Physiology for Dental Students (DENT 625) Fall 2024 PDF
Document Details
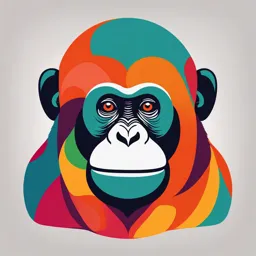
Uploaded by ReasonableRockCrystal9853
University of Maryland, College Park
2024
Tom Adair, Jennifer Duckworth
Tags
Summary
This document is a course outline and notes from a physiology course for dental students. It covers fundamental concepts like transport of substances through cell membranes and ion channels. It discusses basic physiology and integrates topics in biochemistry, bioinformatics, and clinical medicine.
Full Transcript
Fall 2024 Physiology for Dental Students (DENT 625) Fundamentals of Physiology (PHYSIOL 725) What is Physiology? Physiology is concerned with normal function of living organisms and their parts, and of the physical and chemical processes involved in maintaining life. … how the body...
Fall 2024 Physiology for Dental Students (DENT 625) Fundamentals of Physiology (PHYSIOL 725) What is Physiology? Physiology is concerned with normal function of living organisms and their parts, and of the physical and chemical processes involved in maintaining life. … how the body works normally … Human Physiology Physiology links basic sciences and medicine Clinical Medicine Genes Gene Expression Cell Function Biochemistry Proteomics Bioinformatics Integrative Physiology Physiology 2024 Tom Adair, PhD [email protected] Jennifer Duckworth G251 [email protected] Course Information Course Information (cont.) Course Information (cont.) Course Information (cont.) Quizzes will usually be given before the material is presented in class Course Information (cont.) Course Information (cont.) Course Information (cont.) Course Information (cont.) Course Information (cont.) Physiology Course Schedule Physiology Course Schedule Physiology Course Schedule Course Coordinator Jennifer Duckworth, [email protected] 601-984-1810 Instructors Thomas H. Adair, PhD Course Director [email protected] Block 1: Nerve-Muscle-Heart Block 2: GI Physiology Block 3: CNS, Special Senses Instructors (cont.) Barbara Alexander, PhD Professor of Physiology and Biophysics [email protected] Block 4, Part A: Circulatory Physiology Instructors (cont.) Joshua S. Speed, PhD Assistant Professor of Physiology and Biophysics Block 4, Part B: Circulatory Physiology Instructors (cont.) Heather Drummond, PhD Professor of Physiology and Biophysics [email protected] Block 5: Pulmonary Physiology Instructors (cont.) David Stec, PhD Professor of Physiology and Biophysics [email protected] Block 6: Renal Physiology Instructors (cont.) Eric George, PhD Associate Professor of Physiology and Biophysics [email protected] Block 7: Endocrine/Reproductive Physiology UNIT 2 Chapter 4: Transport of Substances Through cell Membranes Slides by Thomas H. Adair, PhD Copyright © 2021 by Saunders, an imprint of Elsevier Inc. Fluid mosaic model proteins phospholipids hydrophilic hydrophobic hydrophilic Van der Waals interactions Cholesterol increases fluidity Singer SJ, Nicolson GL. The fluid mosaic model of the structure of cell membranes. Science 18;175(4023): 720-31, 1972. Lipid Bilayer: The lipid bilayer is a barrier to water and water-soluble substances CO2 O2 H2O ions glucose N2 halothane urea Cell Membrane … but, other molecules can still move through the membrane! urea CO2 ions H2O O2 N2 glucose isoflurane Permeability coefficients (cm/sec) (Permeability coefficients expressed as cm/sec are shown for an artificial lipid bilayer without protein channels) 10-2 high permeability water 10-4 urea glycerol 10-6 glucose 10-8 10-10 Cl- K+ 10-12 low permeability Na+ Molecular Gradients across cell membrane Figure 4-1 Fig. 4.1 Transport across the cell membrane Simple diffusion Active transport Molecules move down a Molecules move against a concentration concentration gradient gradient (or electrochemical gradient) No additional energy is required Involves a protein “carrier” Requires ENERGY (ATP) Movement of molecules through protein channels is now considered to be facilitated diffusion, not simple diffusion. (See The Cell: A molecular approach). TMP15 Figure 4-2 Fig. 4.2 Simple Diffusion and Facilitated Diffusion Simple diffusion: Lipid-soluble Facilitated diffusion: Water-soluble molecules move readily across cell molecules cross the cell membrane membranes. The rate of diffusion by way of protein channels and other depends on lipid solubility. transport proteins. Simple Facilitated Diffusion Diffusion Diffusion of a molecule in water Figure 4-3. Diffusion of a single molecule of water during one-thousandth of a second (1 msec). Copyright © 2011 by Saunder s, an imprint of Elsevier Inc. Facilitated diffusion of water through cell membranes Water molecules cross cell membranes by facilitated diffusion through water- selective protein channels called aquaporins. The rapidity of water diffusion through most cell membranes is astounding, e.g., water diffusion through the RBC membrane per second is 100x the volume of the RBC. There are at least 13 different types of aquaporins in humans. Aquaglyceroporins are a subset of aquaporins that allow the passage of both water and glycerol. Aquaglyceroporins are thought to play an essential role in the hydration of mammalian skin epidermis as well as the proliferation and differentiation of keratinocytes. Recall that keratinocytes are the main epidermal cell type in the skin and play an essential role in the skin's defense against infection. Copyright © 2011 by Saunder s, an imprint of Elsevier Inc. Ion Channels There are two main types of ion channels: A. Ungated channels Transport is determined by the size, shape, and charge of the channel and the ion. B. Gated channels (Two types) Voltage-gated (e.g., voltage-gated Na+ channels) Chemical or ligand gated (e.g., nicotinic acetylcholine receptor channels) Ungated Voltage-gated Ligand-gated Intracellular Extracellular Na+ and other ions Na+ Copyright © 2011 by Saunder s, an imprint of Elsevier Inc. Selectivity of potassium channel Dehydrated Hydrated Sodium 0.95 Å 3.58 Å Potassium 1.33 Å 3.31 Å How can a membrane channel be permeability to potassium, but not to sodium when the potassium molecule is larger? Carbonyl oxygens in the selectivity channel essentially strip water molecules from the potassium molecule (but not the sodium molecule), so only potassium ions can permeate. Figure 4-4 Selectivity of sodium channel Sodium channels are lined with negatively charged amino acids (glutamate) that literally pull the sodium ion away from its water shell. The smaller, unhydrated sodium ion can then diffuse through the channel. Unhydrated potassium ions are too large. Dehydrated Hydrated Sodium 0.95 Å 3.58 Å Potassium 1.33 Å 3.31 Å Figure 4-5 How to Study Ion Channels? Patch Clamp Method Nobel Prize in Physiology or Medicine -1991 Extracellular Intracellular Patch clamp method Figure 4-6B Operation of Voltage-gated sodium channel Intracellular Extracellular 25 mV Na+ The channel conducts “all Fig. 4.5 or none,” open or closed Figure 4-6A Simple vs. Facilitated Diffusion simple diffusion rate of diffusion (Co-Ci) Rate of diffusion Vmax facilitated diffusion (aka, carrier-mediated diffusion) Concentration gradient (Co-Ci) What limits maximum rate of facilitated diffusion? What limits the maximum rate of Facilitated Diffusion (Vmax)? The