Brain and Behaviour: Neurobiology, Consciousness, Learning
Document Details
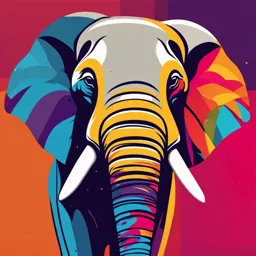
Uploaded by PamperedStrait1339
Università di Padova
Tags
Summary
These notes cover brain and behaviour, with a focus on neurobiology, consciousness, and learning. Topics include the relationship between biology and behavior, dualism vs. monism, neuron doctrine and brain localisation e.g. Broca's area. Other topics covered are neural adaptation, psychoactive substances and neural development.
Full Transcript
BRAIN AND BEHAVIOUR lesson 1 → introduction to the neurobiology of behaviour unit 1 REL. BETWEEN BIOLOGY AND BEH. The relationship between biology and behavior is circular. Just as our biology influences our behavior, so does our behavior influence our biology. We suspect that high levels of testost...
BRAIN AND BEHAVIOUR lesson 1 → introduction to the neurobiology of behaviour unit 1 REL. BETWEEN BIOLOGY AND BEH. The relationship between biology and behavior is circular. Just as our biology influences our behavior, so does our behavior influence our biology. We suspect that high levels of testosterone are associated with increased aggression. Biology in this case appears to be driving behavior. At the same time, we know that watching your favorite sports team lose acts to lower your testosterone. In this case, behavior (watching sports and supporting a team) drives biology (testosterone levels). The separation of psychology from the premises of biology is purely artificial, because the human psyche lives in indissoluble union with the body. Carl Jung DUALISM AND MONISM Descartes is notable for his support of mind-body dualism. According to Descartes, human beings had unique capacities that were contained in the mind, and separated from the body. For Descartes and other dualists, the mind was neither physical nor accessible to study through the physical sciences. The possibility of a physical explanation of mind processes became evident with the rise of experimental psychology, the study of human behavior under controlled conditions. The modern neurosciences, including biological psychology, are based on monism rather than dualism. The monism perspective proposes that the mind is the result of activity in the brain, which can be studied scientifically. In today’s world, we take for granted that the brain and nervous system are the sources of intellect, reason, sensation, and movement. NEURON DOCTRINE At the beginning of the 20h century the Spanish anatomist Santiago Ramón y Cajal applied chemicals used in photography (silver salts) to nerve tissue under the microscope and discovered that neurons had cell bodies and different projections. He argued that the nervous system was composed of an array of separate, independent cells rather than a unique interconnected network of fibers. He won the Nobel Prize in 1906 and his concept came to be known as the Neuron Doctrine: individual neurons are the elementary signaling elements of the nervous system. We now know that neurons are distinct entities that communicate via chemical signals. PHRENOLOGY A classic example of the ups and downs of brain science is the work of Franz Joseph Gall (1758–1828). Gall suggested that bumps on the skull were related with the functions of the brain beneath it. He proposed that the propensity for certain personality traits - such as generosity, reserve, and destructive mania - could be related to head size. To support this hypothesis, Gall and his followers measured the skulls of hundreds of people representative of various personality types, from the highly talented to the criminally insane. This new pseudoscience came to be known as phrenology. Phrenologists reasoned that parts of the brain that were related to a particular trait would have led to increases in brain size reflected in protuberances on the surface of the skull. Despite this mistake, Gall correctly assumed that the brain is the organ of the mind, and he was ahead of his time in suggesting that certain functions were localized in different structures in the brain. BROCA’S APHASIA In fact, in the middle 1800s, Parisian physician Paul Broca correlated the damage he observed in patients with their behavior and concluded that language functions were localized in the brain. Broca began to study a man named Leborgne, who came to be referred to as “Tan” because when questioned, “tan” was one of a very few syllables he could produce. Leborgne died shortly after Broca’s examination of him, and Broca performed an autopsy on his patient’s brain. Broca found significant damage to the patient’s left inferior frontal region, which is believed to play a role in speech production. This area is now referred to as Broca’s area in his honor and the symptoms of Broca’s patient Tan are known as Broca’s aphasia. The primary symptom is difficulty in producing speech: Speech is very slow and requires significant effort. WERNICKE’S APHASIA Shortly after Paul Broca presented his revolutionary discovery, Carl Wernicke published his observations on another type of language deficit. In honor of his contributions, this syndrome is now referred to as Wernicke’s aphasia, and the affected area of the brain - located on the superior surface of the temporal lobe - is known as Wernicke’s area. In Wernicke’s aphasia, speech is rapid and fluent but meaningless. Animal experiments followed soon after, offering solid experimental support for brain localization. The German physiologists Fritsch and Hitzig demonstrated that the application of small electrical currents to the external surface of the brain of a dog could stimulate small movements. It was later discovered that ablation of that same region of the brain caused muscle paralysis, while the occipital lobe was specifically needed for vision. We now know that there is a very clear division of tasks, so that different areas preside over different functions. Gall had the right insight. unit 2 → BIOLOGICAL BASIS OF CONSCIOUSNESS The last frontier of cognitive neuroscience is to understand the biological basis of consciousness. The question is: How do we become aware of our world?Consciousness is a subjective experience, as opposed to the condition of an organism simply being awake and responsive to sensory stimulation. Much progress has been made in conceptualizing consciousness in recent years. This work has focused on the question of how we come to be aware of our sensory world, and has suggested that perceptual consciousness emerges via cognitive processing in cortical circuits that assemble conscious experiences in real-time. STREAM OF CONSCIOUSNESS In 1890, William James defined consciousness as a continuous stream that, although accessible only to the subject experiencing it, might be experimentally approached by analyzing its several functions. The stream of consciousness is a continuous activity of the brain that involves attention, intentionality, and self-awareness. Neurobiology approaches the problem of consciousness in the same way, by studying tractable, well-defined components of consciousness, such as selective attention. SELECTIVE ATTENTION The phenomenon of selective attention is a particularly useful starting point for the study of consciousness. At any given moment we are aware of only a small fraction of the sensory stimuli that impinge upon us. As we look out on the world we focus on specific objects that have particular interest and exclude others. “My experience is what I agree to attend to”. (William James) Processing a stimulus seems all that is needed for there to be phenomenal consciousness of that stimulus. Focalization and concentration of consciousness are the essence of attention. One of the functions of selective attention is the intention to direct a movement to a target object. This is an example of how a percept leads to a voluntary act. Selective attention sharpens our senses to improve motor planning. BLINDSIGHT Neurological disorders such as blindsight casts some light on the study of consciousness. Blindsight is the ability of people with lesions of the primary visual cortex that cannot see what is shown before their eyes, to respond to visual stimuli that they do not consciously see. Patients can make correct guesses when forced to make a choice. They perceive, but are not conscious of perceiving. They can scale their grasping movement as they reach out to pick up objects of different sizes placed in their blind field, even though they cannot report the sizes of the objects. Similarly, they can avoid obstacles placed in their blind field, but they do not report seeing them (Whitwell et al., 2011; Striemer et al., 2009) A possible explanation for the phenomenon of blindsight is that tiny islands of functioning tissue in the visual cortex remain. These islands aren't large enough to provide conscious perception, but nevertheless enough for some unconscious visual perception. UNCONSCIOUS INFO PROCESSING Blindsight challenges the common belief that perceptions must enter consciousness to affect our behavior, by showing that our behavior can be guided by sensory information of which we have no conscious awareness. In fact, consciousness has only recently arrived on the scene of evolution, during which unconscious information processing was the rule (LeDoux, The Emotional Brain, 1996). Just think that entire emotional systems that control innate behaviors (e.g., run away and hide in case of danger) and related physiological responses (e.g., increased heart rate) operate unconsciously. SELF AWARENESS Self-awareness and its development are nowadays the neuropsychological holy grail. In 1970 the American psychologist Gordon Gallup and his colleagues developed the mirror test - an interesting way to test whether chimpanzees had a sense of self. The animal is anesthetized and then marked (e.g., painted or a sticker attached) on an area of the body the animal cannot normally see. When the animal recovers from the anesthetic, it is given access to a mirror. If the animal then touches or investigates the mark, it is taken as an indication that the animal perceives the reflected image as an image of itself, rather