BMS 200 - Physiology of Hearing, Taste, and Olfaction PDF
Document Details
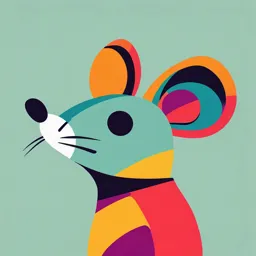
Uploaded by HandierMesa
CCNM
Tags
Summary
These are lecture notes covering the physiology of hearing, taste and olfaction. It includes descriptions of the anatomy and function of the ear structures, including the external, middle, and inner ear.
Full Transcript
BMS 200 – Physiology of hearing, taste, and olfaction Outcomes for today Describe the physiology of taste receptors and taste perception Describe the physiology of olfaction Describe the typical anatomy and physiology of the middle and external ears, including the structures responsible for the tra...
BMS 200 – Physiology of hearing, taste, and olfaction Outcomes for today Describe the physiology of taste receptors and taste perception Describe the physiology of olfaction Describe the typical anatomy and physiology of the middle and external ears, including the structures responsible for the transmission and modulation of auditory stimuli Describe the relationship between extracellular matrix (ECM) components and other middle ear structures, such as the ossicles and tympanic membrane, in facilitating sound conduction Describe the anatomical structures of the organs of hearing and equilibrium, including the external, middle, and inner ear, as well as the vestibular system. Outcomes for today Describe the functions of each component of the auditory system, including the role of the pinna, external auditory canal, tympanic membrane, ossicles, cochlea, and vestibular apparatus. Discuss the physiological processes involved in sound transmission, from the capture of sound waves by the external ear to the transduction and amplification of auditory signals in the inner ear. Describe the intricate mechanisms of the cochlea, including the organization of the hair cells, the role of the basilar membrane, and the function of the cochlear duct in sound perception. Relate the structure of the semicircular canals and otolith organs to the function of detecting rotational and linear acceleration, respectively. The ear – general structure Outer ear - structures Auricle (pinna) – the “floppy” part of your ear, composed of the helix, lobule, and tragus Functions: Focuses sound waves onto the tympanic membrane Certain structures tend to emphasize certain frequencies of sound Structures of the auricle change the nature of the sounds coming from different directions Outer ear - structures Auditory meatus – the opening of the external auditory canal The external auditory canal contains ceruminous glands (modified apocrine sudoriferous glands) Traps foreign substances Protects the delicate skin lining the external canal Cerumen (earwax) is composed of: anti-microbial proteins saturated fatty acids sloughed keratinocytes Mostly found near the entrance of the ear, most of the gland is located under the keratinzed stratified squamous epithelium Fine hairs are located near the meatus, along Middle ear - structures The tympanic membrane is the border between the external/outer ear and the middle ear fibroelastic connective tissue covered externally with epidermis and internally by simple cuboidal epithelium Middle ear - structures The tympanic cavity is found within the petrous part of the temporal bone lined by simple cuboidal epithelium that forms the “top layer” of a thin mucosa Pseudostratified columnar epithelium lines the auditory tube (Eustachian tube) and projects inferiorly and anteriorly, opening into the nasopharynx Usually collapsed – when you swallow or yawn, it opens up and equalizes the pressure between the atmosphere and the middle ear Middle ear - structures Ossicles (little bones) Malleus (latin for hammer) attaches to the tympanic membrane and incus (synovial joint) Tensor tympani muscle “dampens” the movements that are transmitted from the tympanic membrane to the malleus innervated by CN V Incus (latin for anvil) attaches to the malleus and the stapes (synovial joints) Stapes (latin for stirrup) Attaches to the incus and to the oval window Oval window: transition point between the middle and inner ear The stapedius muscle “dampens” the vibrations transmitted from the stapes to the oval window Innervation: CN VII Key middle ear structures If you were building an ear at Home Depot… FIGURE 4.17 Model of the middle ear. Vibrations from the eardrum are transmitted by the lever system formed by the ossicular chain to the oval window of the scala vestibuli. The combination of the four suspensory ligaments produces a virtual pivot point (marked by a cross); its position varies with the frequency and intensity of the sound. The stapedius and tensor tympani muscles modify the lever function of the ossicular chain. Middle ear - structures What is the point of all these little bones? They’re all levers Amplify the small movements of the tympanic membrane (TM) into larger movements at the oval window. The TM has 20X the surface area of the oval window This lever system, combined with the surface area difference, helps