maximum rate of facilitated diffusion for a given protein transporter is limited by the maximum rate of conformational change of the protein transporter. Theoretical mechanism to explain how a protein channel could have a Vmax. Fig. 4.7 Figure 4-8 What is Net Diffusion? A B Magnitude of diffusion from side A to B Magnitude of diffusion from side B to A Magnitude of net diffusion (green arrow – red arrow) Electrical potential (EMF) + − − − − − − − − − − − − − − − − −− − − − − − − − − − − + − − − − − − When will the negatively charged − − − − − − − molecules stop − − − − − − − − entering the cell? − − − − − The Nernst potential (equilibrium potential) is the theoretical intracellular electrical potential that would be equal in magnitude but opposite in direction to the concentration force. The Nernst equation follows: EMF (mV) = ±61 log (Ci / Co) Active Transport Primary Active Transport Molecules are “pumped” against an electrochemical gradient at the expense of energy (ATP) – direct use of energy Secondary Active Transport Transport is driven by the energy stored in the electrochemical gradient of another molecule (usually Na+) – indirect use of energy Primary Active Transport 1. Na+-K+ ATPase Located on the plasma membrane of all animal cells. Pumps sodium ions out of cells and pumps potassium ions into cells against electrochemical gradients. Plays a critical role in regulating osmotic balance by maintaining Na+ and K+ balance (inhibition of pump by ouabain causes the cell to swell and burst!) Pump is activated by an increase in cell volume Requires about 1/5 of a typical cell’s energy and up to 2/3 of a neuron’s energy. Primary Active Transport (cont.) subunit Fig. 4.11 100,000 MW binds ATP, 3 Na+, and 2 K+ subunit 55,000 MW function is not clear Figure 4-12 Transport is electrogenic (3Na+ / 2K+), but the pump's electrogenic nature contributes less than 10% to the membrane potential. A non-electrogenic pump (2Na+ / 2K+) would change membrane potential by less than 10%. Primary Active Transport (cont.) 2. Ca2+ ATPase Present in plasma membrane of ALL cells. Present in sarcoplasmic reticulum of all muscle fibers Maintains a low cytosolic Ca2+ concentration 3. H+ ATPase Found in parietal cells of gastric glands (HCl secretion) and intercalated cells of renal tubules (controls blood pH) Can concentrate H+ ions up to 1 million-fold Secondary Active Transport - Uses an electrochemical gradient (usually for sodium) to power transport of other molecules. - Protein cotransporters are classified as symporters or antiporters 1. Symporters transport a substance in Na+ the same direction as a “driver” ion like Na+. AA extracellular Na+ AA Na+ gluc Na+ 2 HCO3- Examples: intracellular Secondary Active Transport (cont.) 2. Antiporters: substance is transported in the opposite direction of a “driver” ion Na+ like Na+ Ca2+ Na+ Na+ Na+/HCO3- Examples: extracellular intracellular Ca2+ H+ Cl-/H+ Q: How do cardiac glycosides increase cardiac contractility? Glycosides (e.g., digoxin) inhibit Na+-K+ ATPase. This causes: 1. Increased intracellular Na+ concentration 2. Decreased Na + electrochemical gradient 3. Decreased activity of Na +/Ca2+ antiporters 4. Increased intracellular Ca2+ concentration 5. The increase in intracellular Ca 2+ causes increased contractility Digitalis purpurea (Common Foxglove) William Withering, 1775 “Dropsy” is an old term that means edema. Edema is often caused by congestive heart failure Q: How do cardiac glycosides increase cardiac contractility (cont)? Na+ Na + K+ Again, digoxin binds to and inhibits the Na + Na+/K+ pump within the plasma membrane of cardiomyocytes. This Ca++ inhibition increases the intracellular Na+ concentration, which in turn increases the intracellular Ca2+ concentration. The increase in Ca2+ concentration then leads to increased cardiac contractility. Osmosis Osmosis is the net movement of water across a semipermeable membrane. Water moves from an area of high concentration to an area of low concentration, as shown. Figure 4-10 Fig. 4.9 Osmotic Pressure Osmotic pressure is defined as the pressure that must be applied to stop fluid movement when a semipermeable membrane separates a solution from pure water. Osmotic pressure is attributed to the osmolarity of a solution Figure 4-11 Fig. 4.10 Speaking of osmolarity… Why Does Honey Lasts Forever? Honey doesn’t go bad In 2015, archaeologists because it has a high osmolarity and is acidic. reported finding 3,000- year-old honey while The high osmolarity causes water to move out of bacteria, excavating tombs in which essentially makes the bacterial cells implode. Egypt, and it was perfectly edible. Honey is acidic with a pH of about 3.9, which is too acidic for most organisms to survive. Honey also contains an enzyme called glucose oxidase. This enzyme converts some of the sugars into hydrogen peroxide, which kills bacteria and is also why honey is sometimes used for treating wounds. Behavior of Cells in Solution - isotonic, hypertonic, hypotonic - Osmolarity is high in vasa recta Plasma: 300 mOsm Renal medulla: 600-1200 mOsm Estimating plasma osmolarity Plasma is clinically accessible. Dominated by [Na+] and associated anions Under normal conditions, extracellular osmolarity can be roughly estimated as: POSM = 2 [Na+]p = 270-290 mOsm Chapter 5: Membrane Potentials and Action Potentials Slides by Thomas H. Adair, PhD Copyright © 2021 by Saunders, an imprint of Elsevier Inc. Active Transport of Na+ and K + Remember: sodium is pumped out of the cell, potassium is pumped in... inside outside K+ Na+ Na+ ATP K+ 3 Na+ 2 K+ ADP Simple Diffusion of Na+ and K + through leak channels inside outside K+ K+ Na+ Na+ Membrane Potential (Vm): - is a charge difference across the membrane - inside outside …how can the passive diffusion of potassium and sodium lead to the K+ K+ development of a negative membrane potential? Na+ Na+ Simplest Case Scenario for K+ inside outside If a membrane were permeable to only K+ then… K+ would diffuse down its concentration K+ K+ gradient until the electrical potential across the membrane countered diffusion. The electrical potential that counters the net diffusion of K+ is called the K+ equilibrium potential (EK). The Potassium Nernst Potential …also called the equilibrium potential Ki EK = -61 x log Ko Example: If Ko = 4 mM and Ki = 140 mM EK = -61 log(140/4) EK = -61 log(35) EK = -94 mV So, if the membrane were permeable only to K+, the membrane potential (Vm) would be -94 mV Simplest Case Scenario for Na+ If a membrane were permeable to only inside outside Na+ then… Na+ would diffuse down its concentration Na+ Na + gradient until potential across the membrane countered diffusion. The electrical potential that counters the net diffusion of Na+ is called the Na+ equilibrium potential (ENa). The Sodium Nernst Potential Nai ENa = -61 x log Nao Example: If Nao = 142 mM and Nai = 14 mM ENa = -61 log(14/142) ENa = -61 log(0.1) ENa = +61 mV So, if the membrane were permeable only to Na+, the membrane potential (Vm) would be +61 mV Resting Membrane Potential Vm -90 to -70 0 mV EK -94 ENa +61 Why is Vm so close to EK? Why is the cell membrane so permeable to K? Carbonyl oxygens Figure 5-4 100x more K+ leak channels compared to Na+ Channels The Goldman-Hodgkin-Katz Equation (also called the