothorax must be drained immediately. A small PSP can be managed by observation using clinical assessment and Chest X-ray. PSP >30% aspirated in the second intercostal space in the midclavicular line, using a 15 mL syringe connected to a 3-way tap and underwater seal. Used to suck out as much air as possible, if patient coughs, this is achieved. SP and Traumatic pneumothoraces always require hospital admission and intercostal chest drainage. Multiple may be needed. Chest Drain Consists of a length of plastic tubing inserted into the intrapleural space through a small incision made under local anaesthetic between two ribs. Allows air to escape from the intrapleural space so that the underlying lung can re-inflate. A one way valve is placed at the end of the drain, so that the CD removes air from intrapleural space rather than allowing more air to enter it. Usually in the form of an underwater seal. An underwater seal consists of a container of water with a tube extending below the surface of water. Gas can pass from the tube into the atmosphere by forming bubbles in the water, but no gas can pass back up the tube and the water cast as a one-way valve at the end of the tube. As pressure in intrapleural space becomes positive (during coughing/forced expiration), air passes along chest drain and out to the atmosphere through the underwater seal, pneumothorax is drained. Bubbles appear in the underwater seal until the hole in the pleura has sealed. After the hole has healed, the water level will rise and fall slowly and the chest drain can be removed. Underwater seal also collects blood and other secretions that pass along the chest drain. ATLS Protocol Advanced trauma life support is the standard method for the initial management of severely injured patients. The principle is simple - treat the greatest threat to life first. Loss of airway will kill before inability to breathe, and inability to breathe will kill before bleeding and loss of circulation. A definitive diagnosis is not necessary to treat the patient initially. The most important point to remember is that no harm should be done to the patient during treatment. The management of severely injured patients is divided into the primary and secondary survey: Primary Survey During this survey, life-threatening injuries are identified and resuscitation is begun. A simple mnemonic, ABCDE, is used as a memory aid for the order in which problems should be addressed: Airway Maintenance with Cervical Spine Protection ensure airway is clear and patient can breathe neck and cervical spine must be maintained in the neutral position to prevent secondary injuries to the spinal cord Breathing and Ventilation chest examined by inspection, palpation, percussion and auscultation life-threatening injury like tension pneumothorax must be identified Circulation with Haemorrhage Control external bleeding must be controlled internal bleeding must be diagnosed Disability and Neurologic status neurological assessment is made Exposure and Environment patient should be completely undressed, usually by cutting off the garments privacy maintained cover the patient with warm blankets to prevent hypothermia intravenous fluids should be warmed and a warm environment maintained Secondary Survey This begins when the primary survey is completed, resuscitation efforts are well established, and the vital signs are normalizing. It is a head-to-toe evaluation of the trauma patient, including a complete history and physical examination, including the reassessment of all vital signs - each region of the body must be fully examined. If at any time during the secondary survey the patient deteriorates, another primary survey is carried out as a potential life threat may be present. The person should be removed from the hard spine board and placed on a firm mattress as soon as feasible, as the spine board can rapidly cause skin breakdown and pain while a firm mattress provides equivalent stability for potential spinal fractures. Autonomic Nervous System The autonomic nervous system has two subdivisions: The sympathetic nervous system (SNS). The parasympathetic nervous system (PNS). The sympathetic and parasympathetic nerve fibers secrete mainly one of the following; Acetylcholine - Those fibers are said to be cholinergic Norepinephrine - Those fibers are said to be adrenergic All preganglionic neurons are cholinergic. In the postganglionic system; Most of the sympathetic are adrenergic Almost all parasympathetic are cholinergic Acetylcholine Receptors Acetylcholine activates mainly two types of receptors: Nicotinic receptors - found in the autonomic ganglia at the synapses between the preganglionic and postganglionic neurons of both the sympathetic and parasympathetic systems Muscarinic receptors - found on all effector cells that are stimulated by the postganglionic cholinergic neurons of the parasympathetic nervous system. Classification of Adrenergic Receptors There are also two major types of adrenergic receptors: Alpha receptors Alpha 1: Found in the walls of blood vessels. Upon activation, cause smooth muscle contraction. Vasoconstriction and vasodilation are controlled by the SNS. Stimulation of SNS causes vasoconstriction. Less stimulation of SNS causes vasodilation. Alpha 2: Inhibits adenylate cyclase – decreasing cAMP formation. Negative feedback for the release of norepinephrine from the presynaptic neuron. Inhibition of insulin release and induction of glucagon release in the pancreas. Beta receptors All beta receptors stimulate adenylate cyclase Beta 1: Located mainly in the heart - Increase