overcome the acoustic impedance mismatch between air and water. Air is “easier” to move than water without this system in place, sound waves in air would simply bounce off the oval window, failing to transmit vibrations to the cochlea. Vibrations in the fluid of the cochlea are sensed Inner ear – structures for sound Cochlea – the snail-like structure Coiled 2.5 times, ~ 35 mm long, about the size of a large pea It has 1,000,000 moving parts when you count all of the stereovilli (often called stereocilia) most complicated mechanical thing in your body Inner Ear – Lovely Diagram Inner ear – structures for sound Cochlea – the snail thingy Scala vestibuli: Connects to the oval window, filled with perilymph Separated from the scala media by Reissner’s membrane Scala tympani: Connects to the round window, filled with perilymph Separated from the scala media by the basilar membrane Continuous with the scala vestibuli (communicate Inner ear – structures for sound Cochlea – cont… Scala media Houses inner and outer hair cells Contains endolymph – much different ionic composition than the perilymph The basilar membrane houses the inner and outer hair cells The inner and outer hair cells contact the tectorial membrane (see diagram) Reissner’s membrane simply serves as a barrier so that endolymph doesn’t mix with perilymph Inner ear – structures for sound Cochlea – cont… Scala media – the organ of Corti The site of transduction for vibration action potential Organ of Corti stretches along the basilar membrane, 4 rows of hair cells: Inner hair cells – one row, about 3500 cells Project freely into the endolymph Outer hair cells – three rows, about 16,000 cells Project into the Inner ear – structures for sound Cochlea – cont… Endolymph has a very high K+ concentration (around 80 mmol/L, very strange composition) Perilymph is very similar to CSF Low protein, mostly sodium chloride solution Why is there so much K+ in endolymph? Stria vascularis is a highly vascularized tissue found in the peripheral part of the scala media Secretes K+ into the scala media, creating a massive K+ gradient between the endolymph and perilymph FYI: Composed of fibrocytes (perilymph side), intermediate cells, and marginal cells (endolymph The process of hearing – step-by- step Sound wave enters external auditory canal 1. Stapes moves inwards oval window moves inwards drop in pressure of the scala vestibuli 2. Round window moves outward (fluid is not very compressible) and the scala tympani pressure is now higher than the scala vestibuli pressure The process of hearing – step-by-step 3. Basilar membrane bends upward and the organ of Corti (the whole thing) shears towards the tectorial membrane 4. The hair bundles of the outer hair cells tilt toward the longer stereovilli 5. Transduction channels open in the outer hair cells K+ floods in depolarization of the outer hair cell receptor potential The process of hearing – step-by-step 6. Depolarization contraction of prestin contraction of the outer hair cell Very fast, happens within 100 microseconds 7. The basilar membrane moves upwards even more due to the contraction of the outer hair cells 8. Endolymph moves beneath the tectorial membrane The process of hearing – step-by-step 9. Inner hair cells bend towards their longer stereovilli 10. Transduction channels open in the inner hair cells depolarization (just like the outer hair cells) K+ floods in depolarization of the outer hair cell receptor potential The process of hearing – step-by-step 11. VG calcium channels open release of glutamate depolarization of the afferent neuron Afferent cell bodies are within the spiral ganglion Bipolar neurons – dendrites make contact with the inner hair cells – about 50,000 neurons The process of hearing - details Differences in frequency are distinguished by where the basilar membrane vibrates the most: High-frequency sounds are detected closer to the oval window Low-frequency sounds are detected closer to the helicotrema Younger people can hear a range between 20 – 20,000 Hz Pitch is detected based on what part of the organ of Corti detects the sound Differences in loudness are distinguished by how much the basilar membrane vibrates More movement more displacement of stereovilli greater fluctuations in the release of glutamate Therefore, loudness is encoded by frequency of What is sound, anyway? Sound wave formation – compression waves (A) Sound waves generated from a tuning fork cause molecules ahead of the advancing arm to be compressed and the molecules behind the arm to be rarified (B) Sound waves are propagated as sinusoidal, alternating regions of compression and rarefaction of air molecules. The wavelength of a sinusoidal wave is the spatial period between two peak compression waves. Sound action potential (transduction) The movements of the oval window (and round window) result in deflections of the basilar membrane and movement of hair cells Where the basilar membrane resonates the most depends on the frequency of the sound See bottom diagram Amplitude is detected