Goldman Field Equation) Calculates Vm when more than one ion is involved. + + − + + p' [ K ] p' Na [ Na ]o p'Cl [Cl ]i Vm = 61. log K + o + − p'K [ K ]i + p'Na [ Na ]i + p'Cl [Cl ]o or + + − + + p' [ K ] p' Na [ Na ]i p'Cl [Cl ]o Vm = -61. log K + i + − p'K [ K ]o + p' Na [ Na ]o + p'Cl [Cl ]i Vm = membrane potential P’ = permeability The Goldman-Hodgkin-Katz Equation Take home message… The resting membrane potential is closest to the equilibrium potential for the ion with the highest permeability! Understanding the Nernst Equation Nernst potential = equilibrium potential = diffusion potential = reversal potential Inside Nernst potential = Eion = +/- 61 x log Outside + for negative ion What is the Log of 1? What is the log of a number > 1 (+ or -)? – for positive ion What is the log of a number < 1 (+ or -)? Prove to yourself that for positively charged ions: When inside concentration > outside concentration, Eion is negative (e.g., K+) When inside concentration < outside concentration, Eion is positive (e.g., Na+) Answer the following questions. What is the equilibrium potential for any ion when inside concern = outside concentration? What is ECa++? Positive or negative? What is ECl-? Positive or negative? What happens to E K+ when plasma K+ concn increases? What happens to Vm? What happens to E K+ when plasma K+ concn decreases? What happens to Vm? Resting Vm for various cell types Cell type Resting Vm Skeletal muscle fibers -85 to -95 mV Smooth muscle fibers -50 to -60 mV Astrocytes -80 to -90 mV Neurons -60 to -70 mV Erythrocytes -8 to -12 mV Photoreceptor cells -40 mV (dark) to -70 mV (light) Electrochemical Driving Force (VDF) The electrochemical driving force (VDF ) for any ion is the difference in millivolts between the membrane potential (Vm) and the equilibrium potential for that ion (E ion). (VDF = Vm – Eion) Normal conditions EK -94 Vm -74 ENa+61 0 mV +20 mV -135 mV What is the electrochemical driving force on K + ions? What is the electrochemical driving force on Na + ions? What is the direction of Na+ and K+ ion diffusion at rest? How about during increased permeability? Note: Positive VDF -- ion exits cell (Efflux) Negative VDF -- ion enters cell (Influx) Effect of changing permeability of Na + and K + on membrane potential EK -94 Vm ENa+61 0 mV What effect does increasing K+ permeability have on Vm? What effect does increasing Na+ permeability have on Vm? Functions of action potentials Deliver sensory information to CNS – APs in sensory neurons are blocked by local anesthetics (LAs). This usually produces analgesia without paralysis. Why no paralysis? LAs are more effective against small diameter neurons with a large surface area to volume ratio. Hence, small C-fibers that conduct pain sensations are affected more than large, alpha-motor neurons. Pain fiber Motor neuron Information encoding – The frequency of APs encodes information (amplitude of AP is constant). Rapid transmission over distance (nerve cell APs) – The speed of transmission depends on fiber size and whether it is myelinated. Information of lesser importance is carried by slowly conducting unmyelinated fibers. Non-myelinated c-fibers conduct pain sensations. In non-nervous tissues, APs initiate various cellular responses – muscle contraction – secretion (e.g., Epinephrine from chromaffin cells of medulla) Resting and action potentials There are some terms that need to be understood & remembered: – Depolarization overshoot – Hyperpolarization 0 mV – Overshoot excitability repolarization means positive to 0 mV + – Repolarization threshold towards resting potential depolarization resting – Excitability -90 mV hyperpolarization potential – Threshold (for action potential generation) - What is the effect of high and low plasma potassium ion concentration on membrane potential and excitability? Measurement of action potential Figure 5-6 The action potential (AP) stimulate Recording An action potential: AP of AP – is a regenerating depolarization of membrane potential that propagates along an excitable membrane. axon = 60 mm or 6 cm Propagates: conducted without decrement (an AP Velocity ~ 60 m/s ‘active’ membrane event) = 60 mm in 1 ms Excitable: capable of generating action potentials upstroke +61 ENa Action potential basics: All-or-none event (need to reach threshold) Constant amplitude (do not summate) 0 Initiated by depolarization downstroke Involve changes in permeability (mV) Rely on voltage-gated ion channels -90 EK 1 ms Properties of action potentials +60 Action potentials: are all-or-none events 0 ❑ threshold voltage (usually 15 mV positive to mV threshold resting potential) -70 are initiated by depolarization ❑ action potentials can be induced in nerve and muscle by extrinsic (percutaneous) stimulation have constant amplitude ❑ APs do not summate - information is coded by time frequency not amplitude. 75 Myelinated have constant conduction velocity (cat) 50 ❑ True for given nerve fiber. Fibers with a large diameter conduct faster than small fibers. As a non-myelinated general rule: 25 (squid) ❑ myelinated fiber diameter (in mm) x 4.5 = 0 velocity in m/s. 0 3 6 9 12 15 ❑ Square root of unmyelinated fiber diameter 0 400 800 = velocity in m/s Fiber diameter (m) The AP - membrane permeability +61 ENa During upstroke of action potential: ❑ Na permeability increases due to opening of Na+ channels 0 memb. potential approaches ENa (mV) During downstroke of action potential: resting potential ❑ Na permeability decreases -90 due to inactivation of Na + channels EK 1 ms Membrane ❑ K permeability increases hyperpolarized due to opening of K+ channels mem. potential approaches E K Na+ channels After hyperpolarization of membrane following an action potential: K+ channels ❑ not always seen! ❑ There is increased K+ conductance due to delayed closure of K + channels Summary of Voltage and Conductance Changes 2 3 1 3 2 2 1 2 2 1 2 3 1 Figure 5-7 Figure 5-10 Ratio of conductances Figure 5-10 Refractory Periods Absolute refractory period – AP is not possible due to voltage inactivation of Na+ channels. Relative refractory period – greater than normal stimulus is required to elicit AP because of hyperpolarization. Refractory periods limit the maximum frequency of APs Propagation of Action Potential Opening of Na+ channels generates a local current that depolarizes the adjacent membrane, opening more Na+ channels… Rest Stimulated (local depolarization) Propagation (current spread) Figure 5-11 Signal Transmission Myelination Schwann cells surround the nerve axon forming a myelin sheath Sphingomyelin decreases membrane capacitance and ion flow 5,000-fold Sheath is interrupted every 1-3 mm by a node of Ranvier Figure 5-16 Saltatory Conduction AP’s only occur at the nodes (Na + and K + channels are concentrated here!) Increased velocity Energy conservation Figure 5-17 Conduction velocity - non-myelinated vs myelinated - non-myelinated myelinated Multiple Sclerosis - MS is an immune-mediated inflammatory demyelinating Patients have a difficult time describing their disease of the CNS - symptoms. They may present with paresthesias of a hand that resolves, followed in a couple of months by weakness in a leg or visual - About 1 person per 1000 in US is disturbances. Patients frequently do not bring thought to have the