BOTANY 20 - Lecture PDF
Document Details
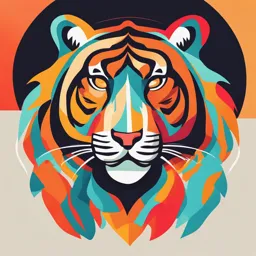
Uploaded by ProlificElegy2706
University of the Philippines Los Baños
Tags
Summary
This document contains lecture notes for a botany course. The topics covered include plant physiology, plant cell types, tissue systems, respiration, and secondary metabolites. It also presents the role of plant parts, and the functions of secondary metabolites.
Full Transcript
BOTANY 20 - Lecture Introduction to Plant Physiology o Pollen for fertilization o Naked seeds I. Plant Physiology o i.e. Ginkgo Dyna...
BOTANY 20 - Lecture Introduction to Plant Physiology o Pollen for fertilization o Naked seeds I. Plant Physiology o i.e. Ginkgo Dynamic processes in plant life – growth, Angiosperms metabolism, and reproduction. o Vascular Crop physiology – applied plant o Diploid dominance physiology in genetically similar o Pollen for fertilization cultivated plants. o Seeds inside an ovary II. Importance of Plant Physiology o i.e. Monocots Efficient use of nutrients. IV. Basic Requirements of Plant Life Coping with biotic and abiotic stresses – Light drought, pollution, pests, weeds. Carbon Dioxide Increase crop yield. Water Increase food and feed quality. Minerals III. Kingdom Plantae V. Unifying Principles of Plant Life Photoautotrophs Cellulosic cell wall Sedentary Avoids desiccation Transport processes Oxygen production VI. The Plant Body Bryophytes o Non-vascular o Haploid dominance o Requires water for fertilization o Seedless – spores o i.e. Liverworts Ferns o Vascular VII. Plant Cell Types o Diploid dominance Parenchyma o Requires water for fertilization Collenchyma o Seedless – spores Sclerenchyma o i.e. horsetails Gymnosperms o Vascular o Diploid dominance o From parenchyma cells o Alive at maturity o Primary and secondary cell wall o i.e. strings in celery Parenchyma Cell o Generic o Abundant and versatile o Function: Storage and Metabolism o Capable of differentiation o Alive at maturity o Primary cell wall Sclerenchyma Cells o Rigid o Function: Support and strengthen non extending regions of plants. o Thick, non-stretchable secondary cell walls. o Dead at maturity. o Two types: ▪ Fibers – long, slender, strands ▪ Sclerids – short, varying shape, groups Collenchyma Cell o Glue o Flexible support o In growing plant organs VIII. Plant Tissue Systems X. Complex Tissues: Vascular Tissues Xylem – water and ion transport. Consists of tracheids and vessel members. Lignin-filled. Phloem – sugar and organic solute transport. Consists of sieve tubes and companion cells. Ground tissue – photosynthesis, storage, and structural support. Vascular tissue – distributes absorbed water, mineral ions, and products of photosynthesis. Dermal tissue – covers and protects exposed plant surfaces. XI. Complex Tissues: Dermal Tissues IX. Simple Tissues: Ground Tissues Epidermis – single outer layer with a Parenchyma – makes up most primary waxy protective cuticle. Contain growth. For secretion, storage, specialized cells for stomates. photosynthesis, and repair. Periderm – epidermis in woody plants. Collenchyma – supports growing plant parts. Pectin for flexibility. Sclerenchyma – contains lignin for support. Cells are dead at maturity. XII. Other Plant Tissues Apical Meristems – shoot and root tips. Increases plant length. Intercalary Meristems – adds length to the plant. 3 primary meristems o Ground meristem – ground tissues o Protoderm – skin coverings o Procambium – plant plumbing Lateral Meristems – adds girth by producing wood and bark. XIII. Vegetative Organs Stems Roots o Supports leaves o Anchor o Conduit for transport o Water and mineral absorption o Storage o Storage o Woody Dicots ▪ Discrete vascular bundles replaced by continuous rings of xylem. ▪ Each ring is xylem produced during one growing season. ▪ Vascular cambium ▪ Taproot is present ▪ Flowers in multiples of four or five Stems: Secondary Growth o Vascular tissue (xylem) makes up the bulk of the stem. o Form tree rings. Leaves o Main photosynthetic structure o Monocotyledon ▪ One cotyledon ▪ Parallel veins ▪ Vascular bundles usually complexly arranged ▪ Fibrous root system ▪ Flowers in multiples of three o Dicotyledon ▪ Two cotyledons ▪ Netlike veins ▪ Vascular bundles in rings Introduction: The Plant Cell II. Cell Wall of Mature Cell I. Plant Cell Wall III. Plasma Membrane Selective permeability of membranes