than of another animal. The rouge test is a version of the mirror test used with human children. If you place a dot of red dye on the forehead of a human infant of about 18 months of age, the infant will touch his or her own forehead when looking in the mirror to wipe the marks off. In other words, infants looking into a mirror understand that they are gazing at an image of themselves. This test is widely cited as the primary measure for mirror self-recognition in human children. NEUROSCIENCE OF THE SELF Is it possible to locate the self in the brain? In their review of brain correlates of the self provide a model for determining whether a certain brain activity is essential to a sense of self. Their approach is to measure activity in different parts of participants’ brains while they first do certain things, then watch other people do the same things. For example, if some area of the brain behaves differently when we think about ourselves reading this text as opposed to when we think about our classmates reading the text, we might have located an area in the brain involved with some aspect of self. Ruby and Decety found distinct patterns of brain activity accompanying consideration of our own actions as opposed to the actions of others. When participants imagined themselves stapling papers or peeling bananas, a different pattern of brain activity occurred than when they imagined the experimenter carrying out the same actions. WHEN THE BRAIN LOSES ITSELF Goldberg and colleagues (2006) presented participants with a series of photos and musical clips while undergoing functional MRI. Brain activity patterns were compared over three conditions. In the first, stimuli were presented slowly, and participants pushed a button if an animal appeared in a picture or if a trumpet was heard in a music clip. In the second condition, stimuli were presented three times as rapidly as in the first. In the third condition, participants used buttons to indicate their emotional response to the stimuli. In two of the conditions, the slow presentation of stimuli and the emotional response conditions, frontal lobe activity accompanied activation of the sensory cortex. However, in the rapid presentation of stimuli, no such frontal activation occurred. The researchers speculated that when faced with demanding sensory tasks, the self part of the brain, represented in the frontal lobe, literally switches off. If there is a sudden danger, such as a snake, it is not helpful to stand around wondering how one feels about the situation! THOUGHT AUTHORSHIP In a very recent experiment, researchers convinced participants doing fMRI that their thoughts were generated by an external technical device. Self-reports indicate that participants experienced their thoughts as self-generated when they believed the (fake) device was deactivated, and attributed their thoughts externally when they believed the device was activated-an experience usually only reported by patients diagnosed with schizophrenia. Interestingly, activations were found in the medial prefrontal cortex (mPFC). Results thus support the notion that the mPFC might be involved in thought authorship as well as anomalous self-experiences in patients with schizophrenia. COLLECTIVE NEURAL EFFORT So far, there is no evidence that there is one site for consciousness, which leads experts to believe that it might be a collective neural effort. Anyway, Eagleman challenges the primacy of consciousness. With most forms of expertise, such as driving, the conscious mind merely gets in the way. Furthermore, unconscious influences on our decisions are pervasive. For instance, judges deciding whether to release on parole prisoners are more likely to do so if they have eaten before. Decisions are little more than the product of unconscious neural battles between competing drives (LeDoux & Brown, 2017). unit 3 TEN PERCENT OF BRAIN MYTH You have probably heard the expression, “You only use 10 percent of your brain.” It is suggested that a person may harness this unused potential and increase intelligence or other skills. This little bit of misinformation has become part of our popular culture. The film Lucy depicts a character who gains increasingly godlike abilities once she surpasses 10 percent, watch here a video. A likely origin for the "ten percent myth" is the reserve energy theory of William James, according to which people only meet a fraction of their full mental potential. It would be nice to think that we all had a large chunk of brain awaiting more extensive use, but we know today that it is definitely false. REFUTING THE 10% MYTH Neuroscientist Barry Beyerstein sets out a number of evidences refuting the ten percent myth: 1. The new techniques for imaging the brain show that separate parts of the brain become more active during different tasks but that each has its particular role to play; 2. From an evolutionary standpoint, it seems unlikely that we would bother to evolve structures that did not contribute directly to our chances of survival; 3. The human brain contains 5 percent of the body’s mass, but it consumes 20 percent of its glucose and oxygen. It would be an extravagant waste to use only 10 percent of an organ that used so many resources; 4. Finally, observations of patients with brain damage show that even small injuries, such as those caused by a concussion, can have a detrimental effect on brain functioning. A person who experienced damage to 90 percent of the brain would likely be dead or, at a minimum, unable to emerge from a coma. BRAIN SIZE AND FUNCTIONAL CONNECTIVITY The outstanding feature of humans is brain size. However, brain size does not correlate well with intelligence. After all, Albert Einstein’s brain was, on average, smaller than age-matched controls. Notably, since the initial appearance of Homo sapiens about 200,000 years ago, brain size has not changed much. What is unclear is why such major cultural changes over the past 200,000 years, such as agriculture, urbanization, and literacy, have not produced additional changes in brain size. Further increases in brain size might simply be too costly in terms of greater difficulties in childbirth and the need for more resources. Higher transmission speed (through myelinated axons) and functional connectivity of white matter tracts seems the ultimate strategy to increase information processing without changing brain size. Note that even in cases of ablation, it is possible a recovery mediated by the connections of white matter. NEW GEOGRAPHY OF THE MIND Neuroscience is changing perspective: from a localization perspective to a connectome approach. In recent years, we are witnessing an epochal turning point that redesigns the new geography of the mind. The concept of connectome is born, that is a comprehensive map of neural connections in the brain. Imagine an extensive network of state highways and freeways that connect the whole territory and by studying them we can know the quantity and direction of information transmitted. This term was directly inspired by the ongoing effort to sequence the human genetic code—to build a genome. In a similar manner, one could consider the brain connectome, a set of all neuronal connections, as one single entity, thus emphasizing the fact that the huge brain neuronal communication capacity and computational power critically relies on this subtle and incredibly complex connectivity architecture. The term "connectome" was popularized by Sebastian Seung's speech given in a TED conference, watch here the video. He discusses the high-level goals of mapping the human connectome, as well as ongoing efforts to build a three-dimensional neural map of brain tissue at the microscale. The Human Connectome Project has tackled one of the great scientific challenges of the 21st century: mapping the human brain, aiming to connect its structure to function and behavior. One of the important implications of connectomics is that it views the brain as a plastic organ, changing in quantity and quality over the course of a lifetime. The connectome changes over the course of life depending on our experiences. For Seung, this is a different perspective than the perspective typical of genomics, according to which everything is already in our genes and what we are is decided from conception. Eagleman challenges our view by describing the five human senses (namely sight, smell, touch, taste, and hearing) as flexible “peripheral plug-and-play” devices. In fact, we already exploit this feature with cochlear and retinal implants for people who have hearing or visual impairments. And we can even control a robot arm through motor-cortex activity. For example, researchers have shown that a person with quadriplegia can sip from a bottle, by simply thinking about the action. Read here the online article "Neuroprosthetics: Once more, with feeling". BRAIN MACHINE INTERFACES (BMI) Over the past 15 years, researchers have shown that a rat can make a robotic arm push a lever, a monkey can play a video game and a person with quadriplegia can sip from a bottle of coffee, all by simply thinking about the action. Improvements in prosthetic limbs have been equally dramatic, with devices now able to move individual fingers and bend at more than two dozen joints. The loss of a limb or paralysis resulting from spinal cord injury has devastating consequences on quality of life. One approach to restoring lost sensory and motor abilities in amputees and patients with tetraplegia is to supply them with implants that provide a direct interface with the CNS. Such brain–machine interfaces (BMI) might enable a patient to exert voluntary control over a prosthetic or robotic limb. → Electronic interfaces with the brain enable us to read out and 'decode' hundreds of neural signals from motor areas of the brain in order to control increasingly sophisticated robotic arms. → Parallel interface, then, could convey sensory information about the consequences of these movements back to the patient. Recent developments in the algorithms that decode motor intention from neuronal activity and in approaches to convey sensory feedback by electrically