disease - The these complaints to their doctors because they female-to-male ratio is 2:1 - whites of have been resolved. Eventually, the resolution of northern European descent have the the neurologic deficits is incomplete or their highest incidence occurrence is too frequent, and the diagnostic dilemma begins. http://www.emedicine.com/pmr/topic82.htm Transmission at a chemical synapse vesicle of tx-R operated transmitter (tx) channel pre post [Ca2+] +++ +++ Na+ Ca2+ Na+, K+, Ca++ V-G Na+ channels V-G Ca2+ tx release open APpost tx-R APpre channels diffuse open [Ca] depolarization 0.5 ms UNIT 2 Chapter 6: Contraction of Skeletal Muscle Slides by Thomas H. Adair, PhD Copyright © 2021 by Saunders, an imprint of Elsevier Inc. Physiological Anatomy of Skeletal muscle Gross Organization Muscle fasciculus – a bundle of muscle fibers Muscle fibers are multinucleated cells diameter: 10-80 micrometers contain hundreds to thousands of myofibrils Figure 6-1 Physiological Anatomy of Skeletal Muscle Cellular Organization Muscle fibers single cells multinucleated surrounded by sarcolemma Sarcolemma – a membrane that encloses muscle fiber. Composed of: 1. true cell membrane 2. outer collagen coat that fuses with a tendon fiber Myofibrils contractile elements composed of actin and myosin filaments Figure 6-1 surrounded by sarcoplasm (cytoplasm) Cellular organelles - lie between myofibrils (mitochondria, sarcoplasmic reticulum, etc.) Physiological Anatomy of Skeletal muscle Cellular Organization (cont.) Physiological Anatomy of Skeletal muscle Molecular Organization - Dystrophin Dystrophin is a tubular protein that tethers cytoskeletal F-actin filaments to extracellular matrix (ECM) in skeletal muscle and heart. The dystrophin complex acts as a membrane stabilizer to prevent contraction-induced damage. A lack of functional dystrophin promotes sarcolemma tears and rupture along with progressive weakness, beginning in early childhood. Sarcolemma There are two main types of muscular dystrophy caused by mutations in the dystrophin gene (also called the DMD Gene). 1. Duchenne Muscular Dystrophy (DMD): most common X-Linked Dilated Cardiomyopathy (XLDC) is a 2. Becker Muscular Dystrophy (BMD): less third type of dystrophinopathy; it is allelic to Duchenne and Becker muscular dystrophies. The common and less severe incidence is unknown. DMD and BMC are X-linked recessive disorders; so, mostly males are affected. Incidence is 1 in every 5,600-7,700 males between the ages of 5 and 24 years. https://www.nature.com/articles/s41572-021-00248-3 (2021) Physiological Anatomy of Skeletal muscle Molecular Organization - Sarcomere The sarcomere is the smallest contractile unit of a muscle fiber. Figure 6-1 The Sarcomere sarcomere A band H zone Z disc M line I band thick filament (myosin) thin filament (actin) titin (filamentous structural protein) Titin Figure 6-3 Titin (also called connectin) is a springy, filamentous molecule. Large polypeptide (MW = 3 million; 34,350 AA) One end is attached to the Z disc and the other to the M band, so the titin molecule is one-half the length of the sarcomere. Function of Titin Acts as a molecular spring and prevents overstretching of sarcomere; it is responsible for the passive elasticity of muscles. Stabilizes thick myosin filaments, keeping them centered between actin filaments during contraction- relaxation cycle. Serves as adhesion template for assembly of contractile machinery Titin gene mutations: Mutations of the TTN gene can be associated with hereditary myopathy with early respiratory failure, early-onset in sarcomeres. myopathy with fatal cardiomyopathy, core myopathy with heart disease, centronuclear myopathy, limb-girdle muscular dystrophy type 2J, and familial dilated cardiomyopathy. Titin also participates in generation of active tension in skeletal muscle. Also, autoantibodies to titin are produced in patients with Myasthenia J Exp Biol 217: 2825-2833, 2014. gravis. Acta Neurol. Suppl. 183: 19–23, 2006. J Appl Physiol 126: 1474–1482, 2019. What are the striations? Z disc A band I band The striated pattern is due to the regular organization of the contractile proteins - within the entire fiber The sarcomere is the functional unit ~2.2 m long The banding pattern is only seen in polarized light or in stained tissue. ✓ ‘I’ - isotropic - appearing light Sarcomere 2.2m ✓ ‘A’ - anisotropic - appearing dark Figure 6-2 The sarcomere is made up of a complete A band light band dark band and the 2 halves of the I bands adjacent to it - Note that the Z disc delimits the sarcomere but is in the middle of an I band Z disc M line Z disc Relaxed and Contracted States – Which band shortens? Contraction results from sliding action of interdigitating actin and myosin filaments. Figure 6-5 Which band shortens - I or A? thin filament thick filament z discs Overall Mechanism of Muscle Contraction 1. Command from motor cortex. 2. Action potential (AP) travels along motor neuron to muscle fibers. 3. Acetylcholine (ACh) is released from motor neuron. 4. ACh-gated cation channels on muscle fiber membrane open, causing small depolarization (End-Plate Potential). 5. End-plate potential reaches threshold potential, causing voltage-gated sodium channels to open in muscle fiber membrane. 6. AP travels over muscle fiber membrane, causing release of calcium ions from sarcoplasmic reticulum. 7. Calcium ions bind to troponin facilitating attractive forces between actin and myosin filaments; muscle contraction follows. 8. Calcium ions are pumped back into sarcoplasmic reticulum, causing termination of contraction. The Actin Filament I band filament tethered at one end to Z disc 1 µm long and very uniform nebulin forms guide for synthesis Figure 6-7 Tropomyosin F-actin: covers active sites at rest double-stranded helix sterically hinders interaction with composed of polymerized G-actin myosin ADP bound to active sites on each G- actin Troponin myosin heads bind to active sites I - binds actin T - binds tropomyosin C - binds Ca2+ Calcium triggers contraction by exposing active sites on actin filaments Theory: Binding of Ca2+ to troponin results in a conformational change in tropomyosin that “uncovers” active sites on actin molecule; this allows myosin to bind to active sites. Active Site The Myosin Filament Composed of: two heavy chains (MW 200,000) four light chains (MW 20,000) “head” region - site of ATPase activity Figure 6-6 “Walk-Along” Theory of Muscle Contraction Figure 6-8 Myosin-Actin Cycling in the Presence of Ca2+ and ATP Myosin-Actin Cycling. Myosin reaches forward, binds to actin, tilts (muscle 2 3 contracts), releases actin, and then reaches forward again to bind actin in a new cycle. Actin ADP ADP Pi Pi Myosin-Actin Cycling. Myosin Weakly 11. ATP binds to myosin heads causing bound heads to detach from actin filaments. Pi Hydrolysis Powerstroke ADP 22. Hydrolysis of ATP causes myosin heads to “cock”; ADP and Pi are bound to heads. ATP + ATP 33. Myosin heads are weakly bound to actin 1 4 filaments with Pi and ADP still attached. Strongly bound 4 The release of Pi and ADP empowers 4. Rigor mortis: the tilting of myosin heads, called the state of contracture that occurs following death Powerstroke. myosin heads cannot detach from actin without ATP Nature Structural Biology 10: 