Endoplasmic Reticulum Golgi Apparatus IV. Different Parts of the Plant Cell Nucleus o Control centre o Storage of genetic information o The Genome: all the genetic material in a cell o Nuclear pore – passage between nucleus and cytoplasm Endomembrane System Endosymbiotic Hypothesis for the Origin Vacuole of Mitochondria and Chloroplast o Stores water, ion, and nutrients o Receptacle for waste products o Turgor pressure regulation by osmosis. Mitochondria o Powerhouse of the cell o ATP production o Cellular respiration o Has its own genome Plastids o Chloroplasts – chlorophyll, photosynthesis. Leaves and stems. ▪ Thylakoids – area of photosynthesis ▪ Stroma – calvin cycle o Amyloplasts – starch storage, colourless. Roots, seeds, and Vesicles and Microbodies fruits. o Vesicles – store or transport o Chromoplasts – red and yellow substances. pigments. Petals and fruits. o Peroxisomes – has enzymes that break down hydrogen peroxide, and other toxins. o Glyoxysomes – break down fats. Cytoskeletons o Network of protein fibers. o Functions: ▪ Internal support ▪ Internal structure ▪ Transport of organelles and protein vesicles ▪ Cell motility Introduction: Energy and Thermodynamics III. Free Energy (Gibbs Free Energy) Measure of energy for the performance I. Energy of work. Energy – capacity to do work ΔH = ΔG + TΔS Potential Energy – stored; at rest; i.e. o Free energy, ΔG, is the energy chemical. available to do work Kinetic Energy – accomplishing work; o Spontaneity of the reaction is motion; i.e. heat or light ΔG, if 0 it is endergonic Generally: energy of the universe is o Spontaneity depends on both constant, but that entropy continues to enthalpy (total energy content increase toward a maximum of system; equivalent to ΔH for 1st Law of Thermodynamics our purposes) and entropy. o Total energy in the universe is ΔG = ΔH – TΔS (Gibbs Free Energy) constant. o Negative ΔG – spontaneous o Energy can be changed from one process: exergonic. Toward form to another but cannot be equilibrium, energy releasing. created not destroyed. o Positive ΔG – non-spontaneous nd 2 Law of Thermodynamics process: endergonic. Away from o The natural tendency of the equilibrium, energy consuming. universe is towards increasing disorder. o The total entropy increases and energy converts to less organized form. o In all energy exchanges and conversions, if no energy leaves or enters the system under study, the potential energy of the final state will always be less than the potential energy of the initial state. IV. Energy Coupling o If potential energy of final state Catabolic processes – degradative, is < initial = exergonic usually oxidative energy yielding o If potential energy of final state (exergonic). is > than initial = endergonic Anabolic processes – synthetic usually o Entropy (S) – measurement of reductive energy utilizing (endergonic). disorder V. An Overview of Metabolism o Spontaneous – events that occur Catabolic pathways – breakdown without the input of external complex substrates into simple end energy. products. o Provides raw materials o Provides chemical energy Anabolic pathways – synthesize complex end products from simple substrates. o Require energy o Use ATP and NADPH VI. Enzymes Biological catalysts, agents of life. Properties of Enzymes: o Specificity – acts on single IX. Enzyme Structure substrate. Primary – amino acid sequence. o Catalytic efficiency – 108-1020 Secondary – local structural units held by times over uncatalyzed reaction. H-bond. o Mostly reversible – except for Tertiary – 3D shape of polypeptide decarboxylases and hydrolases. (subunit). VII. Enzyme Properties and Composition Quaternary – association of two or more Highly specific protein catalyst. subunits. Has active site – uniquely shaped permits specificity. Catalysis occurs at the active site composed of the binding site and catalytic group. Binding site attracts and positions the substrate. Catalytic group – reactive side chains of amino acids. Carry out bond- breaking/forming reactions. VIII. Enzyme Composition Non-protein component o Prosthetic group – tightly bound; organic molecule; more or less permanently associated with the enzyme protein. (i.e. sugar) o Cofactor – not tightly bound; transiently associated with the protein. ▪ Metal activator – metal ion. (i.e. Zn, Fe, Cu, Mo) ▪ Coenzyme – organic molecule (i.e. NAD, FAD, FMN, vitamins) X. Mechanism