cardiac output Beta 2: Found mainly in the lungs – Bronchodilation. Beta 3: Located in fat cells - Lipolysis in adipose tissue. Both alpha and beta receptors are G-protein coupled receptors (intracellular messengers). Norepinephrine excites mainly alpha receptors and beta receptors to a lesser extent Epinephrine excites both types of receptors equally Stress Response Physiological Response In some instances, almost all portions of the sympathetic nervous system discharge simultaneously producing an effect known as mass discharge. This frequently happens when the hypothalamus is activated by fright, fear or severe pain. The result leads to the stress response. The sympathetic nervous system has small preganglionic nerves and long postganglionic nerves. The preganglionic nerves are in close proximity (thoracic and lumbar regions).They ‘talk’ to each other. When the SNS is activated, all of the preganglionic nerves are activated simultaneously. Any condition-physical or emotional-that threatens homeostasis is a form of stress. Upon exposure to a wide variety of stress-causing factor, the body undergoes the stress response; this can be divided into three phases: The Alarm Phase The Resistance Phase The Exhaustion Phase. The Alarm Phase During the alarm phase, an immediate response to the stress occurs. This response is directed by the sympathetic nervous system. Activation of the SNS causes increased secretion of adrenaline. Adrenaline is the dominant hormone of the alarm phase, and its secretion accompanies a generalized sympathetic activation. In the alarm phase: Energy reserves are mobilized, mainly in the form of glucose The body prepares to deal with the stress-causing factor by “fight or flight” responses The characteristics of the alarm phase include the following: Increased mental alertness. Increased energy use by all cells, especially skeletal muscles. Mobilization of glycogen (Hepatocytes perform glycogenolysis) and lipid reserves (adipose tissue cells perform lipolysis). Changes in circulation, including increased blood flow to skeletal muscles and decreased blood flow to the skin, kidneys, and digestive organs. A drastic reduction in digestion and urine production. Increased sweat gland secretion. Increases in blood pressure, heart rate, respiratory rate and metabolic rate. Although the effects of adrenaline are most apparent during the alarm phase, other hormones play supporting roles. In this phase, there is increased production of adrenaline and noradrenaline so that both the alpha and beta adrenergic receptors are activated with better efficiency. For example, the reduction of water losses resulting from ADH production and aldosterone secretion can be very important if the stress involves a loss of blood. The Resistance Phase If a stress lasts longer than a few hours, the individual enters the resistance phase of the stress response. Glucocorticoids (cortisol) are the dominant hormones of the resistance phase. Growth Hormone, ADH and glucagon are also involved. Energy demands in the resistance phase remain higher than normal. Neural tissue has a high demand for energy, and neurons must have a reliable supply of glucose. If blood glucose concentrations fall too far, neural function deteriorates. Glycogen reserves are adequate to maintain normal glucose concentrations during the alarm phase, but are nearly exhausted after several hours. Therefore, alternate energy sources are sought for in the resistance phase. The endocrine secretions of the resistance phase achieve four integrated results: Mobilization of remaining lipid and protein reserves: The hypothalamus produces GHRH, stimulating the release of GH and glucocorticoids. Adipose tissue responds to GH and glucocorticoids by releasing stored fatty acids. Skeletal muscles respond to glucocorticoids by breaking down proteins and releasing amino acids into the bloodstream. Conservation of glucose for neural tissues: Glucocorticoids (cortisol) and GH stimulate lipid metabolism in peripheral tissues. Peripheral tissue (except neural) breaks down lipids to obtain energy. Neural tissues do not alter their metabolic activities, however, and they continue to use glucose as an energy source. Elevation and stabilization of blood glucose concentrations: As blood glucose levels decline, glucagon and glucocorticoids (cortisol) stimulate the liver to manufacture glucose from other carbohydrates (glycerol) and from amino acids provided by skeletal muscles. Conservation of salts and water, and the loss of K+and H+: Blood volume is conserved through the actions of ADH and aldosterone. As Na+ is conserved, K+ and H+ are lost. The resistance phase cannot be sustained indefinitely. If starvation is the primary stress, the resistance phase ends when lipid reserves are exhausted and structural proteins become the primary energy source. If another factor is the cause, the resistance phase ends due to complications brought about by hormonal side effects. There may be side-effects experienced as a result of the hormones: Glucocorticoids (cortisol): anti-inflammatory action slows wound healing and increases the body’s susceptibility to infection. The continued conservation of fluids under the influence of ADH and aldosterone stresses the cardiovascular system by producing elevated blood volumes and higher-than-normal blood pressures. The suprarenal cortex may become unable to continue producing glucocorticoids, eliminating acceptable blood glucose concentrations. The Exhaustion Phase When the resistance phase ends, homeostatic regulation breaks down and the exhaustion phase