stimulating neurons have shown the promise of new interfaces with sensorimotor cortices (Chandrasekaran et al., 2021) REGROWTH OF PERIPHERAL AXONS How much further can we take it? Does regrowth of peripheral axons make body part transplants possible? Cadaver arms have been transplanted onto a woman’s body, by exploiting the ability of peripheral nerves to regenerate and reestablish some movement and sensation in the attached body parts. Unfortunately, duplicating this success in the central nervous system (spinal cord and brain) remains a challenge. TRANSPLANT OF OECs Regeneration within the central nervous system [CNS] generally does not occur naturally. On the other hand, the olfactory system is characterized by its ability for sensory neuron regeneration throughout life in healthy humans following injury or disease. Olfactory ensheathing cells [OECs], the glial cells of the olfactory system, play a key role to support the regeneration and guidance of olfactory receptor neurons from the peripheral nervous system into the central nervous system by creating a permissive environment for neurite outgrowth. These cells allow the nerve cells that give us a sense of smell to re-grow when they are damaged. Due to this unique ability, OECs are a promising potential cell therapy to aid regeneration. Transplantation of OECs into the injured spinal cord has shown positive therapeutic effects in animal models and in human phase I clinical trials, demonstrating the safety of OEC transplantation. However, the results are still variable. Read this online article. Prof. Geoffrey Raisman at the UCL Institute of Neurology developed a pioneering cell transplantation treatment. His team inserted olfactory cells in the spinal cord of a paralyzed man. The man can now walk using a frame. Watch this video to know more. lesson 2 → neural basis of cognition WILL WE READ MINDS WITH NEUROIMAGING TECHNIQUES? Progress in neurosciences over the past hundred years accelerated rapidly as new methods became available for studying the nervous system. In the 2002 film, Minority Report, Tom Cruise plays a police officer who arrests people for crimes they have not yet committed. Is it possible to know what people are planning to do before they actually do it? In 2000, O’Craven and Kanwisher were able to determine, with an 85% accuracy rate, whether a person was imagining a building or a face simply by looking at fMRI scans of the brain. Imagining a face activates a part of the brain that typically responds when we view a face, the fusiform face area (FFA), whereas imagining a building activated a part of the brain that typically responds to places, the parahippocampal place area (PPA). Researchers are closing in on the ability to use fMRI to detect deception (Langleben et al., 2005). The ability to detect deception directly through brain scans promises enormous benefits to criminal justice and national security, but raises equally substantial concerns about ethics. Will legislators need to expand our privacy rights to include “mental privacy”? WHAT WILL EEG TELL US ABOUT THE BRAIN? Nerve cells are capable of generating small electrical charges, much like miniature batteries. This electrical activity can be recorded using electrodes on the surface of the skull. EEG recordings measure the activity of large numbers of cells, known as a field potential. Because the recording electrodes are located on the surface of the scalp, the EEG is most highly influenced by the activity of cortical cells closest to the electrodes. Cells from deeper structures in the brain have little, if any, influence on the EEG. Nowadays, computerized analysis of EEG recordings can be used to generate animations of activity over time and for the construction of 3-D maps of brain activity. EVOKED POTENTIALS An application of basic EEG technology that is used in the assessment of sensory activity is the recording of evoked potentials. This technique allows researchers to correlate the activity of cortical sensory neurons recorded through scalp electrodes with stimuli presented to the participant. This type of analysis can be helpful in cases in which a person’s behavior does not provide a clear indication of whether a particular stimulus has been perceived. For example, young children with autism often behave as though their hearing were impaired. When spoken to by parents or others, a child with autism often shows no reaction at all. Through observations of evoked potentials to sound, we can determine whether the child can actually hear. MRI Magnetic Resonance Imaging (MRI) has become a standard medical diagnostic tool and a valuable research tool. With current imaging technologies, we can watch the living brain as it engages in processes such as reading or emotional response. We can identify differences in the ways the brains of serial murderers function compared with the brains of typical people. This technology uses powerful magnets to align hydrogen atoms within a magnetic field. Next, radio frequency (RF) pulses produce “resonance,” or spinning, of the hydrogen atoms. When the RF pulses cease, the hydrogen atoms return to their natural alignment within the magnetic field. As the atoms “relax,” each emits a pulse that is detected by the scanner. The coloration of each area represents the level of pulse activity in that area. FUNCTIONAL MRI IS USED TO ASSESS BRAIN ACTIVITY Functional MRI (fMRI) uses a series of MRI images taken 1 to 4 seconds apart in order to assess the activity of the brain. Functional MRI takes advantage of the fact that active neurons require more oxygen than less active neurons, and that variations in blood flow to a particular area will reflect this need. The idea of tracking blood flow in the brain was previewed by Italian physiologist Angelo Mosso on patients with head injuries. They were missing skull bones and Mosso was able to measure and correlate blood flow with their mental activity. Hemoglobin, the protein molecule that carries oxygen within the blood, has different magnetic properties when combined with oxygen or not (Ogawa et al., 1990). Consequently, signals from a voxel will change depending on the oxygenation of the blood in that area, known as the Blood Oxygenation Level Dependent (BOLD) effect. BRAIN STIMULATION One of the important questions raised in biological psychology relates to the localization of functions within the brain. We can approach this question by artificially stimulating the area in question and watching for resulting behavior. Electrical stimulation of the brain can be useful during neurosurgery. Before operating, the surgeon will want to know whether an essential function such as language is being managed by neurons in the area in question. By stimulating this area with a small amount of electricity and assessing any changes in behavior, the surgeon can identify whether the area participates in a particular type of behavior. Considerable knowledge regarding the mapping of the cortex has been derived from this technique. Neurosurgeon Penfield investigated the brains of more than a thousand patients undergoing surgery for the treatment of epilepsy and he contributed significantly to our understanding of the mapping of motor cortex, memory, and language (Penfield, 1958). TRANSCRANIAL MAGNETIC STIMULATION (TMS) Single-pulse transcranial magnetic stimulation (spTMS) consists of a magnetic pulse delivered through a coil of wire encased in plastic that is placed on the scalp. Repeated transcranial magnetic stimulation (r TMS) consists of sequences of repeated magnetic pulses. Low frequency rTMS (about one pulse per second) provides an interesting technique for temporarily reducing activity in underlying cortical regions (Hoffman et al., 2003). In some cases, r TMS will inhibit a certain function, such as auditory hallucinations (“hearing voices”) among some patients with schizophrenia (Hoffman et al., 2005). In other cases, r TMS appears to disinhibit behavior, or allow a normally inhibited behavior to be expressed. For example, Snyder et al. (2006) used r TMS to temporarily produce unusual calculation skills, like those occasionally found in people with autism, in their healthy participants. Repeated TMS has also shown promise in treating some cases of depression. TMS AND SAVANT BEHAVIOURS Savant behaviors are exceptional skills and talents found in people whose intellectual functioning falls within the range of mental retardation. Dustin Hoffman in the film Rain Man was able to count the number of pennies in a jar instantly. Although the origin of savant behaviors remains a mystery, current speculation centers on possible damage to the left hemisphere, leading to compensating activity in the right hemisphere. The exceptional talents demonstrated by savants usually occur in modalities that we associate with right-hemisphere function such as art, music, mathematics, and spatial skills. All of us may have the necessary circuitry to be savants, but our left-hemisphere dominance can overshadow these abilities. Repeated transcranial magnetic stimulation (rTMS), which temporarily inhibits the activity of the underlying cortex, was applied to healthy participants in left-hemisphere regions. Following stimulation, some participants experienced savant-like abilities that lasted a few hours. FMRI AND ROMANTIC LOVE Romantic love produces characteristic patterns of activity in the human brain. While undergoing fMRI, participants were shown photographs of people they described as either friends or true loves (Bartels & Zeki, 2000). When participants viewed lovers, increased activity was observed in areas of the brain often associated with reward. At the same time, areas associated with negative emotions and social judgment were less active. This means that “love” is not only rewarding, but it makes us less judgmental of the lover (Acevedo et al., 2012). To learn more about our very real, very physical need for romantic love, Helen Fisher and her research team took fMRIs of people in love -- and people who had just been dumped. CNS + PNS The human brain is estimated to have about a hundred billion nerve cells, two million miles of