BME 350 - Quiz 1 Study Notes PDF
Document Details
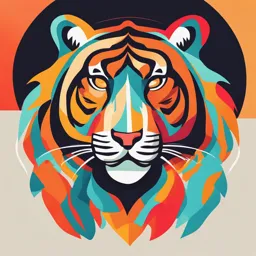
Uploaded by HelpfulWaterfall3031
University of Kentucky
Tags
Summary
The document is a set of study notes for a BME 350 quiz, centered on the importance of materials. The notes cover material properties, the science of materials, and the engineering of materials. Additionally, it looks into atomic structure.
Full Transcript
Chapter 1: The Importance of Materials Used to optimize function as needed Material: Something tangible that goes into the makeup of a Ductility – common property physical object Corrosion resistance Material Sci...
Chapter 1: The Importance of Materials Used to optimize function as needed Material: Something tangible that goes into the makeup of a Ductility – common property physical object Corrosion resistance Material Science: Investigating the relationships between the Example: Goliath structures and properties of materials ITS ALSO ABOUT THE MONEY! Materials Engineering Iron weapons are cost-effective “Scientists do to learn; engineers learn to do” Carbon steel is processed by smelting Goals: Metals and Society o Adapt/modify to meet societal needs Metals: o Create new combos of elements leading to o Ductile materials with desirable properties o Economical NO new materials o Conductor o “new” = different combos of existing o Engineerable materials § Composition At the heart of every engineering problem is a § Processing materials problem Structural steel Human Activates & Advancement o Bridges, buildings, transportation, consumer Humans make things to aid Activities of Daily Living goods, defense (ADL) Metals: Christian Jurgensen Thomsen Useful in engineering industry o 3 categories of quantum materials Beneficial for surgical use technology (Stone, Bronze, Iron Age) Ceramics Ages are defined by events not time! Multiple combos of metals and non-metals Stone Age Not limited to 1 metal + 1 non-metal Longest Age Benefits: Materials: o Chemical stability o Stone, clay, wood, hair, fur, animal skins, o High melting point bone, sinews (tendons/ligaments) o Aesthetics o Not just for beauty, but also for function o Hardness Bronze Age o Non-conducting Bronze: an alloy (2 or more elements) Disadvantages: o Competitive advantage – superior properties o Brittle vs other available materials o Non-conducting o SUPERIOR engineering properties o Acoustics (squeaky) Shaped by hammering or casting Remedy: o Much easier than shaping stone o Add alloying elements or change processing Control hardness by chemical composition (alloying) o Gain toughness and enhance usefulness Corrosion resistant § Toughness gained for hardness lost Thomas Jefferson o Transparent ceramics o “Those who hammer their swords into plows Material Composition and Processing will plow for those who do not” FAST: Field Assisted Sintering Technique Used extensively today for: Material Structure o Bushings and bearing material Metals and ceramics are highly ordered on atomic o Marine hardware scale o Coins and medals Atoms arranged in regular patters = crystalline o Sculpture Glass = non-crystalline structure o Household hardware Impurities in Materials Iron Age Impurities are not necessarily bad Harder and tougher than bronze Impurities in Ceramics o Key materials engineering metric Naturally occurring Hardness How gemstones come to be (examples are ruby, o Greater cutting efficacy sapphire, diamond) o Last longer Polymers o Standard: Rockwell Hardness Testing “plastics” § Trade-offs on knife material Lightweight, ductile, low-cost alternative to metals hardness Lower strength and melting point vs metals Toughness: Greater chemical reactivity o Energy to resist fracture Example: Kevlar Where bronze excelled, it remained Nylon Where iron excelled, it replaced o Thermoplastic polymer Mixed Materials Use Silk To get desired properties and serve a specific role Polyethylene BONE = oldest known composite o Structure of mers is key to properties of this o Biological component: organized collagen polymer o Inorganic component: imperfect mineral o Multiple forms Examples: Molecular weight is most important of structure o plywood: layers of alternating woods determining polymer properties o fiberglass: fibers in glass matrix Materials Testing o auto glass: polymer between tempered glass Empirical discipline layers Composites o reinforced concrete: steel rebar + concrete Combo of 2 or more materials § concrete = cement + aggregate Each have different physical and chemical properties stone Materials Age Prior societies (Stone, bronze, iron) Contemporary society (polymers, silicon, composite Revision arthroplasty never preforms well as the materials, super alloys, nanomaterials) primary arthroplasty (worse patient outcomes) Material strength to density ratio Components showed ® worn, malformed acetabular A Materials Engineering Mindset cups, delaminated pitted 1. Think: Present Materials Advances Composition Smart materials ® shape memory alloys or self- Structure healing materials Processing Nanomaterials ® targeted drug delivery 2. Which Provide SUMMARY Desired engineering properties 1. At the core of every engineering problem is a 3. Enable practical application materials problem Do the above