773-75, 2003. https://www.nature.com/articles/nsb1003-773 Muscle Mechanics Tension as a function of sarcomere length Normal operating range Stress is used to compare tension (force) 1 generated by different-sized muscles – Stress = force/cross-sectional area of muscle; units kg/cm2) In skeletal muscle, maximal active active stress is developed at a normal resting stress length of ~ 2 m 0.5 (tension) At longer lengths, stress declines - At shorter lengths stress also declines - Cardiac muscle normally operates at lengths below optimal length - 0 0 1 2 3 4 sarcomere length (m) Tension as a function of sarcomere length Figure 6-9 Relationship of Contraction Velocity to Load No afterload: maximum velocity at minimum load A Increased afterload: contraction velocity decreases B contraction velocity is zero when afterload = max force of contraction Figure 6-11 A: larger, faster muscle (white muscle) B: smaller, slower muscle (red muscle) Isometric and Isotonic Contractions Isometric and isotonic contractions Isotonic contraction – Muscle shortens. Isometric contraction – Muscle does not shorten. Figure 6-12 Isometric and Isotonic Contractions Isometric and isotonic contractions Force Isometric Completely Transducer Isometric Isometric Isotonic Isotonic Force C B A Stimulator Stimulus Afterload A springs Length B C Time Muscle lever System Figure 6-16 A Single Muscle Twitch Muscle twitch - the contraction generated by a single AP. Latent Period – time between arrival of neuronal AP and start of contraction. Muscle fiber membrane depolarizes Calcium diffuses out of sarcoplasmic reticulum (SR) and binds to troponin Tropomyosin uncovers active sites on actin filaments Cross-bridge formation occurs Slack is taken out of the muscle prior to shortening Contraction Period. Muscle generates tension. Cycling of cross-bridges occurs. Relaxation Period is the time required for the muscle to return to its resting length. Types of Skeletal Muscle Speed of twitch contraction - The speed of twitch contraction is determined by Vmax of myosin ATPase. Type 1 fiber (slow, red) – low Vmax for myosin ATPase – slow cross-bridge cycling – slow rate of shortening Type 2 fiber (fast, white) – high Vmax for myosin ATPase – rapid cross-bridge cycling – rapid rate of shortening Figure 6-13 Most muscles contain both types of fibers, but proportions differ. Fast All fibers in a particular motor unit are the Slow same type, i.e., fast fibers or slow fibers. Stained for ATPase NOT MYOGLOBIN Types of Skeletal Muscle (cont.) Resistance to fatigue - Fast twitch and slow twitch fibers show Slow (red muscle) different resistances to fatigue. Slow fibers (Type I) - oxidative high resistance to fatigue small diameter high myoglobin content Force (% initial) high capillary density high mitochondrial content high oxidative enzyme content Fast (white muscle) Fast fibers (Type II) - glycolytic low resistance to fatigue large diameter low myoglobin content low capillary density low mitochondrial content 0 5 60 high glycolytic enzyme content time (min) Hereditary differences among athletes Fast-twitch slow-twitch Fast and slow (and intermediate) muscle fiber types can be identified by Marathon 18% 82% histochemistry. Runners In any muscle, there is a mixture of slow and fast fibers. Swimmers 26 74 Motor units containing Type 1 fibers are recruited first to power normal Average 55 45 contractions. man Type 2 fibers help out when particularly forceful contraction is required Weight 55 45 Different people have different Lifters proportions of fiber types. Sprinters 64 37 There is little evidence that training alters fiber proportions in humans. Jumpers 63 37 Long-term increases in muscular activity can change muscle fiber type in rats Type 2 fibers (upper panel) in anterior tibialis muscle can be converted to Type 1 fibers (lower panel) by a long-term increase in activity, i.e., electrical stimulation via motor nerve for 60 days (10 Hz, 2 hrs stim/4 hrs rest). Before Exercise (upper panel) – predominantly Type 2 fibers – stains light: few left AT mitochondria – low capillary density – small capillaries – large fibers After Exercise (lower panel) – predominantly Type 1 fibers – stains dark: many mitochondria right AT – high capillary density – large capillaries – small fibers Motor Unit A motor unit is a collection of muscle fibers innervated by a single motor neuron. Figure 6-14 Motor Unit All fibers are the same type (fast or slow) in each motor unit. Ask yourself why? Small motor units (e.g., larynx, extraocular) − as few as 10 fibers/unit − precise control − rapid reacting Large motor units (e.g., quadriceps muscles) as many as 1000 fibers/unit coarse control slower reacting Overlap of motor units provides coordination. There is not a good relation between fiber type and size of a motor unit. Force Summation of Muscle Contraction Force summation is the increase in contraction intensity that results from the additive effects of individual twitch contractions. (1) Multiple fiber summation results from an increase in number of motor units that contract simultaneously (fiber recruitment). Size principle: When a weak signal is sent by Figure 6-15 CNS to contract a muscle, the smaller motor units (that mostly (2) Frequency summation results from innervate Type I fibers), being more an increase in the frequency of excitable than the larger ones (that contraction of individual motor units. mostly innervate Type II fibers), are stimulated first. Frequency summation of twitches and tetanus Myoplasmic [Ca2+] What kind of summation is shown? Fused tetanus Force AP Time (1 second) Myoplasmic (sarcoplasm) Ca2+ falls (initiating relaxation) before the development of maximal contractile force If the muscle is stimulated before complete relaxation has occurred, the new twitch will add to the previous one, etc. If action potential frequency is sufficiently high, the individual contractions are not resolved, and a ‘fused tetanus’ contraction is recorded. What causes muscle tone? Toned muscles Your muscles are in a constant state of partial contraction, which keeps them firm. This is called muscle tone. Even when a muscle is relaxed, nerve impulses from your brain stimulate groups of muscle fibers within it to contract and keep your muscle toned. Also, signals originate in muscle spindles. This is involuntary, but regular exercise clearly increases muscle tone. Flaccid muscles If the nerve supply to a muscle is destroyed, its muscle fibers are no longer stimulated to contract. This causes the muscle to lose its tone and become flaccid, and will eventually start to waste away. Muscle Remodelling - growth Hypertrophy (common, weeks) – Caused by near maximal force development hypertrophy (e.g., weight lifting) – Increase in actin and myosin – Myofibrils split Hyperplasia (rare) – Formation of new muscle fibers – Can be caused by endurance exercise training Hypertrophy and hyperplasia – Increased force generation – No change in shortening capacity or maximum velocity of contraction Lengthening (normal) lengthening – Occurs with normal growth – No change in force development – Increased shortening capacity hyperplasia – Increased maximum contraction velocity Why does increasing the number of sarcomeres in series lead to a faster velocity of shortening? Sarcomeres