begins. Unless corrective actions are taken almost immediately, the failure of one or more organ systems will prove fatal. Mineral imbalances contribute to the existing problems with major systems. The production of aldosterone throughout the resistance phase results in a conservation of Na+ at the expense of K+. As the body’s K+ content declines, a variety of cells—notably neurons and muscle fibers—begin to malfunction. Although a single cause (such as heart failure) may be listed as the cause of death, the underlying problem is the inability to sustain the endocrine and metabolic adjustments of the resistance phase. Stress occurs when the perceived demands of a situation are appraised as exceeding a person’s perceived resources and ability to cope These situations are stressors and lead to the stress response The more control we perceive we have over a situation the less stressful it is If the stressor can be predicted, its effect is lessened Appraisal is central to whether a person feels stressed or not Severe or chronic stress is associated with psychological problems Severe or chronic stress is associated with a range of illness and mortality Psychological Response The psychological appraisal of a stressor is central to the stress response; Without appraisal physiological changes are absent or minimal. The degree of appraisal also influences the extent of the physiological response. It is likely that the mind–body interactions illustrated by stress are dynamic and ongoing. Therefore, rather than appraisal causing a change in physiology which constitutes the response, appraisal probably triggers a change in physiology which is then detected and appraised causing a further response and so on. In addition, psychological factors such as control, personality, coping and social support will impact upon this ongoing process. Approach coping strategies try to deal with the situation pro-actively and thus share some overlap with problem-focused strategies. Avoidant coping strategies try to avoid the problem Stress occurs when the perceived demands of a situation are appraised as exceeding a person’s perceived resources and ability to cope. These situations are stressors and lead to the stress response The more control we perceive we have over a situation the less stressful it is If the stressor can be predicted, its effect is lessened Appraisal is central to weather a person feels stressed or not Severe or chronic stress is associated with psychological problems Severe or chronic stress is associated with a range of illness and mortality Males tend to respond to stress with increased levels of cortisol relating to ah higher chance of the fight-or-flight behavior in males. In females, they discovered that instead of increased cortisol levels, an increase in the activity of the limbic system was initiated. Females’ increased limbic activity gave proof to the tend-and-befriend theory for female coping strategies and says that females are more likely to alleviate stressful situations by nurturing and running to acceptable social groups Stress Reactivity Changes in physiology are known as ‘stress reactivity’ and vary enormously between people. For example, some individuals respond to stressful events with high levels of sweating, raised blood pressure and heart rate while others show only a minimal response. research also shows that some people are simply more reactive to stress than others. Stress Recovery After reacting to stress, the body then recovers and levels of sympathetic activation return to baseline. However, there is great variability in the rate of recovery both between individuals, as some people recover more quickly than others, and within the same individual across the lifespan. Allostatic Load Stress recovery is linked with allostatic load. The body’s physiological systems constantly fluctuate as the individual responds and recovers from stress – a state of allostasis – and that, as time progresses, recovery is less and less complete and the body is left increasingly depleted. Stress Stress as a STIMULUS Stress can act as interactions/ pressures to elicit a stress response. Example: Lazarus’ Model of Appraisal The Transaction Model of Stress The Role of Appraisal (Model of Appraisal) Lazarus argued that stress involved a transaction between the individual and their external world, and that a stress response was elicited if the individual appraised a potentially stressful event as actually being stressful. Lazarus’s “model of stress”: Described individuals as psychological beings who appraised the outside world, not simply passively responding to it. Lazarus defined two forms of appraisal: primary and secondary. Primary appraisal: The individual initially appraises the event itself. There are four possible ways that the event can be appraised: Irrelevant Benign (gentle) and Positive Harmful and a Threat Harmful and a Challenge Secondary appraisal: The individual evaluating the pros and cons of their different coping strategies. Therefore primary appraisal involves an appraisal of the outside world and secondary appraisal involves an appraisal of the individual themselves. The form of the primary and secondary appraisals determines whether the individual shows a stress response or not. This stress response can take different forms: Direct action Seeking information Doing nothing Developing a means of coping with the stress in terms of relaxation or defense mechanisms Lazarus’s model of appraisal and the transaction between the individual and the environment indicated a novel way of looking at the stress