Archaea Biology PDF
Document Details
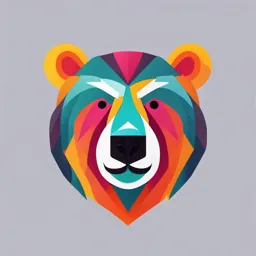
Uploaded by CompatibleAntigorite7412
University of Brighton
Tags
Summary
This document provides a lecture on archaea biology, including learning outcomes, introduction, basic cell biology, cellular organization, examples, and physiological functions. It explores the adaptations of archaea to extreme environments and the structural differences between archaea and other microorganisms. The document also covers specific adaptations, such as acid tolerance, temperature tolerance, pressure tolerance, and high salt tolerance. The summary also includes notes on cell membranes, genetic aspects, and further reading suggestions.
Full Transcript
Archaea Learning Outcomes To understand the basic cell biology of Archaea. To understand the function of cellular features. To understand the adaptations of Archaea to their unique habitats, and learn examples of species adapted to different habitats. Introdu...
Archaea Learning Outcomes To understand the basic cell biology of Archaea. To understand the function of cellular features. To understand the adaptations of Archaea to their unique habitats, and learn examples of species adapted to different habitats. Introduction to Archaea This lecture will focus on the cell biology of Archaea, and the adaptations that allow them to survive in what we would term extreme environmental conditions. It is important to note that these are normal conditions for these organisms. Basic Cell Biology – similarities & differences between bacteria & archaea Fimbria (pl. Flagellum (pl. fimbriae) flagella) Cytoplasm Inclusion body Sex pilus Cell wall Chromosome Ribosomes Plasmid Capsule Plasma (nucleoid) membrane Cellular organisation of Archaea? & Eubacteria (Bacteria)? Example species: Halobacterium walsbyi Numerous species Example archaea & bacteria species Methanococcus Methanosarcina Methanothermus Methanococcus Methanosarcina Methanothermus janaschii barkeri fervidus http://t1.gstatic.com/images?q=tbn:fRwtI2F6vPWMsM:http://biochem.iisc.ernet.in/patrick/E.coli.jpg http://t3.gstatic.com/images?q=tbn:MlvnUZOUyb6OIM:http://upload.wikimedia.org/wikipedia/commons/5/5d/MRSA_SEM_7821_ http://t3.gstatic.com/images?q=tbn:FqvnLlPxcKrT3M:http:/ Escherichia coli Staph. aureus Bacillus anthracis Physiological functions What do each of these features do? – Flagellum? – Capsule? – Ribosome? – Fimbria? Most bacterial cell walls contain peptidoglycan, but Archaea do not. They contain pseudo-peptidoglycan. Gram negative bacteria also possess lipopolysaccharides in their cell walls, Archaea do not. Introduction to Archaeal cells Like Bacteria, the Archaea lack a membrane-enclosed nucleus and their DNA exists in a single circular form (a chromosome). However, their DNA is associated with histones, a characteristic of Eukarya, and their cell ‘machinery’ (such as protein synthesis enzymes and RNA polymerases) resemble eukaryotic ones. The lipids that comprise their membranes are unique, resembling neither the bacteria nor the eukaryotes. Archaea Archaeal physiology appears to relate to their early evolutionary history: when Earth was hot & anaerobic They are specifically adapted to live in what we call extreme environmental conditions. Archaea have cell membranes that are chemically distinct from Eubacteria and Eukaryotes: They contain branched hydrocarbons with ether links, as opposed to straight-chain fatty acids and ester links of Bacteria & Eukaryotes The three primary regions of an Archaeal cell are the cytoplasm, cell membrane, and cell wall. Archaeal cell membranes are chemically different from all other living things, including a reversed glycerol molecule (L-glycerol) and isoprene derivatives in place of fatty acids. Cell Membranes A B A = ether linkage B = ester linkage Archaea Their cell walls do not contain peptidoglycan, nor any D-amino acids: they are all L- isomers. But, they do contain pseudopeptidoglycan! Their cell wall contain proteins & glycoproteins, but using L-isomers. Those of bacteria and eukaryotes contain D-isomers. This means that the cell formation, structure and metabolism is different from that of Eubacteria and Eukarya. This has been suggested as evidence of evolutionary distance. Sulfolobus solfataricus Example archaea species: Sulfolobus solfataricus Archaeal S-Layers Notes: 1. the presence of the cytoplasmic membrane (similar to bacteria & eukaryotes) 2. presence of pseudomurein (also known as pseudopeptidoglycan) 3. presence of S-layer proteins (unique to archaea) Nature.com http://jb.asm.org/content/vol182/issue4/images/medium/jb0401610001.gif Electron micrograph of a freeze- etched preparation showing a whole cell with a hexagonally ordered S-layer lattice. Bar, 100 nm. Sára M., Sleytr U. (2000) S-Layer Proteins. Journal of Bacteriology, 182 (4): 859-868. Cell walls for bacteria & archaea Pseudopeptidoglycan is composed of alternating repeats of two sugars: – N-acetylglucosamine (bacteria also use this chemical) – N-acetyltalosaminuronic acid (unique to archaea) These are cross-linked by L-amino acids, rather than D, and the linkages are 1 