A and B have individual contraction velocities of 1 m/s. A. B. If sarcomere B does not contract, its end will still move with a velocity of 1 m/s, which is the contraction velocity of sarcomere A. Now, if sarcomere B also contracts with a velocity of 1 m/s, the two sarcomeres together will have a shortening velocity of 1 + 1 = 2 m/s, etc. Eight sarcomeres in series has twice the velocity of shortening as four sarcomeres in series. See animation below. Muscle Remodelling - atrophy Atrophy Causes of atrophy – Denervation/neuropathy – Tenotomy – Sedentary life style weeks – Plaster cast – Space flight (zero gravity) Muscle performance – Degeneration of contractile proteins – Decreased max force of contraction – Decreased velocity of contraction Atrophy with fiber loss Many months/ – Disuse for 1-2 years years – Very difficult to replace lost fibers atrophy with fiber loss UNIT 2 Chapter 7: Excitation of Skeletal Muscle: Neuromuscular Transmission and Excitation-Contraction Coupling Slides by Thomas H. Adair, PhD Copyright © 2021 by Saunders, an imprint of Elsevier Inc. Motor neuron synapses on skeletal muscle fibers nmj Neuromuscular Junction (NMJ) Figure 7-1A NMJ is a synaptic connection between terminal end of motor neuron and motor end-plate of muscle fiber. There is usually only one NMJ for each muscle fiber. Motor end-plate is located directly across synaptic cleft from presynaptic axon terminal. Neuromuscular Junction (Cont.) Synaptic cleft: Synaptic trough: is an − 20-30 nm wide invagination in the motor end- − contains large quantities plate membrane of acetylcholinesterase (AChE) Subneural clefts: − increase surface area of post-synaptic membrane − ACh-gated channels are located at tops of clefts − Voltage-gated Na+ channels are in bottom part of clefts Figure 7-1C Structural Reality Axon myofibril The Motoneuron – vesicle formation Synaptic vesicles formed in Golgi apparatus are transported to nerve terminal by “axoplasmic streaming”; there are ~300,000 vesicles per terminal. Acetylcholine (ACh) is formed in cytoplasm at terminal end of neuron where it is transported into vesicles. There are ~10,000 molecules of ACh per vesicle. ACh filled vesicles often fuse with pre-synaptic membrane (spontaneously without AP) and release contents into synaptic cleft; this causes miniature end-plate potentials (MEPP) in post-synaptic membrane. MEPPs do not cause muscle contraction. The Motoneuron - ACh Release 3 1. The action potential begins in the ventral horn of the spinal cord. 1 2. Local depolarization opens voltage- AP gated Ca2+ channels at the nerve terminal. 3. An increase in cytosolic Ca 2+ triggers the fusion of ~125 synaptic vesicles with the pre-synaptic membrane; ACh is released by 2 exocytosis. Ca2+ ACh Release - details Ca2+ channels are localized around linear structures on pre-synaptic membrane called dense bars. Vesicles fuse with membrane in region of dense bars. The dense bars are thought to align vesicles in preparation for exocytosis. ACh receptors are located at tops of subneural clefts. Voltage-gated Na+ channels are in bottom halves of subneural clefts. Figure 7-2 Acetylcholine-Gated Channel The ACh-gated channel is a cation channel that does not differentiate between sodium, potassium, or calcium; each ion moves down its electrochemical gradient as shown. Ca ++ The net equilibrium potential of all three cations averaged together is ~0 mV. K+ Figure 7-3 End plate potential and action potential - at the motor endplate - ACh released into neuromuscular junction binds to and opens nicotinic ACh receptor channels (nACh) on muscle fiber membrane increasing permeability to cations (Na+, K+, and Ca2+). The opening of nACh receptor channels produces an end-plate potential. Under mV normal conditions, the end-plate potential is always sufficient to open voltage-gated 40 sodium channels. 0 What terminates the process? -40 Acetylcholinesterase (AChE) -80 0 15 30 45 60 75 nAChr mSec Na channel Miniature End-Plate Potential and End-Plate Potential Miniature End-Plate Potential An MEPP is about 0.5 mV as shown. Vesicles containing ACh collide spontaneously with membrane at nerve terminal and release ACh into neuromuscular junction. MEPPs occur without an action potential. MEPPs do not cause muscle to contract. Note that a typical end-plate potential is about 20 mV, and that a typical MEPP is about 0.5 mV. Drug Effects on End Plate Potential - Inhibitors - Curariform drugs (D-turbocurarine) “Normal” blocks nicotinic ACh channels by competing for ACh binding sites on nACh receptors reduces amplitude of end-plate curare botulinum toxin potential preventing AP in muscle membrane. Botulinum toxin decreases release of ACh from nerve terminals Figure 7-4 end-plate potential is too small to initiate AP Drug Effects on End-Plate Potential - Stimulants ACh-like drugs (methacholine, carbachol, nicotine) bind to and activate nACh receptors not destroyed by AChE, so their effects are prolonged Anti-AChE drugs (neostigmine, physostigmine, Di- isopropyl fluorophosphate or “nerve gas”) block degradation of ACh prolong effects of ACh Myasthenia Gravis (MG) Incidence / symptoms: Muscle paralysis can be lethal in extreme cases when respiratory muscles are involved. Prevalence in U.S. is 14-20 per 100,000 people; it is likely to be underdiagnosed. https://myasthenia.org/ Cause: MG is an autoimmune disease caused by IgG antibodies that damage or destroy nACh receptors on muscle fiber membrane. The result is weak end-plate potentials. Treatment: Sometimes MG can be ameliorated by the anti-AChE drug neostigmine; this can increase the amount of ACh in neuromuscular junction. Suppression of immune system with steroids. Vyvgart (efgartigimod) – a new treatment that lowers ALL IgG antibodies. Plasmapheresis (plasma exchange removes all antibodies). Lambert-Eaton Myasthenic Syndrome (LEMS) Incidence / symptoms: A rare disease; about 400 known cases in United States. 40% also have small cell lung cancer. Major symptom is muscle weakness/paralysis. Cause: Results from autoimmune attack against voltage-gated calcium channels on presynaptic motor nerve terminal. A decrease in release of ACh results in weak end-plate potentials. Treatment: Can be treated with anti-AChE drugs like neostigmine. Chemotherapy with radiation therapy. Plasmapheresis is not as effective as with MG. Excitation-Contraction Coupling Transverse tubule / SR System T-tubules: Invaginations of the sarcolemma filled with extracellular fluid Penetrate the muscle fiber, branch and form networks Transmits AP’s deep into the muscle fiber Sarcoplasmic Reticulum: terminal cisternae and longitudinal tubules terminal cisternae form junctional “feet” adjacent to the T- tubule membrane intracellular storage compartment for Ca2+ Figure 7-5 EC Coupling - Overview Figure 7-7 EC Coupling - the “Triad” the junction between two terminal cisternae and a T- tubule dihydropyridine receptor: it’s a T-tubule Terminal voltage sensor cisterne of SR ryanodine Ca2+ release channel EC Coupling – Skeletal muscle Figure 7-6 EC Coupling – how it works (skeletal muscle) AP Sequence of Events: 1. AP moves along T-tubule 2. The voltage change is sensed by Ca2+ pump the DHP receptor 3. is communicated to the ryanodine receptor which opens. (VACR) 4. Contraction occurs 5. Calcium is pumped back into SR. Calcium binds to calsequestrin to facilitate storage. 