response – the individual no longer passively responded to their external world, but interacted with it. It is not an event itself that elicits stress, but the individual’s interpretation or appraisal of those events. This appraisal can be modified by providing information or withholding information from the individual. An event needs to be appraised as stressful before it can elicit a stress response. It could be concluded from this that the nature of the event itself is irrelevant – it is all down to the individual’s own perception. Multitasking seems to result in more stress than the chance to focus on fewer tasks at any one time. Therefore a single stressor which adds to a background of other stressors will be apprised as more stressful than when the same stressor occurs in isolation. If a stressor can be predicted and controlled then it is usually appraised as less stressful than a more random uncontrollable event. A feeling of being in control reduces the stress of an event and contributes to the process of primary appraisal. Stress as a RESPONSE Cannon’s Fight/ Flight Response Acute: Increased sympathetic activation Increased cognitive performance Increased muscular priming Increased immune functioning Chronic: Decreased immune functioning Decreased cognitive performance This eventually leads to exhaustion. Hans Selye’s General Adaption Syndrome (GAS) This is broken down into three phases (see previous notes): Alarm Phase Shock (initial symptoms) Flight-or-Fight response (sympathetic nervous system activated) Hormones such as cortisol and adrenaline (epinephrine) released. Counter-shock (homeostasis) Resistance Phase Adaption Parasympathetic nervous system returns many physiological functions back to normal levels while body focuses resources against the stressor. Blood glucose levels remain high (cortisol and adrenaline continue to circulate at elevated levels). Increased heart rate, breathing and blood pressure. Exhaustion Phase Symptoms reappear If stressor continues beyond body’s capacity, organism exhausts resources and becomes susceptible to diseases and death. Effects of Anaesthesia and Analgesia Sodium Channels Sodium channels exist in three distinct functional states: Resting Open Refractory/ Inactive Depolarisation: Channels switch rapidly from the resting state to the open state – ‘activation’. Repolarization: Channels switch rapidly from the open state to the refractory/inactive state – ‘inactivation’. Lidocaine Lidocaine is an anaesthetic (blocks sensation) with minor analgesic (pain killer) properties. Lidocaine is a Class Ib drug. Class I drugs: block voltage-sensitive (voltage-gated) sodium channels; this blockage causes a loss of sensation and eliminates the responsiveness of these cells to pain. bind to the sodium channels most strongly when they are in either the open or the refractory/inactive state, less strongly to channels in the resting state. Their action therefore shows the property of 'use dependence' (i.e. the more frequently the channels are activated, the greater the degree of block produced). Class I agents can be subdivided: Ia: lengthen the action potential (bind to sodium channel in the open or inactive/refractory states (open state binding > refractory/inactive state binding)) Ib: shorten the action potential (bind to sodium channel in the open or inactive/refractory states (refractory/inactive state binding >> open state binding)) Ic: no significant effect on the action potential (bind to sodium channel in the open state) Lidocaine is a Class Ib drug: It has a fast onset. It counters kinetics – it has little or no effect at slower heart rates, and more effects at faster heart rates Shorten the action potential duration. Decrease refractoriness. Lidocaine concentration administered is usually too low for it to effect on motor neurons in the area. Uses: Given by intravenous infusion to treat and prevent ventricular dysrhythmias in the immediate aftermath of a myocardial infarction. Also used as a local anaesthetic (analgesic) for minor surgery. Widely used for local anaesthesia (analgesic) (for needle aspirations/ chest drains). 2 hour half-life, increased by reducing hepatic blood flow (97% clearance in 10 hours). Careful titration allows for a high degree of selectivity in the blockage of sensory neurons, whereas higher concentrations will also affect other modalities of neuron signaling. Side-effects – drowsiness, disorientation, convulsions, bradycardia, decreased cardiac output, vasodilation. Post-Traumatic Stress Disorder (PTSD) People who live through events that are beyond the normal range of human suffering sometimes develop a severe set of anxiety-related symptoms known as post-traumatic stress disorder (PTSD). PTSD is a condition where exposure to an intense and frightening emotional experience leads to lasting changes in behaviour, mood and cognition. The major symptoms are: Feeling numb to the world with a lack of interest in former activities and a sense of estrangement from others Reliving the trauma repeatedly Sleep disturbances Difficulty concentrating Over alertness The major PTSD categories are: Category A: Exposure to event involving actual/threatened death or serious injury or threat to physical integrity. Category B: 1+ symptom of reliving the trauma repeatedly. Category C: 3+ symptoms of persistent avoidance of trauma stimuli or numbing of general responsiveness. Category D: 2+ symptoms of persistent increased arousal. Diagnosis: Duration of symptoms = ≥1 month. Clinically significant distress or impairment in functioning. Acute = symptoms of 1 to 3 