3, rather than 1 4 (bacteria) They contain large amounts of polysaccharides, including glucose, galactosamine & glucuronic acid. Basic genetics of Archaea Like bacteria, they possess a singular covalently closed DNA molecule (chromosome) Archaea genomes can be up to 1900 kb pairs; ~ ½ the size of the E. coli genome! Archaea transcription processes closely resemble those of eukaryotes (e.g. using RNA polymerase), but not those of bacteria. However, the full genetic processes are not fully understood (& can be difficult to model in the lab)… Archaea – unique cell adaptations As opposed to Eubacteria & Eukaryotes, different species of Archaea can possess the following in their cell walls, depending on habitat… Co-factor M (transfer of methyl group amongst methanogen species) Factor F20 (cold shock proteins) 7-mercaptoheptanoylthreonine phosphate (methanogenesis) Tetrehydromethanopterin (instead of folate) Methanofuran (methane & CO2 metabolism in methanogens) Retinal (an archaeal rhodopsin) Metabolic diversity of micro-organisms In biological systems, ATP is used to make endergonic reactions favourable. e.g. A+B C+D (energetically unfavourable to perform this metabolic reaction) When coupled to ATP, this becomes: ATP ADP + Pi A+B C+D Proton motive force (PMF) Protons can move either due to metabolic activity or due to specialised proton pumps which derive their energy from electron transport. The resultant movement is called PMF, and it relates to an unequal distribution of charge across a membrane (e.g. in acid – H+ ions). Electron flow causes protons to move across the plasma membrane, causing the flagellum to rotate. Check! – What function does the flagellum have? What does it do…? Proton motive force (PMF) PMF is essential; it drives bacterial & archael flagella, and therefore enables motility. Typical cell sizes Organism Size Escherichia coli 1 x 3 µm (a bacillus) Bacteria Staphylococcus aureus 0.2-2 x 2-8 µm (a coccus) Methanococcus ~ 1µm maripaludis Archaea (a coccus) Methanothermus fervidus 0.4 -1-4 x 0.3 µm (a bacillus) Summary of cell structures Complete this prior to the online session Component Eubacteria Archaea Cell (plasma) membrane Peptidoglycan Flagella Glycoproteins in membrane DNA organisation Membrane glycerol Isoprene in membrane Membrane links Key learning Points Archaea & bacteria form two distinct “domains of life” Differences centre upon their: ◦ cellular structure ◦ DNA organisation ◦ DNA translation ‘machinery’ ◦ adaptation to environmental niches One key feature is the difference in composition of their cell membranes (e.g. Archaea do not possess peptidoglycan, but bacteria do) Examples of “Extreme Environments” Pointers Through the next section of this lecture, we will be looking at examples of species adapted to life at environmental extremes. Focus on learning examples of cellular adaptations to protect against: Acid tolerance Temperature tolerance Salinity tolerance Pressure tolerance And then, contrast how adaptation to one tolerance is compatible or incompatible with survival at the reverse tolerance Why do cells/ species adapt..? Adaptations are due to: selection pressures genetic change, or environmental pressures These result in the divergence of cells, leading to species that are well-adapted to a new environment, but sometimes these adaptations mean that the organism can no longer survive in other environments Acid tolerance: pH range Example species pH Minimum Optimum Maximum Escherichia coli 4.4 6-7 9.0 Enterobacter aerogenes 4.4 6-7 9.0 Pseudomonas aeruginosa 5.6 6.6-7 9.0 Erwinia cartovora 5.6 6.6-7 8.0 Nitrosomas spp. 7.0 8-8.8 9.4 Nitrobacter spp. 6.6 7.6-8.6 10.0 Thiobacillus thioxidans 1.0 2.0-2.8 6.0 Lactobacillus acidophilus 4.0 4.6-5.8 6.8 Thermoplasma 1.0 1.5 4.0 acidophilus Sulfobus acidocaldarius 1.0 2.5 4.0 Archaea thermophiles Neutrophiles & mesophiles Halophiles Acid tolerance: Protein adaptation Proteins are chemically adapted into stable isoforms to enable the cell to survive in acidic conditions. Within the membrane of thermophilic species, proteins exhibit increased inter-subunit hydrogen bonding and an increased number of arginine-containing salt bridges. The free energies of transfer of ionised amino acid side chains in water to both their ion-paired and neutral hydrogen-bonded states results in a proton transfer energy of approximately zero. Acid tolerance: membranes Cell membranes are directly adapted to tolerate external pH~2, whilst still tolerate pH~7 on the internal side! The membrane in a “normal” environment Amino acid NH2 H+ Amino acid NH2 NH3+ In acidic environments Acid tolerance: enzymes One of the key adaptations of species to survive at highly acidic (low pH) environments, is to produce membranes & proteins that have an excess negative charge. For example, endo-𝛽-glucanase from Sulfolobus solfataricus, which possesses a large number of glutamic and aspartic acid residues. It should be noted that a large number of acidophiles are also thermophiles. Therefore, acidophilc adaptations can resemble adaptations to high temperature as well. Temperature tolerance- THERMOPHILES Geothermal heat & acid-tolerance Yellowstone Park, USA Sulfolobus solfataricus (and other species) oxidise elemental sulphur to sulphates to release energy Thermophile temperature range Hyper-thermophile Thermophile growth rate Relative Mesophile Psychrotolerant Psychrophile 0 10 20 30 40 50 60 70 80 90 100 Temperature, degrees Celsius Optimal temperatures of Archael thermophile species ˚C Species 50 Methanosarcina thermophila 75 Thermococcus stetteri 83 Methanothermus fervidus 92 Staphylothermus marinus 102 Pyrococcus furiosus 105 Pyrodictium brockii High temperature adaptations High temperature adaptations include large numbers of saturated lipids in the cell membranes Enzymes are chemically altered to allow activity at elevated temperatures When growing at near maximal temperatures, some additional growth factors are required This is an example of temperature-dependant auxotrophy Large guanidine + cytosine (G+C) content in the DNA are believed to be key cellular adaptations to high temperature High temperature adaptations Thermophile membranes have many long-chain fatty acids- lots of saturated fatty acids. Membrane lipids "freeze" into solid form at lower temperatures, thus inhibiting transport. But at very high temperatures, membranes demonstrate good fluidity. Cell Membranes – reminder! A B A = ether linkage B = ester linkage Effect of temperature on membrane fatty acid composition Palmitic acid 50 (saturated) 40 % of total fatty acids 20 30 Octadecanoic acid (unsaturated) 10 0 0 10 20 30 40 50 Temperature, degrees Celsius Low temperature adaptations Psychrophiles produce enzymes with lower temperature optima. They often denature at room temperatures. Psychrophiles have higher unsaturated fatty acids plus short-chain membrane lipids: keeps membranes fluid at lower temperatures. Image result for Methanogenium frigidum Example species: Methanogenium frigidum (it is both a psychrophile, and a halophile) ijs.microbiologyresearch.org Membrane fluidity Membranes adapted to high temperatures will not survive at low temperatures This is where the membrane structure becomes important (Archaea possess ether linakges & Eubacteria posses ester links) The individual archael bilayers are held together by hydrophobic bonds. These forces plus the ether bonds and hydrocarbon branching increases the heat tolerance of the archael membranes High salt environments: HALOPHILES Halophiles exist where there is a high level of dissolved ions; consequentially this means that there is a reduced water availability for the cells to utilise for respiration NB- water availability can be influenced by agriculture; e.g. irrigation and aeration alters soil profile Water availability In hypertonic environments, non-halophiles become dehydrated In addition to affecting osmotic pressure, high salt concentrations act to denature proteins Halophiles effectively act to exclude the sodium ion at concentrations where it could become toxic. Archaea such as Halorubum sp. accumulate high intracellular concentrations of potassium ions, and potassium chloride becomes the dominant salt inside the cell. Pressure tolerance Pyrococcus abyssi is a hyperthermophilic piezophile (or barophile). The archaeon is adapted to high temperature & high pressure environments by expressing an increased concentration of small chain amino acids compared to other species adapted to high temperature, but not high pressure (e.g. Pyrococcus furiosus). Image result for Pyrococcus abyssi Pyrococcus abyssi L'Internaute Pressure tolerance The effect of this amino acid change is to reduce the number of hydrophic residues, making the proteins tighter & more stable. Further research with Sulfolobus solfataricus revealed that changes in amino acid chains leading to changes in protein shape, resulted in a decrease in temperature & pressure stability of the proteins, and reduced the cells’ ability to survive in the extreme conditions. Therefore, sometimes, adaptations can work together to allow survival – in this example, high temperature and high pressure. But, this does not mean that the cells are adapted to survive at ALL environmental conditions. Summary points The ability of organisms to inhabit extreme environments is dependant upon their biochemistry and cell structure In general, organisms adapted to high temperature environments cannot survive at low temperatures, etc. These adaptations, are sometimes (but not always), the reverse adaptation- e.g. fatty acid composition of the cell membranes for high/low temperatures Some adaptations are complementary to other extreme environments – e.g. fatty acid composition for high temp. & low pH; while others are unique to that stress- e.g. halophile membranes lacking peptidoglycan, relying upon ionic stability from sodium ions in the environment. Summary of adaptations Location in High Low High Low High pH Low pH Example the cell tempe- temper- pressure pressure Organism rature ature Cell membrane Ribosomes Replication/ growth alterations Photo- synthetic OR mutualistic? Further Reading Brock Biology of Microorganisms – Chapter 2 Pepper et al. Environmental Microbiology – Chapter 2 – Chapter 4