6. Contraction is terminated. calsequestrin EC Coupling – Cardiac muscle Ca2+/Na+ exchanger (Ca2+ out / Na+ in) AP Sequence of Events: 1. AP moves along T-tubule Ca2+ pump 2. Voltage-gated L-type Ca2+ requires ATP channels are activated, releasing Ca2+ into the sarcoplasm of the fiber; these channels are also called slow-calcium channels and calcium-sodium channels. 3. Ca2+ binds to the ryanodine receptor causing it to open. A large amount of Ca2+ is released. (Ca2+ activated Ca2+ release) 4. Ca2+ is pumped (a) back into SR, calsequestrin and (b) back into T-tubule. 5. Contraction is terminated. EC Coupling - Comparison Skeletal Muscle Cardiac Muscle 1. The trigger for SR release of 1. The trigger for SR release of Ca2+ is voltage (Voltage Ca2+ is calcium (Calcium Activated Calcium Release - Activated Calcium Release – VACR). CACR). 2. The T-tubule membrane has a 2. The T-tubule membrane has a voltage sensor (DHP receptor) voltage-gated L-type Ca2+ channel 3. The ryanodine receptor is the SR 3. The ryanodine receptor is the SR Ca2+ release channel (RYR-1) Ca2+ release channel (RYR-2) 4. Ca2+ release is proportional to 4. The ryanodine receptor is Ca 2+ the frequency of action potentials gated and Ca2+ release is proportional to Ca2+ entry. 5. Source of Ca2+: sarcoplasmic 5. Source of Ca2+: sarcoplasmic reticulum only reticulum and T-tubule (ECF) Clinical Oddity: Malignant Hyperthermia Symptoms: Malignant hyperthermia is a hypermetabolic crisis Skeletal muscle rigidity High fever Tachycardia – Tachypnea Lactic acidosis (hypermetabolism) Cause: Unregulated release of Ca 2+ within muscle fiber Triggered by succinylcholine and halogenated anesthetics (e.g., isoflurane, halothane) Genetic mutation – at lease 6 genetic mutations of ryanodine receptor or dihydropyridine receptor genes. Can be tested for by muscle biopsy Constant leak of Ca2+ from SR Why is so much heat generated? Treatment: Rapid cooling Ans: our bodies are only about 45% Administration of dantrolene (antagonizes energy efficient, 55% of the energy ryanodine receptors which inhibits Ca 2+ appears as heat. release) UNIT 2 Chapter 8: Excitation and Contraction of Smooth Muscle Slides by Thomas H. Adair, PhD Copyright © 2021 by Saunders, an imprint of Elsevier Inc. Types of Smooth Muscle Mononucleate cells with no striations 100 µm (appear smooth in polarized light). There are sorts of organization of SM 1. Unitary or visceral SM: sheets of cells are coupled electrically via gap junctions. Cells contract in unison and are often spontaneously active. Include SM of the gut, airways, blood vessels, and urogenital system. 2. Multiunitary SM: discrete bundles of cells are densely innervated and contract only in response to innervation (e.g., vas deferens, iris, piloerectors) Figure 8-1 Special features of smooth muscle Smooth muscle: intermediate filament (form structural backbone) Can operate over a large range of lengths (60 - 75% shortening possible) Is very energy efficient (O2 dense body (membrane consumption is ~1% of the same dense area analogous to weight of skeletal muscle at the same Z discs) tension!) Can maintain force for long periods (hours, days, weeks) via latch state Can be myogenic (spontaneously mechanical active) junction Has Ca2+ action potentials. (coupling cells) Ca2+ entering through channels in the gap junction cell membrane is a very important source of Ca2+ (like cardiac muscle and unlike skeletal muscle). Bell-shaped length-tension curve Poorly developed SR Figure 8-2 Smooth Muscle Neuromuscular Junction Major points Less complex and less well understood compared to NMJ in skeletal muscle. Autonomic nerve fibers branch and form “diffuse junctions” with underlying smooth muscle fibers. Varicosities in the terminal axons contain neurotransmitters. Neurotransmitter is secreted into the matrix coating and diffuses to the muscle cells. Excitation is transmitted by Ca 2+ action potential or simple diffusion of Ca2+ into a fiber without an action potential. Also, Ca++ can Figure. 8-6 be released from the SR. Smooth muscle - EC coupling Contraction occurs by the same actin-myosin interaction as in striated muscle (sliding filament). The troponin complex is absent. Calmodulin is very similar in structure, and also binds Ca++. Regulation of contraction is myosin-based, not actin-based as in skeletal and cardiac muscle. Myosin does not hydrolyse ATP (to become energized) unless it is first phosphorylated (on the regulatory light chain) The enzyme myosin light chain kinase (MLCK) phosphorylates the light chain. This step is Ca++ sensitive - How? MLCK is active only in the presence of a small Ca2+-binding protein called calmodulin (and only when it has Ca2+ bound). Contraction-Relaxation – myosin-based regulation Ca2+ Major points: 1. Initiated by Ca2+ from ECF or SR. 2. Ca2+ binds to calmodulin (instead of SR Ca2+ Ca2+ troponin as in skm). 3. Ca2+-calmodulin-MLCK complex leads calmodulin to phosphorylation of MLC (requires 1 Relaxation ATP). 4. MLC is part of myosin head 5. Phosphorylated myosin head binds to actin and power stroke occurs MLC inactive automatically. (dephosphorylated) 6. A second ATP is required to release MLCP MLCK myosin head from actin. 7. Cross-bridge cycling requires both MLC active MLCK and MLCP. (phosphorylated) 8. MLCP activity is regulated and can change Ca2+ sensitivity (i.e., if MLCP activity is high, contraction will be attenuated in the face of high [Ca2+]i. Contraction MLCK, myosin light chain kinase MLCP, myosin light chain phosphatase Control of SM is Diverse 1. Endocrine: 2. Paracrine: 3. Local nervous 4. Autonomic nervous system: system: Epinephrine Histamine Enteric system of Norepinephrine relaxes gut and stimulates the gut – more on sometimes relaxes airway muscle, gastric this later in sometimes contracts. and constricts contraction. course. blood vessels. Extracellular Acetylcholine sometimes Cholecystokinin [K+], nitric relaxes sometimes stimulates oxide, CO 2, contracts. contraction of adenosine gall bladder. mediate Angiotensin II relaxation of and antidiuretic blood vessels. hormone constrict blood vessels. UNIT 3 Chapter 9: Cardiac Muscle; The Heart as a Pump and Function of the Heart Valves Copyright © 2021 by Saunders, an imprint of Elsevier Inc. Structure of the Heart The epicardium and visceral serous pericardium are the same thing. Figure 9-1 Overview of circulation What are functions? Atria / ventricles R. heart – volume pump R.A. L.A. L. heart – pressure pump LUNGS BODY Valves R.V. L.V. Semi-lunar valves Pulmonary Aortic A-V valves Mitral Tricuspid Syncytial nature of cardiac muscle Fibers are arranged in series and in parallel Intercalated disks Gap junctions Electrical resistance Syncytia of heart What is a syncytium? Atrial/Ventricular syncytia. What separates syncytia? What connects syncytia? Figure 9-2 Action Potentials of Muscle and Purkinje Fibers Intracellular recordings show 200 ms plateau for ventricular muscle AP. Skeletal muscle AP is 1 to 5 ms Figure 9-4 Ventricular muscle action potential 1 2 0 +20 Phase 0 (depolarization) 0 Membrane -20 Rapid inward Na+ current potential -40 0 3 (mV) Phase 1 (early repolarization) -60 4 1 -80 Fast Na+ channels close -100 Brief elevation in outward K+ current Relative High 2 Phase 2 (plateau period) Potassium Increased inward Ca2+/Na+ current Conductance Low Decreased outward K+ current High Relative 3 Phase 3 (repolarization) Sodium Decreased inward Ca2+/Na+ current Conductance Increased outward K+ current (Fast Na+ channel) 4 Phase 4 (Resting potential) Low Relative The following do not occur in skeletal muscle: High Ca2+/Na+ Decreased K+ conductance Conductance Increased Ca2+ conductance (important (L-Type Ca2+ source of Ca2+ for contraction in cardiac channel) Low muscle). 