on multiple dimensional scales 2. Materials structure is determined by composition and Dimensional Scales processing Subatomic level 3. Material composition and processing determines Atomic level properties Microscopic level 4. Material properties determine function and Macroscopic level engineering application Analyze on each scale size 5. Materials science and engineering occurs at all scale Do for each material challenge you encounter levels 6. Desing always involves material selection Relevant structure, properties, processing and 7. Biomedical technology is critically dependent on performance material performance Wear of Total Joint Polyethylene (MAJOR material 8. Materials interact with biological systems (vice problem!) versa) Small wear particle disease 9. Ignore any of the above at risk of peril-case studies in o Particles causing “aseptic” loosening of point implants Consequences ® pain, disability, surgery and cost Chapter 2: Atomic Bonding 2. Identify available resources and work backwards Testing = BANNED (reverse engineering) Open air 3. “Primum non nocere” (first, do no harm) Underground Fundamentals Nuclear deterrence remains NEVER change Computer modeling of nuclear reactions is only Always applicable permitted Provide guidance to problem solution Bonding Biomaterials Engineering At the heart of material engineering properties Biological design ® cell fundamentals Absent an understanding of the scientific basis of Materials design ® material fundamentals bonding, description of macro level material Primary Bonding properties is merely phenomenological and not based Major source of cohesion in engineering materials on sound engineering Transfer or sharing of electrons Design Strategies Secondary Bonding 1. Identify endpoints and start from fundamentals No sharing or transfer of electrons (weaker attraction) Bonding is a major determinant of engineering material Occurs by events happening to the outer orbital type and the properties of the materials (valence) electrons when one atom comes into the Chemical Bonding vicinity of another atom Heisenberg’s Uncertainty Principle Result from mixing of 1 s orbital with 3 p orbitals to (PUT EQUATION) yield 4 sp3 hybrid orbitals Electrons Carbon 12 Both particles and waves (dual nature) C chemistry is the basis of the chemistry of life! Particles Atomic Bonding o Dot-bonding = ok It’s all about how the outer shell electrons interact o Position is deterministic between atoms Waves Ionic Bond o Dot-bonding = not ok Electron transfer from one atom to another o Position is probabilistic “Both parties in the contract benefit from the deal” Orbitals are obtained by the Schrodinger equation Results from the electrostatic attraction between ions o Many solutions possible with opposite charges o Each gives a different electron probability Ionic bonds are non-directional distribution around the atom o Have isotropic mechanical properties o Each has a unique shape Unstrained material ® opposite charges are adjacent o Quantum numbers are identified ® material maintains integrity Nuclear Details Strained material ® like charges adjacent ® material Mass of proton/neutron = 1.66 x 10-24 grams integrity compromised Mass of 1 = 1 Atomic Mass Unit (AMU) Coulomb’s Law of Electrostatics = basis of the ionic Reciprocal of AMU = Avogadro’s number bond Periodic Table Example: NaCl Each row = same number of electron shells NaCl “Arrangement” Each column = same number out orbital electrons Atomic level: Na meets Cl Atomic weight = number of protons + number of Supra-atomic level: Na surrounds Cl neutrons Stacking arrangement Atomic number = number of protons in element Coordination number: the number of adjacent ions (or Chemical identification occurs relative to nucleus atoms) surrounding a reference ion (or atom) Chemical bonding occurs relative to electrons and Force of Repulsion electron orbitals Arises from negative charged inner shells or positive Electronegativity charged nuclei getting too close (like charges repel) Ability of an atom to attract electrons to itself (how Bond Energy thirsty) Energy = the ability to do work Lower left of period table = least thirsty, upper right = o Force x distance greatest thirst (except noble gases) Minimum bond energy occurs when Fnet = 0 and a = a0 Larger the difference between 2 elements, the more Macro Tension / Compression of Materials tightly held the electrons are to the more Compress a material ® pushing ions closer than a0 electronegative element Pull on a material (tension) ® pulling ions father apart Isotopes than a0 Equal number of protons but different number of Coordination Number neutrons Relative size = radius ratio = r/R Number of protons of all isotopes of a chemical Number for covalent bonding do not follow the rules species are equal as closely as occurs for ionic bonding Number of electrons of all isotopes of a chemical Covalent Bond species are equal Cooperative sharing of valence electrons between two Electron Orbitals adjacent atoms Electrons of all atoms are in orbitals about the nucleus Highly directional Each orbital is associated with a different energy level Example: C-C crystal structure of electrons in that orbital 4 representations Orbitals are