axons, and a million billion synapses, making it the most complex structure on earth. We divide the entire nervous system into two components, the central nervous system (CNS) and the peripheral nervous system (PNS). CNS→ The central nervous system includes the brain and spinal cord. PNS→ The peripheral nervous system contains all the nerves that exit the brain and spinal cord, carrying sensory and motor messages to and from the other parts of the body. The tissue of the CNS is encased in bone, but the tissue of the PNS is not. Crucially, damage to the CNS is considered permanent, whereas recovery can occur in the PNS. SPINAL CORD The spinal cord is a long cylinder of nerve tissue that extends from the brain, down to the first lumbar vertebra (a bone in the vertebral column) The spinal cord is the information superhighway and it appears white. Do you know why? White matter is made up of nerve fibers known as axons, the parts of neurons that carry signals to other neurons. The tissue looks white due to myelin, which covers the axons. These large tracts of axons are responsible for carrying information to and from the brain: Axons from sensory neurons carry information about touch, position, pain, and temperature. Axons from motor neurons are responsible for movement. The spinal cord neurons are capable of some important reflexes. The patellar reflex, that your doctor checks by tapping your knee, produces a reflexive contraction of the quadriceps muscle of the thigh, causing the foot to kick. Spinal reflexes also protect us from injury. If you touch something hot or step on something sharp, your spinal cord produces a withdrawal reflex. You immediately pull your body away from the source of the pain. Running down the center of the spinal cord is the central canal. The central canal (also known as ependymal canal) is the cerebrospinal fluid-filled space that runs through the spinal cord. The central canal helps to transport nutrients to the spinal cord as well as protect it by cushioning the impact of a force when the spine is affected. NERVES A nerve - an enclosed bundle of axons - is the basic unit of the PNS. It provides a common pathway for the action potentials that are transmitted along each of the axons to peripheral organs or, in the case of sensory nerves, from the periphery back to the central nervous system. Starting closest to the brain, there are eight cervical nerves that serve the area of the head, neck, and arms. - Below the cervical nerves are the 12 thoracic nerves, which serve most of the torso. - Five lumbar nerves come next, serving the lower back and legs. - The five sacral nerves serve the backs of the legs and the genitals. - Finally, we have the single coccygeal nerve. → Spinal injuries A person with cervical damage is a quadriplegic (quadri meaning “four,” indicating loss of control over all four limbs). All sensation and ability to move the arms, legs, and torso are lost. A person with lumbar-level damage is a paraplegic. Use of the arms and torso is maintained, but sensation and movement in the lower torso and legs are lost. Spinal damage is considered permanent, but significant progress is being made in repairing the spinal cord CEREBELLUM Just above the spinal cord is located the Brainstem (“stem” is the part of a plant that grows from the root and that supports the leaves). It contains a major structure: the cerebellum Cerebellum means “little brain” in Latin and it looks indeed like a second little brain. The use of “little” is misleading because the cerebellum actually contains more nerve cells (neurons) than the rest of the brain. The internal structure of the cerebellum resembles a tree. White matter, or axons, forms the trunk and branches, while gray matter, or cell bodies, forms the leaves. The traditional view of the cerebellum emphasizes its role in coordinating voluntary movements, maintaining muscle tone, and regulating balance. Input from the spinal cord tells the cerebellum about the current location of the body in 3-D space. Input from the cerebral cortex tells the cerebellum about the movements you intend to make. The cerebellum processes the sequences and timing of muscle movements required to carry out the plan you intend to make. More contemporary views see the cerebellum is responsible for much more than balance and motor coordination, the cerebellum also participates in learning (Marr, 1969). Because the cerebellum is one of the first structures affected by the consumption of alcohol, most sobriety tests, such as walking a straight line, are actually tests of cerebellar function. FOREBRAIN The forebrain contains the most advanced and most recently evolved structures of the brain. In particular, it contains the symmetrical left and right: 1. thalamus 2. hypothalamus 3. hippocampus 4. amygdala cerebral hemispheres THALAMUS AND HYPOTHALAMUS Inputs from most of our sensory systems converge on the thalamus, which then forwards the information on to the cerebral cortex for further processing. In addition to its role in sensation, the thalamus is also involved with states of arousal and consciousness. Just below the thalamus is the hypothalamus. The name hypothalamus literally means “below the thalamus.” The hypothalamus is a major regulatory center for such behaviors as eating, drinking, sex, biorhythms, and temperature control. The hypothalamus also produces our so-called fight-or-flight response to emergencies. Electrical stimulation to parts of the hypothalamus can produce pleasure, rage, and fear as well as predatory behavior. HIPPOCAMPUS AND AMYGDALA The hippocampus, named after the Greek word for “seahorse,” participates in learning and memory. Damage to the hippocampus in both hemispheres produces a difficulty in forming new memories for facts, language, and personal experience. The amygdala plays important roles in fear, rage, and aggression. In addition, the amygdala interacts with the hippocampus during the encoding and storage of emotional memories. Damage to the amygdala specifically interferes with an organism’s ability to respond appropriately to dangerous situations. In laboratory studies, rats with damaged amygdala were unable to learn to fear tones that reliably predicted electric shock. Stimuli that normally elicit fear in monkeys, such as rubber snakes or unfamiliar humans, failed to do so in monkeys with lesions in their amygdala. In humans, autism, which produces either extreme and inappropriate fear and anxiety or a complete lack of fear, might involve abnormalities of the amygdala. CORTEX The outer covering of the cerebral hemispheres is called cortex from Latin, as is the outside covering of the trees. This thin layer of gray matter (i.e., made by cell bodies) varies from 1.5 mm to 4 mm in thickness in different parts of the brain. Below there are vast fiber pathways that connect the cortex with the rest of the nervous system. The cerebral cortex has a wrinkled appearance like the outside of a walnut. Why is the cerebral cortex so wrinkled? This feature of the cortex provides more surface area for cortical cells. We have limited space within the skull for brain tissue, and the wrinkled surface of the cortex allows us to pack in more neurons than we could otherwise. If stretched out flat, the human cortex would cover an area of about 1 square meter. SYNAPSES The human brain has more synapses than there are stars in our galaxy. A first step toward understanding the mind is to learn how neurons communicate by means of synaptic transmission. The synaptic gap is a space between the transmitting and receiving neurons. Most neurons have a large number of branches known as dendrites, from the Greek word for “tree.” The dendrites serve as locations at which information from other neurons is received. At each synapse on a dendrite, special ion channels serving as receptor sites are embedded in the neural membrane. These receptor sites interact with molecules of neurotransmitter released by adjacent neurons that float across the synaptic gap, a fluid-filled space between the transmitting and receiving neurons. ELECTRICAL AND CHEMICAL SYNAPSES The birth and propagation of the action potential within the presynaptic neuron makes up the first half of neural communication. The second half begins when the action potential reaches the axon terminal and the message crosses the synaptic gap to the adjacent postsynaptic neuron. Electrical synapse → type of synapse in which a neuron directly affects an adjacent neuron through the movement of ions from one cell to the other. Chemical synapse → type of synapse in which messages are transmitted from one neuron to another by chemical neurotransmitters. The signaling at chemical synapses can be divided into 2 steps: 1. The 1st step is release of the neurotransmitters by the presynaptic cell. The neurotransmitters bind to postsynaptic receptors and then the signal is terminated. 