0 100 200 300 400 Plateau period Time (msec) Refractoriness of Heart Muscle Tension Skeletal muscle AP ARP RRP Tension AP Absolute refractory period (ARP) Relative refractory period (RRP) AP cannot be initiated during ARP Greater than normal stimulus is ARP is similar to duration of contraction required to initiate AP Prevents tetanus in heart muscle EC Coupling – Cardiac muscle Ca2+/Na+ exchanger (Ca2+ out / Na+ in) AP Sequence of Events: 1. AP moves along T-tubule Ca2+ pump 2. Voltage-gated L-type Ca2+ requires ATP channels are activated, releasing Ca2+ into the sarcoplasm of the fiber; these channels are also called slow-calcium channels and calcium-sodium channels. 3. Ca2+ binds to the ryanodine receptor causing it to open. A large amount of Ca2+ is released. (Ca2+ activated Ca2+ release) 4. Ca2+ is pumped (a) back into SR, calsequestrin and (b) back into T-tubule. 5. Contraction is terminated. EC Coupling – Cardiac muscle (cont.) Detection of Ca2+ spark. Calcium causes Fluo-4 to fluoresce (i.e., spark); seen with confocal microscope. Also present in skeletal muscle but not as striking. Figure 9-7 EC Coupling – Cardiac muscle (cont.) Source of calcium Sarcoplasmic reticulum Transverse tubules (T-tubules) Cardiac T-tubules High volume: 25x greater volume compared to skeletal muscle Store calcium - negatively charged mucopolysaccharides bind Ca2+ The strength of ventricular contraction is dependent upon extracellular calcium concentration. What about skeletal muscle? EC Coupling - Comparison Skeletal Muscle Cardiac Muscle 1. The trigger for SR release of 1. The trigger for SR release of Ca2+ is voltage (Voltage Ca2+ is calcium (Calcium Activated Calcium Release - Activated Calcium Release – VACR). CACR). 2. The T-tubule membrane has a 2. The T-tubule membrane has a voltage sensor (DHP receptor) voltage-gated L-type Ca2+ channel 3. The ryanodine receptor is the SR 3. The ryanodine receptor is the SR Ca2+ release channel (RYR-1) Ca2+ release channel (RYR-2) 4. Ca2+ release is proportional to 4. The ryanodine receptor is Ca 2+ the frequency of action potentials gated and Ca2+ release is proportional to Ca2+ entry. 5. Source of Ca2+: sarcoplasmic 5. Source of Ca2+: sarcoplasmic reticulum only reticulum and T-tubule (ECF) Cardiac cycle – Wiggers Diagram LEFT VENTRICLE Systole 1. Isovolumic contraction 2. Ejection Diastole 1. Isovolumic relaxation 2. Rapid inflow 3. Diastasis 4. Atrial systole Heart sounds – caused by valve closure (see Ch. 23) 1st heart sound: A-V valves 2nd heart sound: semilunar valves "lub" "dub" "lub" "dub" Figure 9-8 Heart Valves Valve Location Isovolumic Systole Isovolumic Diastole Contraction Relaxation Mitral LA - LV Closed Closed Closed Open Tricuspid RA - RV Closed Closed Closed Open Aortic LV - Aorta Closed Open Closed Closed Pulmonary RV - PA Closed Open Closed Closed Valves Semi-lunar valves: Pulmonary and Aortic A-V valves: Mitral and Tricuspid Closure Sounds A-V valves: S1, soft closure, LUB Semi-lunar valves: S2, rapid snap, DUB Papillary muscles Contract when ventricles contract They do not help the valves to close Prevent vanes of valves from bulging Figure 9-9 into atria Cardiac cycle Systole 1. Isovolumic contraction 2. Ejection Diastole 1. Isovolumic relaxation 2. Rapid inflow 3. Diastasis 4. Atrial systole Isovolumic states: all valves closed Heart sounds – valve closure 1st heart sound: A-V valves 2nd heart sound: semilunar valves Ventricular volumes (cont.) EDV: End Diastolic Volume LV-EDV SV: Stroke Volume 120 mL ESV: End Systolic Volume EF: Ejection fraction SV 70 mL SV = EDV – ESV (120 – 50 = 70 mL) EF = SV / EDV (70 / 120 ≈ 0.6) Low EF Ejection fraction is used clinically to assess cardiac LV-ESV pumping capability. 50 mL 20 mL SV = 120 - 20 = 100 mL EF = 100 / 120 ≈ 0.8 An echocardiogram is the most common Lifestyle changes to improve ejection method to estimate fraction include: ejection fraction. Eat a heart-healthy, low-sodium diet. Quit smoking (or don't start) Avoid alcohol or reduce intake. Exercise regularly, such as daily walks. Get to and stay at a healthy weight. Aortic and Atrial Pressure Curves Systolic pressure – 120 mmHg Incisura Diastolic pressure – 80 mmHg Incisura (aka, dicrotic notch) Occurs with aortic valve closure. Caused by short period of backward flow immediately before valve closure, followed by the sudden cessation of backflow. Absent with aortic regurgitation Pressure changes in the atria: a wave: atrial contraction c wave: contracting ventricles cause A-V valves to bulge backward toward atria v wave: slow flow of blood into atria and then rapid entry of blood into ventricle when A-V valves open Questions Questions 1-6. Match each of the following cardiovascular events with its correct place in the cardiac cycle. 1. Aortic valve opens 2. Left ventricle is filling 3. Isovolumic contraction of left ventricle 4. Aortic valve closes here. S2 is produced 5. Atrial systole 6. Isovolumic relaxation of left ventricle 7. Mitral valve opens Frank-Starling Mechanism Definition: Within physiological limits, the heart can pump all of the blood that comes to it without allowing excessive damming of blood in the veins. How does it work? (improved interdigitation of actin-myosin filaments) L.V. L.V. Normal venous return Increased venous return (suboptimal interdigitation) (optimal interdigitation) Cardiac function curve Provides an indication of heart’s pumping ability Frank-Starling Mechanism (normal curve) Based on length-tension relationship Independent of innervation Independent of contractility Matches CO to VR. CO can double without increasing HR. Questions The graph shown depicts the relationship between stroke volume (SV) and end-diastolic volume (EDV). Match each of the following effects to the proper area of the graph. a. A to B b. A to C c. A to D d. D to C e. D to B 1. Indicates an increase in preload only 2. Results of injection of epinephrine only 3. Occurs during beta-1 blockade 4. Occurs during moderate exercise Myocardial contractility Definition: the ability to develop force at a given sarcomere length, i.e., at a given amount of actin-myosin interdigitation. Increased contractility estimates: Increased ejection fraction (SV/EDV) Increased peak systolic pressure Increased dP/dT or (dP/dT)/P Factors that increase contractility (+ inotropic effect): Increased heart rate Sympathetic stimulation ↑iCa2+ Cardiac glycosides (digoxin, digitalis) Increased extracellular calcium concn. Factors that decrease contractility (– inotropic effect): Decreased heart rate Decreased sympathetic stimulation ↓iCa2+ Parasympathetic stimulation Decrease extracellular calcium concn. UNIT 3 Chapter 10: Rhythmical Excitation of the Heart Copyright © 2021 by S