not “pure” o Planetary model “Filling of electron shells” o Actual electron density Lewis dot models o Electron dot schematic Orbital diagrams o Bond-line representation Hybrid Orbitals Bond Angle Group sharing of outer orbital electrons Determined by the directional nature of valence “sea of electrons” electron sharing Cations are attracted to the cloud of electrons Apply to covalent bonds NOT ionic bonds o Basis of material integrity Semiconductors Valence electrons are not transferred or shared Material integrity governed by covalent bonding o They are delocalized and freely able to move Metallic Bonds Non-directional Atomic level structure determines macro level Charge * separation distance properties Secondary Bonds II Coordination numbers are typically high and Hydrogen bonds determined by efficient packing considerations o Due to permanent distortions caused by Metal properties electronegativity of an atom o Luster o Attractive force between H atom to a highly o Heat conductive electronegative atom (N,O,F) o Ductile o Can occur in organic molecules o Malleable o Stronger than van der Waals o Strong o Weaker than ionic/covalent/metallic bonds o Electrically conductive Water Example: titanium alloy Solid water expands uniformly Involves different ionization energy levels for electron Liquid water packs together more randomly and atoms in the outer orbitals of metal atoms densley o Outer shell energy (metallic) < outer shell Secondary bond energy (covalent) Polyethylene o Outer orbitals are S-shaped that may overlap # of mers determines molecular weight with up to 12 additional S-orbitals Molecular weight determines properties o “Well defined” electron sharing does not Covalent bonds give strength within the polymer occur Secondary bonds give weak links between un- Outer shell valence electrons are NOT associated with crosslinked chains any single positive meal ion Material Melting Points o Bonds can form by the exchange of valence Gives info regarding bond strength electrons without asymmetrical dispersal Shows relative values of thermal energy needed to Quantum Mechanics of Metallic Bonding disrupt various types of bonds Lower energy state of outer shell “delocalized” The strength of induced dipoles vs permanent dipoles electrons is responsible for the boding that occurs as reflected in melting temp If outer shell electrons become “localized”: Chemical Bonding o Bonding would assume a covalent nature NOT always ideally separated o Be directional o Pure bonds vs mixed bonding o Have lower electron mobility Within a single bond ® mixed boding may occur Dipole Among bonds between molecules Created when a pair of electrical charges are separated Composite Materials Permanent Combos of ionic, covalent, metallic, and secondary o 2 atoms with large differences in bonding each introduces by the constituent added electronegativity AND the interactions between the constituents o 1 atom is (-) and the other is (+) SUMMARY o Polar molecule Primary Bonding Instantaneous o Ionic bonds: o Redistribution of continuously moving § Electrons transferred between atoms electrons § Creates opposing charged ionic § More electrons in one region than species another § Non-directional electrostatic Induced attraction is the basis of material o Molecule with a permanent dipole repels integrity and the basis of simple another molecules electron ® induces a geometric packing efficient dipole moment in the 2nd molecule determining coordination number Secondary Bonds I § Equilibrium ion spacing balances Van Der Waals Bonds between attractive forces of o Attraction of oppositely charged atoms oppositely charged ions with without electron transfer repulsive forces arising from atomic o BASIS: atom A causes distortion of the cores “probability” cloud of the electron cloud o Covalent bonds: distribution of atom B § Specific outer shell electrons are § Instantaneous net region of charge shared between atoms in one location of atom B vs. another § Packing between atoms is ® inducing a dipole compromised by directional nature o Example: Argon of bonds o WEAK § Result in more “open” material o Low melting points structures Dipole Moment § Highly directional mechanical properties o Metallic bonds: § Outer shell de-localized electrons are shared among all atoms § Lower energy state is basis for attraction among metal ions. § 3D electron sharing ® non- directional bonding High coordination number Chemical bond basis of material isotropy § High electrical and thermal conductivity Secondary Bonding o Fundamentally electrostatic in nature Bonding & Materials o Van der Waals bonds o Metals § Temporary dipoles created by § Metal atoms statistical fluctuations in location of § Material integrity via metallic orbital electrons bonding § Liquids and solids ONLY o Ceramics o Hydrogen bonds § Metals + nonmetals § Permanent dipoles created by § Material integrity via ionic bonding structure of individual molecules via Coulombic forces o MUCH weaker bonds o Polymers § Quantified by lower melting points § Few atoms showing in periodic Chemical Bonds table o All about the electrons and how they are § Material integrity via covalent and shared or transferred between adjacent atoms van der Waals bonding