2. The 2nd step is the reaction of the postsynaptic cell. The neurotransmitters can produce a slight depolarization/hyperpolarization of the postsynaptic membrane. NEUROTRANSMITTER RELEASE Neurotransmitters are stored in synaptic vesicles. When these vesicles are released from their anchors, they migrate toward the release sites. Here, calcium stimulates the fusion between the membrane of the vesicle and the membrane of the axon terminal, forming a channel through which the neurotransmitters escape Exocytosis is the process in which vesicles fuse with the membrane of the axon terminal and release neurotransmitter molecules into the synaptic gap. Neurotransmitters Bind to Postsynaptic Receptor Sites The newly released molecules of neurotransmitter float across the synaptic gap. On the postsynaptic side of the synapse, receptor sites are characterized by recognition molecules that respond only to certain types of neurotransmitter. The molecules of neurotransmitters function as keys that fit into the locks. As soon as the postsynaptic receptor captures molecules of neurotransmitter, the ion channel opens. TERMINATION OF THE CHEMICAL SIGNAL Before we can make a second telephone call, we need to hang up the phone to end the first call. Likewise, if we want to send a second message across a synapse, it’s necessary to end the first message. Neurotransmitters released into the synaptic gap must be deactivated before another signal is sent by the presynaptic neuron. Neurons have three ways of ending a chemical message: 1. The first method is simple diffusion: neurotransmitters diffuse away from areas of high concentration to areas of low concentration; 2. The second method is deactivation: neurotransmitters are deactivated by enzymes in the synaptic gap. The enzymes break the neurotransmitter molecules into their components. The presynaptic neuron collects these components and then synthesizes and packages other neurotransmitters; 3. The third method is reuptake: the presynaptic membrane recapture neurotransmitters and return them to the axon terminal. Here they can be repackaged in vesicles for subsequent release. Reuptake spares the extra step of reconstructing the molecules out of component parts. POST-SYNAPTIC POTENTIALS When neurotransmitters bind to postsynaptic receptors, they can produce one of two outcomes: 1. a slight depolarization of the postsynaptic membrane, known as an excitatory postsynaptic potential; 2. a slight hyperpolarization of the postsynaptic membrane, which reduces the likelihood that the postsynaptic cell will produce an action potential, known as an inhibitory postsynaptic potential. Each neuron in the CNS is constantly bombarded by synaptic input from other neurons. Some are excitatory, others inhibitory. The different inputs can reinforce or cancel one another. These competing inputs are integrated by a process called Neuronal Integration. If the net input is above threshold, an action potential may be generated. To fire or not to fire an action potential? According to Charles Sherrington, this decision making is the brain’s most fundamental operation. TETANUS AND LACK OF MUSCULAR INHIBITION Worldwide, over 1 million deaths per year still occur due to tetanus. Tetanus occurs when spores of the bacterium Clostridium tetani enter the body through a wound and produce a neurotoxin. This toxin blocks a neurotransmitter (GABA) in charge of inhibitory inputs to the nervous system. GABA is the most common transmitter in the CNS that activates inhibitory receptors and hyperpolarizes the membrane. Without normal inhibition from GABA, muscles begin to involuntary contract, and this may lead to respiratory or cardiac arrest. Three important classes of drugs – benzodiazepines, barbiturates, and alcohol – bind to GABA receptors and enhance the inhibitory effect. lesson 3 → psychopharmacology unit 1 PSYCHOACTIVE SUBSTANCES Psychoactive substances produce their behavioral effects by interacting with the existing chemistry of the CNS. In the previous lesson, we traced the propagation of neurotransmitters through the synapse. Neurotransmitters are chemical messengers synthesized within the neuron, and are released in response to the arrival of an action potential. They produce a reaction on the postsynaptic cell, and are deactivated following release by reuptake or by the action of enzymes. Drugs can affect neurotransmitter’s propagation at different stages: 1. Synthesis 2. Storage within the axon terminal 3. Release 4. Interactions with receptor sites 5. Reuptake or enzyme activity following release AGONISTS AND ANTAGONISTS Drugs can boost or reduce the activity of a neurotransmitter. Drugs that enhance the activity of a neurotransmitter are known as agonists. Drugs that reduce the activity of a neurotransmitter are known as antagonists. It is important to avoid equating agonists with excitation and antagonists with inhibition.If a neurotransmitter has an inhibitory effect on the postsynaptic neuron, the action of an agonist would increase the amount of inhibitory input, resulting in reduced postsynaptic activity. The action of an antagonist, instead, interfering with the inhibitory neurotransmitter, would result in greater than normal postsynaptic activity. Consider the case of caffeine. Caffeine produces behavioral stimulation. Caffeine is an antagonist for adenosine, which means it reduces adenosine’s effects. Because adenosine acts as an inhibitor at the synapse, inhibiting an inhibitor leads to behavioral excitation. SYNTHESIS AND STORAGE OF NEUROTRANSMITTERS Let’s see how drugs can affect neurotransmitter’s propagation at different stages: Synthesis of neurotransmitters → Manipulating the synthesis of a neurotransmitter will affect the amount available for release. Substances that increase production will act as agonists, whereas substances that interfere with production will act as antagonists. The simplest way to boost the rate of neurotransmitter synthesis is to provide the basic building blocks, or precursors, for the neurotransmitter. The synthesis of Serotonin begins with the tryptophan. Tryptophan is obtained from grains, meat, and dairy products. Serotonergic activity has been implicated in a variety of behaviors, including sleep, mood, and appetite. Storage of neurotransmitters → Certain drugs have an antagonistic effect by interfering with the storage of neurotransmitters within the neuron. For example, the drug reserpine, used to reduce blood pressure, interferes with the storage of serotonin into synaptic vesicles. As a result, reserpine’s interference often results in profound depression. 15 percent of all patients who used reserpine to lower blood pressure also experienced severe depression. RELEASE OF NEUROTR. Black widow spider venom is a cholinergic agonist, promoting greater than normal release of ACh at the neuromuscular junction. This release overstimulates muscle fibers, leading to convulsions. Neurons that use acetylcholine (ACh) as their major neurotransmitter are referred to as cholinergic neurons. ACh is the primary neurotransmitter at the neuromuscular junction, the synapse (2) between a neuron (1) and a muscle fiber (3). In addition, cholinergic neurons participate in learning and memory. Not too surprisingly, given the memory loss associated with Alzheimer’s disease, they deteriorate as a result of this disease. Nicotinic receptors respond to both ACh and to nicotine, found in all tobacco products. Interestingly, researchers have identified a gene that is involved with the development of nicotinic receptors (i.e., receptor subtypes of Ach) that appears to play important roles in lung cancer and addiction to nicotine. One variant of this gene is strongly associated with increased risk of lung cancer. Other drugs act as antagonists by preventing neurotransmitter release. Powerful toxins produced by botulinum bacteria, found in spoiled food, prevent the release of ACh at the neuromuscular junction. The resulting disease, botulism, leads rapidly to paralysis and death. Botox is the trade name for one of the seven botulinum toxins. Botox is used to paralyze muscles to prevent the formation of wrinkles and to treat a variety of medical conditions involving excess muscle tension (e.g., focal dystonia). Concerns have been raised by an Italian team of researchers about the ability of Botox to move from the injection site to the brain (Antonucci et al., 2008). RECEPTOR EFFECTS The greatest number of drug effects occur at the receptor. In some cases, drugs mimic the action of neurotransmitters at the receptor site. In other cases, drugs can block synaptic activity by occupying a receptor without activating it. Consider the lock-and-key analogy. Both neurotransmitters and agonists at the receptor site act like keys that can open locks. Antagonists act like a poorly made key that fits in the lock but fails to open it. As long as the ineffective key is in the lock, real keys cannot be used to open it. For example, nicotine opens locks at the Ach receptor sites and acts as an agonist. Curare, instead, blocks these receptors and causes paralysis. Curare is a substance derived from Amazonian plants. People in this region have historically used curare to tip their darts for hunting. The venom from the cobra acts in the same way as curare. It occupies the nicotinic receptors located at the neuromuscular junction. ACh is unable to stimulate muscle fibers. Inactivation of the muscles of the diaphragm, required for breathing, leads to paralysis and death. A number of important drugs exert their influence on the GABA receptors. GABA serves as the major inhibitory neurotransmitter of the central nervous system. These receptors can be activated by the benzodiazepines, a class of tranquilizers that includes Valium, alcohol, and barbiturates, which are used in anesthesia. Because GABA has an inhibitory effect on postsynaptic neurons, GABA agonists enhance inhibition. REUPTAKE EFFECTS AND ENZYMATIC DEGRADATION Drugs that interfere with either reuptake or enzymatic degradation of neurotransmitters are usually powerful agonists. They promote the activity of the neurotransmitter by allowing more of the released substance to stay active in the synapse for a longer period of time. People who suffer from major depressive disorder (see Lesson 9) generally have lower than normal levels of Serotonin. With slower reuptake, Serotonin can remain active in the synapse for a longer period of time, providing some relief from symptoms of depression. The antidepressant medication Prozac is a reuptake inhibitor Cocaine, amphetamine, and Ritalin inhibit the reuptake of dopamine. Consequently, they are powerful dopamine agonists. Dopamine is widely distributed throughout the brain and is particularly involved with systems mediating movement, reinforcement, and planning: 1. Projections to the basal ganglia provide an important modulation of motor activity. This pathway appears to be particularly damaged in cases of Parkinson’s disease, in which patients have great difficulties initiating movement (see Lesson 5); 2. Another dopaminergic pathway projects to the limbic system (the hippocampus, the amygdala, and the nucleus accumbens). This pathway participates in feelings of reward, and it plays an important role in addiction; 3. Finally, another group of dopaminergic neurons projects to the frontal lobe of the cerebral cortex. These neurons participate in