of Enzyme Action XII. Model of Enzyme-Substrate Interaction Enzyme catalysis proceeds as follows: o E+S -> E-S -> E+P Enzymes lower the barrier of activation energy. o Unit of energy needed to start a reaction; free energy barrier between substrates and products. XIII. Kinetics of Enzyme-Catalysed Reaction Hyperbolic relationship between reaction velocity (v) and substrate concentration (S), Where reaction rate becomes independent of substrate concentration when the enzyme becomes saturated with the substrate. The rate remains relatively constant (steady state) until the substrate is depleted. XI. Catalytic Cycle of Enzyme Reaction rates depend on the number of successful collisions between substrate and enzyme molecules which in turn depend on concentration. Doubling the enzyme concentration usually doubles the reaction rate given enough substrate. Further addition of enzymes brings the rate to a constant, as substrate is limiting. At any given [E], reaction rate reaches a XV. Environmental Factors Affecting Enzyme maximum at a certain [S], as the enzyme Activity become limiting, thus no more enzyme Temperature sites are available for catalysis. The [S] required to halve the maximum reaction rate is Km value. XIV. Enzyme Inhibitor pH Inhibitors – molecules preventing o Changing pH influences the enzymes from maximum turnover ionization of the substrate numbers. resulting in the loss of H- o Active Site Directed Inhibition – bonding inhibitors competing with the o Denaturation state substrate for the active site. o Presence of charged or o Non-active Site Directed uncharged amino or carboxyl Inhibition – inhibitors that affect group active site shape by binding onto the enzyme. ▪ Neutral carboxyl requires low pH optimum ▪ Uncharged amino group needs high pH optimum [S] Entropy XVI. Enzyme Activity is Often Regulated Cells can control the flux of metabolites through: o Gene expression ▪ regulates enzyme activity through the amount or concentration of enzymes present in the cell --- determined by relative rates of enzyme synthesis and degradation o Compartmentation ▪ localizes enzymes with different catalytic properties in the different parts of the cell enzymes involved in PS are found in chloroplast hydrolases are found in vacuoles o Covalent modification ▪ regulates enzyme activity through phosphorylation --- phosphorylated form is active while non- phosphorylated form is inactive o Feedback inhibition ▪ enzyme activity is regulated by the end products of the metabolic pathways which inhibit the enzymes involved in earlier steps. Plant Respiration o Electron Transport System I. Aerobic Respiration Higher plants – aerobic organisms; need oxygen for normal metabolism. Obtain energy and carbon by oxidizing photoassimilates. III. Mitochondrial Structure and Function Mitochondrial membranes enclose two spaces: the matrix and intermembrane space. II. Respiration: An Overview Outer mitochondrial membrane as outer Direct oxidation of hexose by molecular boundary. oxygen while releasing free energy as Inner mitochondrial membrane is heat. subdivided into: By breaking the oxidation of hexose into o Inner boundary membrane small steps, energy release is controlled o Cristae – machinery for ATP is and conserved in metabolically useful located. forms. Provides the carbon skeleton for a number of plant products: o Amino acids for proteins o Nucleotides for nucleic acids o Carbon precursors for fats o Porphyrin pigments o Aromatic compounds (lignin) Products are utilized for plant growth and maintenance. In growth respiration, ATP, NADPH/NADH or structural building blocks of carbon skeletons are used for synthesis of new plant biomass. In maintenance respiration, products are used for repair and maintenance of membrane proteins and ion gradients to Mitochondrial membranes: keep cells mature and viable. o Outer Mitochondrial Membrane Takes place in 3 stages: ▪ 50% protein o Glycolysis o Krebs Cycle ▪ Contains pore-forming protein called porin. ▪ Permeable to even some proteins. o Inner Mitochondrial Membrane ▪ 75% protein ▪ Contains cardiolipin. ▪ Impermeable to small molecules. The Mitochondrial Matrix o Contains circular DNA molecule, ribosomes, and enzymes. o RNA and proteins can be synthesized in the matrix. IV. Glycolysis Converts sugars to pyruvate. Takes place in the cytosol. ATP is synthesized through substrate