months. Chronic = symptoms duration of 3 months or more. Delayed onset = onset of symptoms at least 6 months after stressor Compulsory psychological debriefing immediately after a trauma does not prevent PTSD and may be harmful. Prevention is better achieved by the support offered by others who were also involved. Trauma focused CBT is often effective. Cognitive Behavioural Therapy (CBT) The primary goal of CBT for PTSD is to undergo some sustained emotional processing of the traumatic experiences. Exposure (relearning response) Acclimatization of anxiety through exposure to trauma related stimuli. Anxiety management Learning how to cope. Cognitive therapy Modification of thinking styles. CBT is a structured therapy that focuses on clearly identified and achievable treatment goals. CBT for PTSD is designed to desensitise the person to the traumatic event. CBT techniques are used to reprocess the feared event and improve their strategies to decrease the sense of threat. The aim of CBT is to modify unhelpful and maladaptive beliefs and to generate more flexible, rational and adaptive beliefs. CBT emphasizes that the way we think about situations (ourselves, others, our world, future) influences our mood and behaviours. Behavioural therapy involves changing maladaptive behavioural responses (behaviors that inhibit a person’s ability to adjust to particular situations) and substituting them with new responses. Behavioural techniques include monitoring behaviour through activity charts, rehearsing positive behavioural responses and using goal-setting to encourage/reinforce new behaviours. Apathy of Bystanders The bystander effect is a psychological phenomenon in which someone is less likely to intervene in an emergency situation when other people are present. If someone defines an ambiguous situation as non-emergency, this can lead to others around the person defining the situation as non-emergency. This develops a state of pluralistic ignorance. That is, everybody in the group misleads everybody else by defining the situation as non-emergency. But, it is Diffusion of responsibility, which causes a person to not react in a clear cut emergency. When each individual knows that many others are present, the burden of responsibility does not simply fall on him/her. People may also assume that other bystanders may be more qualified to help, and their intervention would thus be unneeded. To counter the bystander effect: When you are the victim, pick a specific person in the crowd to appeal to for help rather than appealing to the larger group generally. If you are the only person reacting to an emergency, point directly to a specific bystander and give them a specific task such as, "You. Call the police." These steps place all responsibility on a specific person instead of allowing it to diffuse. This counters pluralistic ignorance. Factors affecting the likelihood of receiving help: Situational ambiguity - In ambiguous situations, people are much less likely to offer assistance than in situations involving a clear-cut emergency. They are also less likely to help in unfamiliar environments than in familiar. Perceived cost - The likelihood of helping increases as the perceived cost to ourselves declines. Similarity - People are more willing to help others whom they perceive to be similar to themselves Mood - People are generally more willing to help others when they are in a good mood. Gender - Women in need are more likely than men in need to receive assistance from strangers. Attributions of the cause of need - People are much more likely to help others they judge to be innocent victim. Thus, they may fail to lend assistance to homeless people and drug addicts. Social norms - Social norms prescribe behaviours that are expected of people in social situations. The social norm of "doing your part" in helping a worthy cause places a demand on people to help, especially in situations where their behaviour is observed by others. Competence - Feeling competent to deal with an emergency makes it more likely that help will be given. Life Events Theory The life events theory was developed to examine stress and stress-related changes as a response to life experiences. A schedule of recent experiences (SRE) was developed, which provided respondents with an extensive list of possible life changes or life events. This list ranged from life threatening experiences to simple experiences. (Holmes and Rahe Scale) Originally the SRE was scored by simply counting the number of actual recent experiences. This crude method was replaced to include a weighting system so that death of a spouse has more value than change in eating habit. This created a degree of differentiation between the different life events. Problems with life events theory The use of the SRE and similar measures of life experiences have been criticized for the following reasons: The individual’s own rating of the event is important – A muscle injury is much more stressful for an athlete than an office worker. The problem of retrospective assessment - The present state of mind will influence their recollection of the stress. Life experiences may interact with each other – This can lead to stresses countering each other or compounding the problem. What is the outcome of a series of life experiences? Stressors may be short term or on-going – This leads to the reaction of the life stressors and social resources inventory (LISRES) – which represented an attempt to emphasize the chronic nature of life experiences and to place them within the context of the individual’s coping resources.