higher-level cognitive functions, including the planning of behavior. unit 2 PLACEBO EFFECTS Drugs have different effects on the nervous system, depending on their method of administration (e.g., smoking or inhaling). In the case of nicotine, smoking a cigarette produces a faster increase in blood nicotine concentration than chewing the same dose of tobacco. Drug effects are also influenced by a number of individual factors, such as body weight. Larger bodies have more blood than smaller bodies and therefore require larger quantities of a drug to reach an equivalent concentration. Drug effects can be influenced also by expectations. These outcomes are called “placebo effects”. A placebo is an inactive substance, but when a participant expects a placebo to reduce pain, for instance, brain imaging demonstrates activation of the endorphin system (Zubieta et al., 2005). NEURAL ADAPTATION What you probably do not know is that your body has protective mechanisms designed to deactivate toxins. For instance, as we already saw, the blood–brain barrier prevents many toxins from entering the tissues of the nervous system. In some cases, a vomiting reflex triggered by the brainstem can clear the stomach and prevent further damage. In general, the body attempts to maintain homeostasis (i.e., balance). Repeated administration of a drug often leads to compensating effects, such as Neural Adaptation, that cancel out drug effects, leading to stable behavior. As a consequence, the person must take greater and greater quantities of the drug to obtain again the desired effects (tolerance). TOLERANCE Tolerance develops when a drug’s effects are lessened as a result of repeated administration. Notably, when the use of the drug is reduced or removed (withdrawal), only the compensating behaviors remain. As a result, withdrawal has the opposite effects of the formerly used drug. A person in withdrawal from a sedative will become agitated, whereas a person in withdrawal from a stimulant will become lethargic. Heroin, nicotine, and caffeine are associated with significant withdrawal symptoms. Tolerance effects can occur due to different processes: changes in enzymes, changes at the level of the synapse (e.g., changes in receptor density), and learning. For instance, “classical conditioning” can produce tolerance, so that a conditioned cue (e.g., seeing a smoker lightening a cigarette) can trigger the bodies’ compensatory mechanisms for the drug by itself. Environmental factors as well can cause or reduce addiction. During Vietnam War many soldiers used heroin due to the ready availability in Southeast Asia. However, fewer than 10 percent of addicted veterans relapsed, when returning to the United States. STIMULANT DRUGS:COFFEE Psychoactive drugs circumvent the protective systems of the blood–brain barrier to gain access to the central nervous system and produce psychological effects. Stimulant drugs share the capacity to increase alertness and mobility. For most people, caffeine increases blood pressure and heart rate, improves concentration, and wards off sleepiness. The substance is found in tea, coffee, and pain relievers. Caffeine acts as an antagonist for adenosine: it reduces the normal inhibitory activity of adenosine, producing thereby excitation. Caffeine’s interference with adenosine’s inhibition in the basal ganglia produces improvements in reaction time. Caffeine can produce a withdrawal syndrome. Because caffeine reduces blood circulation to the brain, withdrawal can produce headaches due to increased blood flow. Caffeine withdrawal also produces feelings of fatigue. Because caffeine crosses the placenta easily, and the fetus is relatively unable to metabolize caffeine, it is prudent for pregnant women to abstain from all sources of caffeine (Aldrich et al., 1979). NICOTINE,COCAINE AND AMPHETAMINE After caffeine, the most commonly used stimulant is nicotine. Nicotine acts as an agonist at the nicotinic cholinergic receptor. These receptors are present at the neuromuscular junction and in the basal forebrain, thus explaining increased alertness and cognitive performance. Nicotine also stimulates dopaminergic neurons in the nucleus accumbens. This action is the likely cause of the addictive properties of nicotine.Symptoms of withdrawal include inability to concentrate and agitation. Cocaine and Amphetamine are both powerful dopamine agonists. This is why they are among the most addictive drugs known. A single dose of cocaine is sufficient to produce addiction in mice. Cocaine acts as a dopamine reuptake inhibitor, whereas amphetamine and methamphetamine stimulate dopamine and norepinephrine release and inhibit their reuptake. The result is higher activity at dopaminergic synapses. At lower doses, these drugs produce alertness, elevated mood, confidence, and a sense of well-being. At higher doses, these drugs can produce symptoms that are similar to schizophrenia (e.g., hallucinations, such as feeling bugs on the skin, and paranoid delusions, such as thinking that others wish to harm). In some cases, users might show repetitive motor behaviors, particularly chewing movements or grinding of the teeth, leading eventually to loss of teeth. Cocaine was originally used by the indigenous people of Peru as a mild stimulant and appetite suppressant. Sigmund Freud believed that cocaine was an antidepressant, before becoming aware of its potential for addiction. The original formulation of Coca-Cola included extracts of the coca leaf. When cocaine was designated as an illegal substance, Coca-Cola simply substituted caffeine to compensate for the missing stimulant in its secret formula. During the past 50 years, amphetamine has been widely used by pilots and military personnel to ward off fatigue. More recently, methamphetamine has emerged as a cheap drug of abuse, leading to extensive neural damage (Barr et al., 2006). MDMA,MORPHINE,CODEINE,HEROIN AND ENDORPHINS 1. MDMA (or Ecstasy) is a relative of amphetamine: it increases sociability by stimulating the release of serotonin and the neurohormone oxytocin. MDMA use, though, destroys serotonergic neurons (Capela et al., 2007), thereby causing depression (Falck et al., 2008). MDMA also leads to memory deficits (Ward, Hall, & Haslam, 2006). 2. GHB is similar in structure to GABA. Because of its ability to mimic the inhibitory effects of GABA, it produces alcohol-like intoxication ad it is also known as a “date rape” drug due to its sedative action. 3. Morphine and Codeine are natural psychoactive substances found in the sap of the opium poppy, known as opiates. Heroin is synthesized from morphine. At low doses, opiates have several medical purposes, including pain relief, lack of anxiety, muscle relaxation, sense of euphoria, and sleep. With higher doses, opiates depress respiration, potentially leading to death. Notably, we have naturally occurring substances similar to opiates: the so called endogenous morphines, or endorphins (Hughes et al., 1977). These endorphins probably help us manage emergency situations in spite of extreme pain. Opiates mimic the effects of our bodies’ own endorphins at the opiate receptors. unit 3 → SYSTEM OF A REWARD The defining feature of an addiction is the compulsive craving for drug effects or other experience (either physical or psychological). “Every form of addiction is bad, no matter whether the narcotic be alcohol or morphine or idealism.” (Carl Gustav Jung) A likely basis for addiction is the ability of a drug to stimulate our natural neural system of reward, which we experience as feelings of pleasure. This system rewards us for engaging in behaviors that are important either for our personal survival or for the survival of the species. When we eat, drink, or engage in sexual behavior, the same reward circuits of the brain are activated. Even winning money activates the brain’s reward circuits (Breiter et al., 2001). These behaviors produce activity in dopamine circuits in the brainstem and in the nucleus accumbens. DOPAMINE Many addictive drugs share the ability to stimulate intense and long-lasting dopamine release (Volkow et al., 2004). In fact, research evidence shows that an interruption to the dopaminergic pathways connected to the nucleus accumbens reduces the self- administration of drugs by addicted animals. You might be wondering why these techniques are not used to help humans as well. Addiction cannot be treated in this manner because damaging these general reward circuits could deprive people of all pleasure. Notably, dopamine is not only associated with reward, but also plays a role in attention, motivation and inhibition. Disrupted dopamine activity due to repeated drug abuse results in lower levels of frontal lobe activity, which in turn can account for the lack of inhibition seen in addicts. This is the reason why new treatment frontlines are using TMS on the frontal lobe to cure some form of addiction. Once an addiction has been established, it is remarkably difficult to end it. Methadone is frequently used to wean heroin addicts away from their addiction. CANNABIS Like our natural opiate receptors, the brain also contains cannabinoid receptors that interact with the major active ingredients found in Cannabis. Cannabis contains over 50 psychoactive compounds, known as cannabinoids. Cannabinoids are implicated in pain, appetite, learning, and movement. The most important of these is THC, which acts as an agonist for endogenous cannabinoids, substances produced within the body that are very similar to THC in chemical composition. Cannabis has a long human history. It was included in the pharmacy written by Chinese emperor Shen Neng nearly 5,000 years ago and appeared in Europe when Napoleon’s soldiers brought it from Egypt. Marijuana, the smoked form of cannabis, is the most commonly used illegal substance in the United States today. The behavioral effects of cannabis are variable: most individuals experience mild euphoria, others experience social withdrawal. At higher doses, cannabis produces hallucinations. Marijuana distorts a person’s sense of time, distance, and speed. Within 2 hours of smoking, impairment of driving ability