level phosphorylation. ATP synthesis is catalyzed by an enzyme and involves the transfer of phosphate group of an organic substrate molecule to ADP. V. Glycolysis: Functions Partial oxidation of hexose (2 molecules of pyruvic acid/hexose) → 2 molecules of NAD is reduced to NADH (+2H+) that uses no O2 and releases no CO2 Produces ATP (hexose is twice phosphorylated by ATP (net: 2ATP) Leads to formation of molecules that can be removed from the pathway for synthesis of other compounds. Pyruvate can be synthesized in the mitochondrion and yield larger ATP. When O2 is limiting: NADH and pyruvate accumulates → fermentation VI. Glycolysis: Overview o Ethanol (Alcoholic fermentation) The first steps in oxidative metabolism o Lactic acid (Lactic Acid are carried out in glycolysis: fermentation) o Glycolysis produces: i.e. roots growing in waterlogged ▪ Pyruvate conditions. ▪ NADH ▪ 2 ATP o Aerobic organisms use O2 to extract more than 30 additional ATPs from pyruvate and NADH. o Pyruvate is transported across the inner membrane and decarboxylated to form acetyl CoA, which enters the next stage. VII. Krebs Cycle or Tricarboxylic Acid Cycle Stepwise cycle where substrate is oxidized (loss of an electron) and its energy conserved. The 2-carbon acetyl group from acetyl CoA is condensed with the 4-carbon oxaloacetate to form a 6-carbon citrate. During the cycle, 2 carbons are oxidized to CO2, regenerating the 4-carbon oxaloacetate needed to continue the cycle. 4 reactions in the cycle transfer a pair of electrons to NAD+ to form NADH, pr to FAD+ to form FADH2 One ATP is formed though substrate level phosphorylation when succinyl CoA is converted to succinic acid Reaction intermediates in the TCA cycle are common compounds generated in other catabolic reactions making the TCA cycle the central metabolic pathway of the cell. Three molecules of ATP are formed from each pair of electrons donated by NADH; two molecules of ATP are formed from each pair of electrons donated by FADH2. VIII. TCA/Krebs Cycle: Functions harnessed to make ATP from Reduction of NAD and Fad to electron ADP + Pi through the ATP donor NADH and FADH2 – these are synthase in the process called – subsequently oxidized to yield ATP chemiosmosis. during Electron Transport System Direct synthesis of ATP – 1 molecular of each pyruvate is oxidized. Formation of carbon skeletons for the synthesis of certain amino acids. IX. The Role of Mitochondria in the Formation of ATP Electron Transport System o Electrons move through the inner membrane via a series of carriers of decreasing redox potential. o Electrons associated with either Electron Transport Complexes NADH or FADH2 are transferred o Complex I through specific electron ▪ NADH dehydrogenase carriers that make up the ETS. ▪ Catalyzes transfer of o The NADH from Glycolysis and electrons from NADH to Krebs Cycle, and FADH2 from ubiquinone and Krebs Cycle are oxidized – transports 4 H+ per pair. producing ATP. o Complex II o The oxidation of NADH involves ▪ Succinate O2 uptake dehydrogenase o Electrons are transferred ▪ Catalyzes transfer of through electron carriers before eelctrons from H2O is produced. succinate to FAD to o The electron carriers are ubiquinone without arranged in 4 major protein transport of H+ complexes in the inner o Complex III mitochondrial membrane. ▪ Cytochrome bc1 o The electrons gradually lose ▪ Catalyses the transfer of energy as they pass along the electron from chain of electron carriers. ubiquinone to o The released energy pumps H+ cytochrome c and into the intermembrane space, transports 4 H+ per pair. creating an H+ or pH gradient o Complex IV across the membrane. ▪ Cytochrome C Oxidase o The free energy associated with ▪ Catalyses transfer of the formation of the proton electrons from O2 and electrochemical gradient is transports H+ across the called – proton motive force. It is inner membrane. o Cytochrome oxidase – a large XIV. Oxidative Metabolism in the complex that adds 4 electrons to Mitochondrion O2 to form 2 molecules of H2O The importance of Reduced Coenzymes o The metabolic poisons CO, N3-, o Step 1: High energy electrons and CN- bind catalytic sites in are passed from FADH2 or NADH Complex IV. ▪ As electrons move though the electron- transport chain, H+ are pumped out across the inner membrane. o Step 2: The controlled movement of protons back across the membrane. ▪ ATP is