based on the size of the standing wave vibration The Home Depot ear and hearing See notes below for discussion Equilibrium and the vestibular apparatus Two types of equilibrium are detected by the ear Static Detects position of head with respect to pull of gravity when body is not moving Is head tilted up? Down? To the side? Detects linear acceleration/deceleration An elevator speeding up or slowing down Car speeding up or slowing down Dynamic Detects angular movements of the head Is head being turned to the side? To the front? Is it being tilted? The vestibular system Each ear contains: three semicircular canals two otolithic organs, the utricle and the saccule Basic sensing elements of the vestibular system are neurosensory hair cells Semicircular canals are arranged within the temporal bone in planes that are at approximately right angles to each other horizontal (lateral), anterior (superior), and the posterior (inferior) semicircular canals. The three pairs of canals work in a push–pull fashion —when one canal is stimulated, its corresponding partner on the other side is inhibited and vice versa allows the vestibular apparatus to sense unequivocally all directions of rotation the right horizontal canal gets stimulated during head rotations to the right, and the left horizontal canal gets stimulated by head Vestibular apparatus FIGURE 4.22 The vestibular apparatus in the bony labyrinth of the inner ear (A) Activation of hair cells in the semicircular canals (B) The semicircular canals sense rotary acceleration and motion, whereas the utricle and saccule sense linear acceleration and static position Semicircular canal function Each canal has a dilated portion called the ampulla (near the utricle) Each ampulla contains the crista ampullaris ridge of tissue that is lined on its apical surface by vestibular hair cells, similar to those in the auditory system, and supporting cells Each vestibular hair cell contains up to a 100 stereocilia and a single longer kinocilium tips of the stereocilia and kinocilium are embedded in the undersurface of a dome-shaped gelatinous covering called the cupula. Rotation of the head in a particular direction causes movement of the endolymph fluid in the canals in the opposite direction (due to inertia deflects the cupula, which in turn bends the hair cell stereocilia. Semicircular canal function Three canals at right angles to each other Anterior canal: Vertical in frontal plane Detects side tilts Posterior canal: Vertical in sagittal plane Detects “Yes” nod Lateral canal: Horizontal Detects “No” nod Utricle and the Saccule Otolithic organs sense: static equilibrium (i.e., the resting position of the head while sitting, standing, or lying down) linear accelerations and decelerations The utricle and the saccule are saclike structures located in the vestibule, the bony chamber located between the semicircular canals and the cochlea. Saccule is oriented more vertically, utricle is oriented more horizontally Utricle and the Saccule Macula – plaque-like mound of specialized neurosensory tissue similar to the crista ampullaris Consists of three basic components: neurosensory hair cells, supporting cells, and a gelatinous covering known as the otolithic membrane surface of the otolithic membrane is embedded with calcium carbonate crystals, called otoliths or otoconia, which make the During membranelineardenser than the acceleration or endolymph deceleration, (like in a car or elevator) the otolithic membranes slide because of inertial lag, which is accentuated by the weight of the otolith This sliding over the surface of the maculae deflects hair cell stereocilia producing sensory signal In addition to detecting linear acceleration or deceleration, the Smell and Taste Chemoreceptors dedicated to smell and taste are activated by chemical molecules found in mucus within the nose (odorants) and saliva in the mouth (tastants) Taste – approximately 5000 taste buds Found in papilla along the dorsum and sides of the tongue Types of papillae: Fungiform papillae - near the tongue's tip Circumvallate papillae, forming a V-shape on the back of the tongue foliate papillae, located on the posterior edge Taste buds Fungiform papillae typically harbor up to five taste buds, primarily at their apex. In contrast, each circumvallate and foliate papilla can contain up to 100 taste buds, mainly along the papillae's sides filiform papillae – lack taste buds contribute to the tongue's rough texture and aid in the detection of food textures. Taste buds Beyond the tongue, taste buds are also present in the soft palate, epiglottis, and pharynx. Taste buds: 50–100 taste receptor cells – modified epithelial cells with microvilli at the apex, studded with receptors for tastants Microvilli project through the taste pore basal cells (stem cells) and support cells. Taste Saliva in the oral cavity serves as a solvent for tastants, facilitating their dissolution. This dissolved chemical then diffuses to the taste receptor sites. Additional function for saliva: bicarbonate