is similar to people that are legally too drunk to drive (Ramaekers et al., 2006). THC has also the ability to produce dopamine release at the nucleus accumbens, therefore it has the potential to produce dependency (Merritt et al., 2005). Proponents of medical marijuana argue that the drug alleviates symptoms of glaucoma, AIDS, and chemotherapy related weight loss and a variety of neurological and muscular disorders. Many physicians, however, do not recommend marijuana for their patients due to its potential for dependence. ALCOHOL Alcohol is one of the earliest drugs used by humans, dating back into our prehistory. At lower doses, alcohol reduces anxiety, promotes assertiveness, and reduces behavioral inhibitions. At higher doses, however, assertiveness becomes aggression, and motor coordination drops can lead to risky behaviors. At very high doses, coma and death can result from suppression of respiration. Alcohol acts as an agonist at the GABA receptors, which normally produce neural inhibition, leading to antianxiety and sedative effects similar to those caused by the benzodiazepines. Alcohol also stimulates dopaminergic pathways, which explain the euphoric and highly addictive qualities of the drug. Alcohol produces rapid tolerance due to the increase in the production of liver enzymes that eliminate alcohol from the system and to the changes in receptor number and characteristics. These changes produce a life-threatening withdrawal syndrome. The person will experience nausea, vomiting, sleeplessness, and anxiety. Chronic use of alcohol damages several areas of the brain, including the frontal lobes, which are responsible for higher-order cognitive functions. Alcoholism can impair the ability to form new memories (Korsakoff ’s syndrome) and the lack of vitamin B1 leads to damage to the hippocampus. Alcohol can also have devastating effects on the developing fetus. lesson 4 → development of the nervous system unit 1 → NATURE OR NURTURE Are our personality traits the result of experience or do they have strong roots in genetics? One thing's for sure, the tremendous increase in our understanding of the human genome has brought an increase of genetic testing. Couples ask for screening for the odds of producing a child with a particular genetic disorder. Embryos to be used for assisted reproductive technologies are screened for genetic abnormalities and, in some cases, for the desired sex of the child. Adults can be screened for their genetic vulnerability for certain cancers, for Huntington’s disease, and even for Alzheimer’s disease, a degenerative and fatal condition marked initially by memory loss. Did you know that the SNPs alleles can reliably predict a person’s risk for Alzheimer’s disease? Alleles whose genetic code differs in only one location are known as single nucleotide polymorphisms, or SNPs (pronounced “snips”). A person with two E4 genes has a 91 percent chance of developing Alzheimer’s disease at the age of 68 years. GENOTYPE AND PHENOTYPE The human genome contains two copies of 23 chromosomes, made up of DNA. One chromosome of each pair is donated by the mother and the other by the father. This personal set of genetic instructions is called genotype. DNA sequences, or genes, provide instructions for producing proteins. Notably, alternative versions of a gene, or alleles, are either dominant or recessive. According to the rules of dominance, one person needs two copies of a recessive gene or only one copy of a dominant gene to produce the trait in the organism: the phenotype. The study of identical and fraternal twins raised together or apart by adoptive parents provides one classical technique for comparing the relative contributions of genetics and environment. Studies comparing identical, or monozygotic, and fraternal, or dizygotic, twins raised together or apart by adoptive parents are particularly useful in sorting out the relative contributions of heredity and environment. Monozygotic (MZ) twins share the same genes, whereas dizygotic (DZ) twins share the same number of genes (about 50%) as ordinary siblings. All twins, however, share a similar environment, both before and after birth, whereas ordinary siblings who are born at different times experience greater variations in their environments. GROWTH OF NS The cell formed by the merger of egg and sperm is known as a zygote. After the second week following conception, the developing individual is an embryo. After the eighth week, the individual is a fetus. One week following conception, the human embryo divides into three bands of cells. The outer layer is the ectoderm, which will develop into the nervous system. During the third week, cells in the ectoderm begin to differentiate into a new layer known as the neural plate, with two ridges of tissue that eventually join to form the neural tube. The interior of the neural tube will be retained in the adult brain as the system of ventricles and the central canal of the spinal cord. The surrounding neural tissue will form the brain and spinal cord. SPINA BIFIDA In spina bifida, the caudal portion of the neural tube fails to close normally, leading to paralysis of the lower limbs. Deficiencies of folic acid might be responsible for a large number of cases. Folic acid occurs naturally in dark green leafy vegetables, egg yolks, fruits, and juices. However, the diet might not contain sufficient levels of folic acid to prevent neural tube defects (for instance, the American diet). Consequently, it is often added to fortified breakfast cereals as a preventive measure. Unfortunately, folic acid fortification might lead to increased risk for colon cancer. USE IT OR LOSE IT The development of the mature nervous system proceeds in a series of six distinct stages: 1. continued birth of neurons and glia; 2. migration of cells to their eventual locations in the nervous system; 3. differentiation of neurons into distinctive types; 4. formation of connections between neurons; 5. death of particular neurons; 6. rearrangement of neural connections. As concern step 5 & 6, it is crucial to note that a significant number of new neurons die during the development process. And synapses follow a similar pattern of overproduction followed by pruning. Why is it so? The nervous system operates according to a “use it or lose it” philosophy, meaning that one must continually engage in and practice an ability or risk losing it. The "use it or lose it" philosophy makes a lot of sense and recent advances in brain science have provided clear evidence that the brain continuously shrinks and expands depending on our experiences. Although many parts of the brain retain the ability to change throughout the lifetime, some processes, including vision and language learning, have critical periods in which change and learning occur. After this segment of time experience has little or no effect. FORMATION OF SYNAPSES To wire the nervous system, new axons must identify appropriate postsynaptic cells. The process can be observed easily at the neuromuscular junction. Initially, the receptors are evenly distributed within the membrane of the muscle fiber. Then, chemical release by both the presynaptic and postsynaptic structures stimulates movement of the receptors to the synaptic site and development of the motor axon terminal. Once the synapse is mature, the receptors are tightly clustered at synaptic sites. CELL DEATH AND SYNAPTIC PRUNING During the course of development, large numbers of cells die in the process known as apoptosis, or programmed cell death. The term apoptosis comes from the Greek word for “falling leaves.” Cohen, Levi- Montalcini, and Hamburger (1954) isolated a substance they called nerve growth factor (NGF). Axons absorb NGF released by target cells and compete for the limited quantities of NGF. Neurons that fail to receive adequate amounts of NGF experience apoptosis. Synaptic Pruning → During the course of development, we also lose large numbers of synapses. The brain initially overproduces synapses, then synaptic growth is followed by a period of synaptic pruning, in which the number of functional synapses is reduced. Only those synapses that participate in functional neural networks are maintained. The nervous system operates according to a “use it or lose it” philosophy. unit 2 HOMOSEXUALITY The field of psychology has extensively studied homosexuality as a human sexual orientation. The APA listed homosexuality in the "Diagnostic and Statistical Manual of Mental Disorders" (DSM-I) in 1952, but it was removed in 1973, thus removing the stigma of mental illness that has long been associated with homosexuality. Homosexuality is now considered a natural and normal sexual orientation that is a healthy and positive expression of human sexuality. In the 20th century, people began studying homosexuality scientifically and theories about the genetic and hormonal origin of homosexuality were becoming accepted. CAUSES OF HOMOSEXUALITY Although no theory on the cause of sexual orientation has yet gained widespread support, scientists favor biologically-based theories. There is considerably more evidence supporting nonsocial, biological causes of sexual orientation than social ones, especially for males. Scientists theorize that homosexuality is caused by a complex interplay of genetic, hormonal, and environmental influences. The most recent literature says that sexual orientation is not a choice that can be changed at will, and that sexual orientation is most likely the result of a complex interaction of genetic, hormonal, environmental, cognitive and biological factors. These factors, which may be related to the development of a sexual orientation, include the early uterine environment (such as prenatal hormones), and brain structure (Bailey et al., 2016) INFLUENCE OF HORMONES The influence of hormones on the developing fetus has been the most influential causal hypothesis of the development of sexual orientation (Bailey et al., 2016; Balthazart 2011) The presence of the Y-chromosome in males prompts the development of testes, which release testosterone, the primary androgen receptor-activating hormone, to masculinize