formed by the controlled movement of H+ back across the membrane through the ATP-synthesizing Chemiosmotic Phosphorylation enzyme. XV. Summary of Oxidative Phosphorylation Substrate Level Phosphorylation XVI. Unique Features of Plant Aerobic Respiration In Krebs Cycle o The succinyl-coA synthetase step produces ATP instead of GTP which the step produces in animals. o Significant NAD+-malic enzyme activity that enables the plant mitochondria to operate an alternative pathway for metabolism of PEP produced by glycolysis. In Electron Transport System o The availability of an alternative pathway for the reduction of O2 which leads to cyanide resistant respiration. o An external dehydrogenase that faces the intermembrane space and is capable of oxidizing cystolic NADPH. o A rotenone-insensitive NADPH dehydrogenase that faces the mitochondrial matrix. XVII. Other Pathways of Plant Respiration Aerobic respiration is strongly inhibited by certain negative ions such as cyanide, azide, and carbon monoxide. Combined with iron in the cytochrome oxidase (Complex IV) prevents respiration. Cyanide-resistant respiration/Alternative Pathway Pentose phosphate pathway/ Hexose monophosphate shunt/ Oxidative pentose phosphate pathway/ phosphogluconate pathway XVIII. Alternative Pathway Electrons from ubiquinone are directly accepted by O2, via an alternative oxidase Occurs in leaves and roots Contributes to ATP synthesis when the cytochrome path is saturated (consuming carbohydrates not needed carbohydrates to cells --- excess for growth, energy overflow) supply over and above what is Bulk of heat has thermogenic required for metabolism or significance in pollination and in plant processed for storage. response to low temp. XIX. Pentose Phosphate Pathway Hexose monophosphate shunt; Oxidative pentose phosphate pathway; phosphogluconate pathway Pathway from glucose degradation where plants obtain energy from the oxidation of sugars in CO2 and H2O Electron acceptor is NADP instead of NAD Products: NADPH, erythrose-4- Phosphate, ribose-5-Phosphate Like glycolysis it occurs in cytosol Oxidation is achieved by dehydrogenation using NADP+, not NAD+ It is carried out in 2 steps: o Irreversible oxidative phase: Glu-6-P give rise to CO2 and 5- carbon sugars o Reversible non-oxidative phase: Rearranged to regenerate Glu-6- P and G3P Oxidative Phase o Dehydrogenation of Glu-6-P to 6-phosphogluconolactone Thermogenesis o Followed by conversion to 6- o Floral development in skunk phosphogluconate catalyzed by cabbage. enzyme Gluconolactonase o Tissues of the spadix undergoes o Decarboxylation follows with a surge in oxygen consumption, the formation of ribulose-5- called respiratory crisis. phosphate o Higher temp volatizes amines o Both steps require NADP+ as that attract insect pollinators. hydrogen acceptor Energy Flow Hypothesis o In most tissues the alternative pathway is inoperative until the normal cytochrome pathway has become saturated. o The rate can be increased by increasing the supply of. Non-Oxidative Phase o Ribulose-5-P is the substrate for two enzymes: ▪ Ribulose-5-phosphate isomerase: conversion to ribose-5-P - used for nucleotide and nucleic Pentose Phosphate Pathway acid synthesis o NADPH is oxidized to form ATP ▪ Ribulose-5-phosphate needed for fatty acid 3-epimerase: alters the biosynthesis; configuration about o Erythrose-4-phosphate as carbon giving xylulose 5- starting compounds for P production of phenolic o Xylulose-5-P and Ribose-5-P compounds like anthocyanins reacts in the presence of enzyme and lignin Transketolaseto give G3P and o Ribose-5-phosphate as Sedoheptulose-7-P precursor for ribose and o Acted by enzyme Transaldolase deoxyribose units in to give Fru-6-P and Erythrose-4- nucleotides, RNA and DNA P o In flooded soils where hypoxia (low O2) or anoxia (no O2) exists → high CO2 production and low ATP yield, with sugar used more as substrate – Pasteur effect (practical importance during storage) o Decreasing O2 can minimize aerobic respiration without stimulating anaerobic respiration Temperature o Respiration is temperature dependent o Quantitative measure to describe the effect of temp is the temperature coefficient or Q10 o At temp above 30°C, the Q10 in most plants begin to fall off as substrate availability becomes limiting o the O2 penetrating the cells begin to limit respiration at XX. Factors Affecting Respiration