secretion, aiding in swallowing, limited chemical digestion Each taste bud is innervated by approximately 50 nerve fibers, while each nerve fiber receives input from an average of five taste buds. Basal cells, originating from the epithelial cells surrounding the taste bud, differentiate into new taste cells, as taste cells have a lifespan of about 10 days Human taste perception is characterized by five fundamental modalities: salt, sweet, sour, bitter, and umami The central nervous system distinguishes between tastes because each taste receptor cell connects to a specific gustatory axon. Taste perception Salt sensitivity involves the activation of epithelial sodium channels (ENaC), leading to membrane depolarization through Na+ entry. Sour taste results from proton (H+) stimulation, facilitated by ENaCs that permit proton entry. Additionally, H+ ions may block K+- sensitive channels, causing membrane depolarization Sour transduction may involve hyperpolarization-activated cyclic nucleotide-gated cation channels (HCN) and other mechanisms. Taste perception Sweet taste perception involves at least two G protein- coupled receptors (GPCRs), T1R2 and T1R3. Both natural sugars and structurally different compounds like saccharin activate sweet receptors Bitter taste is evoked by a range of unrelated compounds, often serving as a warning against poisons. Some bitter compounds, such as quinine, block K+-selective channels, while others, like strychnine, bind to GPCRs (T2R family), Umami tastants activate a receptor composed of T1R1 and T1R3. The umami taste may also involve the activation of a truncated metabotropic glutamate receptor, mGluR4, within taste buds. BMS 200 – Physiology of hearing, taste, and olfaction P2 Structure of the Olfactory Epithelium Specialized segment of the nasal mucosa that houses olfactory sensory neurons approximately 10 cm² upper part of the nasal cavity, near the septum in humans. Major cell types of the olfactory epithelium: olfactory sensory neurons – bipolar neuron responsible for signal transduction of odorant receptor potential These neurons feature a short, thick dendrite that extends into the nasal cavity, terminating in a knob housing 6–12 cilia. These cilia protrude into a thin layer of mucus Structure of the Olfactory Epithelium The axons of olfactory sensory neurons, forming the olfactory nerve, traverse the cribriform plate of the ethmoid bone to reach the olfactory bulbs. Supporting cells within the olfactory epithelium are responsible for secreting mucus, creating an optimal molecular and ionic environment for detecting odors. Odor-producing molecules, or odorants, dissolve in the mucus and bind to odorant receptors on the cilia of olfactory sensory neurons. Structure of the Olfactory Epithelium Odorant-binding proteins present in the mucus may enhance the diffusion of odorants to and from the odorant receptors. Basal stem cells replace olfactory neurons Olfactory sensory neurons typically have a lifespan of only 1–2 months. Odorant Receptors and Signal Transduction Odorant receptors considerable diversity in their amino acid sequences, but all are G- protein-coupled receptors (GPCRs). Typical Gs proteins for the most part What is the signal transduction cascade? Usually results in the opening of Cl- and Ca+2 channels Olfactory Sensory Pathway Overview Within the olfactory bulb, olfactory sensory neurons' axons synapse on the primary dendrites of mitral cells and tufted cells, forming distinctive olfactory glomeruli. Each olfactory sensory neuron expresses a single one of the 400 functional olfactory genes, while individual odorants can bind to a diverse array of odorant receptors. Olfactory Sensory Pathway Overview A unique two-dimensional map in the olfactory bulb is created, as each olfactory sensory neuron projects to only one or two glomeruli, providing specificity to the associated odorant. Mitral cells, along with their glomeruli, project to various regions of the olfactory cortex. The central olfactory system decodes the receptor-cell activity pattern, revealing the identity of the odorant. Otosclerosis Definition: Abnormal bone deposition in the middle ear – Occurs around the rim of the oval window where the footplate of the stapes fits (obviously will affect hearing) – Both ears are usually affected Pathogenesis – Familial (autosomal dominant), but cause of the bony overgrowth, genes involved, are unknown… which is hard to believe because it’s so common May be related to measles infection – Begins with fibrous ankylosis of the footplate bony overgrowth anchoring it into the oval window Seems to be caused by an imbalance between bone deposition and resorption Otosclerosis Clinical features Progressive hearing loss due to immobilization of the oval window is the main symptom Epidemiology Usually begins in the early decades of life; minimal degrees of this derangement are very common (10%!!) More severe symptomatic otosclerosis is relatively uncommon Even those without severe disease can progress to significant hearing loss over decades