the fetus and fetal brain. It has been hypothesized that gay men may have been exposed to little testosterone in key regions of the brain, or had different levels of receptivity to its masculinizing effects, or experienced fluctuations at critical times. In women, it is hypothesized that high levels of exposure to testosterone in key regions may increase likelihood of same sex attraction. Supporting this hypothesis are studies of the finger digit ratio of the right hand, which is a robust marker of prenatal testosterone exposure. Lesbians on average have significantly more masculine digit ratios, a finding which has been replicated numerous times in studies cross-culturally (Breedlove, 2017) SEXUALLY DIMORPHIC NUCLEUS The sexually dimorphic nucleus of the preoptic area (SDN-POA) is a key region of the brain which differs between males and females in humans. Differences are caused by sex differences in hormone exposure. The INAH-3 region is bigger in males than in females, and is thought to be a critical region in sexual behavior. Dissection studies found that gay men had significantly smaller sized INAH-3 than heterosexual males, which shifted in the female typical direction, a finding first demonstrated by neuroscientist Simon LeVay, which has been replicated. This highlights the role of prenatal hormones in masculinization of the brain for sexual attraction. GENETIC INFLUENCES Multiple genes have been found to play a role in sexual orientation. Identical twins are more likely to have the same sexual orientation than non-identical twins. This indicates that genes have some influence on sexual orientation; however, scientists have found evidence that other events in the womb play a role. A number of twin studies have attempted to compare the relative importance of genetics and environment in the determination of sexual orientation. In a 1991 study, Bailey and Pillard found that 52% of monozygotic (MZ) brothers and 22% of the dizygotic (DZ) twins were concordant for homosexuality. Dizygotic twins have separate amniotic sacs and placentas, resulting in different exposure and timing of hormones. CHROMOSOME STUDIES Chromosome linkage studies of sexual orientation have indicated the presence of multiple contributing genetic factors throughout the genome. In 1993, Dean Hamer and colleagues published findings from a linkage analysis of a sample of 76 gay brothers and their families. Hamer et al. found that the gay men had more gay male uncles and cousins on the maternal side of the family than on the paternal side. Results from the first large genetic linkage study of male sexual orientation were reported by an independent group of researchers at the American Society of Human Genetics in 2012. The study population included 409 pairs of gay brothers, who were analyzed with over 300,000 single-nucleotide polymorphism markers. Significant linkage was detected in the distal region of Xq28 and in the pericentromeric region of chromosome 8. The authors concluded that "our findings suggest that genetic variation in each of these regions contributes to development of the important psychological trait of male sexual orientation". unit 3 EFFECT OF EXPERIENCE ON DEV. Prior to the availability of imaging technologies, researchers believed that the majority of human brain development took place up to the age of 2 years. Now we know that a second wave of gray matter development and myelination begins at puberty, peaking at about age 11 until the age of 25. This burst of new growth especially affects the frontal lobes, influencing abilities to plan, control impulses, and reason. Although the numbers of neurons added to the mature brain are quite small compared with neurogenesis early in development, these new neurons may play a very important role in the modification of the brain in response to experience. HEBB SYNAPSES Throughout the lifespan, we retain the ability to rearrange our synaptic connections. This adjustment occurs as a result of the strengthening or weakening of existing synapses due to experience. In general, synapses are strengthened when the pre- and postsynaptic neurons are simultaneously active and weakened when their activity is not synchronized. The importance of correlated activity in strengthening synapses was first suggested in the 1940s by Donald Hebb. As a result, synapses strengthened by simultaneous activity are often referred to as Hebb synapses. CRITICAL PERIOD David Hubel and Torsten Wiesel (1965, 1977) demonstrated how experience with the sensory environment could influence the development of the visual cortex. In the kitten subjected to monocular deprivation, the right eye was sutured closed for the first three months of life. Then the right eye was opened, and the left eye was sutured closed for the next three months. Subsequent recordings showed that the reversal of open eyes had little impact on the organization of the kitten’s visual cortex. Most cells continued to respond only to light falling in the left eye, which had been open during the first three months of life. Hubel and Wiesel argued that these data support the existence of a critical period during which the visual cortex can be modified by experience. This is why corrective surgery for vision must take place as early as possible in young children. EXPERIENCE AND BEHAVIOUR In 1952 Konrad Lorenz described the phenomenon of imprinting. If a newly hatched chick saw Lorenz right away instead of the mother bird, it treated Lorenz as its mother. As in the visual system, imprinting shows a critical period, a window of time in which experience will modify behavior. Imprinting in baby geese only occurs during the first two days of life but not at later ages. Very few people who learn a second language after puberty will be able to pass as native speakers. Several hypotheses have been proposed to account for the closing of critical periods. Growth spurts in myelin have been observed in parts of the brain involved with language only between the ages of 6 and 13 years (Thompson et al., 2000). The presence or absence of neurotrophins might also influence the timing of critical periods. AFFECTIVE DEPRIVATION Human intellectual development is also subject to critical periods. Children raised in orphanages have very few opportunities to interact with other people or the environment and they recover from their earlier deprivation only when adopted prior to 6 months of age. → article “impact of childhood maltreatment on the recognition of facial expressions of emotions” DISORDERS OF BRAIN DEV. Abnormal development can occur due to: neural tube defects (in spina bifida the neural tube fails to close normally) genetic disorders environmental causes Genetic Disorders → A wide variety of genetic errors can occur in development. For example, Trisomy 21, also known as Down syndrome, is characterized by an individual having three, rather than the normal two, copies of chromosome 21. The major cause is abnormal division of the mother’s twenty-first chromosome during meiosis. Disjunction is related to maternal age, so rates of Down syndrome increase from 1 out of 1,000 births to mothers under 33 years of age to 38 out of 1,000 to mothers over 45. ENVIRONMENTAL CAUSES A frequent cause of mental disability is maternal alcohol use. Children with fetal alcohol syndrome experience growth retardation, and reduced IQ. They are more likely to be diagnosed with attention deficits, poor impulse control, and severe behavioral problems. RESPONSE TO DAMAGE Any damage to the cell body of a neuron will result in neural death. Damage to an axon, instead, might have different outcomes depending on the lesion site. In anterograde degeneration, the segment of the axon separated from the cell body will die. On occasion, the remaining segment, still attached to the cell body, will resprout and regrow to its former targets. Typically, damage that is very close to the cell body is more likely to produce retrograde degeneration, in which the axon stub and the cell body degenerate and die. The death of a neuron has repercussions among the other neurons with which it was formerly connected. Without input from the now-dead cell, postsynaptic cells deteriorate due to transneuronal degeneration. Damaged axons in the peripheral nervous system can regrow successfully, due at least in part to the response of the Schwann cells. Axons resprout and are guided back to their original destination by the template formed by Schwann cells and by adhering to the extracellular environment. Unfortunately, the response of the oligodendrocytes to damage in the central nervous system is not helpful. Although most central nervous system damage is permanent, promising new treatments, such as the implantation of stem cells, may be available soon (Bambakidis et al., 2008). ALZHEIMER’S DISEASE Alzheimer’s disease is a degenerative condition associated with aging that results in loss of normal cognitive and emotional function. Behaviorally, the condition usually begins with mild memory loss. As the disease progresses, problem solving, language, and social behavior deteriorate. The emergence of abnormal structures known as neurofibrillary tangles is one of the hallmark features of the disease. Tangles result from the detachment of the tau protein. Without tau, the microtubules do not maintain their structure, and the neuron basically collapses. Transneuronal degeneration spreads deterioration rapidly throughout the brain. The memory impairment probably results from deterioration in the hippocampus. Language and problem-solving deficits arise from damage to the association areas of the cortex. The amygdala and other limbic system structures connected to the hypothalamus are also affected, leading to emotional changes. Currently, there are no effective treatments for reversing the course of Alzheimer’s disease. Because one of the characteristics of Alzheimer’s disease is a decrease in cholinergic activity in the brain, medications that boost acetylcholine levels or activity might be helpful. lesson 5 → movement lesson 6 → mirror neuron system lesson 7 → emotion lesson 8 → stress lesson 9 → psychological disorders