higher temp at which chemical Substrate Availability reactions could proceed rapidly o Plants deficient in sugars or low o When temp rises to 40°C, if substrate have low respiration prolonged, rate decreases as a rates result of enzyme denaturation. o Substrate starvation → Plant Type and Age proteins/fats are used o Plants exhibit differences in o Respiration rates of leaves are morphology and metabolism very fast at sundown → high → rate differs among various sugar levels due to accumulation plants and between cells, of photosynthates during the tissues, and organs day. o Root tips/meristematic tissues Oxygen Availability have higher respiratory rates on o Variations in the O2 content of a dry weight basis than non- air are too small to influence metabolic tissues (woody stems) respiration in leaves/stems o Age influences respiration: o The rate of O2 penetration in ▪ High during rapid plant organs are usually vegetative growth, sufficient to maintain normal declines before respiration levels in flowering mitochondria ▪ Young leaves, roots and growing flowers (high respiration) ▪ During fruit development (ripening)- increases; declines when picked ▪ “Climacteric rise” – coincides with the full ripeness and full flavor of fruits Membrane lipids occur in ER and mitochondrial membrane Fats and oils exist in the form of triglycerides (spherosomes - oleosomes or lipid bodies). XXII. Conversion of Fats and Oils to Sugars In the spherosomes, triglycerides are hydrolyzed to fatty acids and glycerol In the glyoxysome, FA are activated to fatty acyl-CoA which undergoes ᵦ- oxidation and becomes acetyl CoA The glyoxylate cycle generates succinate that moves to mitochondrion and is converted to malate Malate oxidized to oxaloacetate, decarboxylated to PEP and by reverse glycolysis (gluconeogenesis) in the cytosol produces glucose → sucrose XXIII. Gluconeogenesis a metabolic pathway that results in the generation of glucose from noncarbohydrate carbon substrates (i.e., XXI. Physiochemistry of Lipids lactate, glycerol and glucogenic amino Structurally diverse compounds soluble acids) in organic solvents but insoluble to water 3 key steps: irreversible Biosynthesis of fats, oils and other lipids o Conversion of pyruvate to PEP requires large investment of metabolic via oxaloacetate, catalyzed by energy PEPCase and PEPCK Fatty acid synthesis is localized in the chloroplast and proplastids of non-green tissues o Dephosphorylation of Fru-1,6- bipohospate by Fru-1,6- phosphatase o Dephosphorylation of Glu-6-P by Glu-6-phosphatase Secondary Metabolites III. Principal Groups of Secondary Metabolites (Biosynthetic) I. Primary Metabolites Products from plant’s vital processes. Terpenoids Found in all plants. From acetyl CoA via mevalonic acid Commercially important as food pathway sources. Examples: essential oils (menthol), Examples include: lycopene, limonene, saponin, taxol, o Carbohydrates – sucrose, carotenoid, sterol, rubber starch, cellulose. o Proteins – amino acids. o Lipids – fatty acids. o Nucleic acids o Chlorophyll II. Secondary Metabolites Metabolic diversion of a product from a major pathway. Restricted in distribution. Function in plant defense, pollination, and competition. Commercially important in medicines and industries. Phenolics From erythrose-4-phosphate and phosphoenol pyruvate via shikimic acid pathway Examples: simple phenolics (vanillin, IV. Functions of Secondary Metabolites salicylic acid), flavonoids Defend plants against herbivore and (anthocyanin, flavone and pathogen attack (primary function) isoflavonoid), lignin, tannin. Examples: o Terpenes (essential oils, saponin, rubber) o Phenolics (lignin, tannin, iso- flavonoid) o Nitrogen-containing compounds (alkaloid, cyanogenic glycoside) Serve as attractants for pollinators and fruit- dispersing animals Examples: o Terpenes (carotenoid) o Phenolics (anthocyanin, flavone) o Nitrogen-containing Nitrogen-containing compounds compounds (betacyanin) From amino acids Agents of plant-plant competition Examples: alkaloids, cyanogenic glycosides, non-protein amino acids Examples: (canavanine) o Simple phenolics V. Why Are Plants Not Poisoned by Their Own Toxic Secondary Metabolites? Stored as inactive precursors separate from their activating enzymes. Have modifications rendering them insensitive to the toxic effects. Stored away from sensitive metabolic processes.