Bioquímica 2 Exam 3 PDF
Document Details
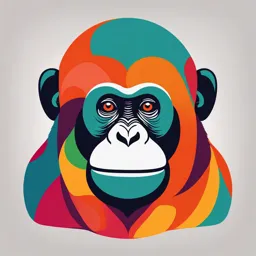
Uploaded by AdroitDramaticIrony324
Dr. Carla Ortiz, ND
Tags
Summary
This document provides an overview of protein digestion, nitrogen metabolism, and amino acid absorption. It details the primary role of dietary proteins, the mechanisms of protein synthesis and degradation, discusses protein digestion in the stomach and small intestine, and touches upon the absorption of amino acids. The document also examines the source of energy within amino acids, the role of the liver in oxidation and the different types of digestion, including endo- and exopeptidases.
Full Transcript
NMDP 734 Biochemistry II: Protein Digestion Dr. Carla Ortiz, ND Naturopathic Medicine Program Nitrogen Metabolism: Protein Digestion and Amino Acid Absorption Dietary proteins are the primary source of the nitrogen that is metabolized by the body, thus all the nitrogen-containing com...
NMDP 734 Biochemistry II: Protein Digestion Dr. Carla Ortiz, ND Naturopathic Medicine Program Nitrogen Metabolism: Protein Digestion and Amino Acid Absorption Dietary proteins are the primary source of the nitrogen that is metabolized by the body, thus all the nitrogen-containing compounds of the body are synthesized from amino acids. Proteins are constantly being synthesized and degraded, partially draining and then refilling the cellular amino acid pools. Compounds derived from amino acids include cellular proteins, non-steroidal hormones, purines/pyrimidines, neurotransmitters, the heme of hemoglobin and cytochromes, creatine phosphate, and the skin pigment melanin. Source of energy Amino acid carbons may be oxidized directly or The aa carbons are converted to glucose and then oxidized or stored as glycogen. The aa carbons may be converted to ketone bodies and released into the blood to be oxidized by other tissues. The aa carbons may also be converted to FAs and stored as adipose TGs. Ultimately, these carbons are converted to CO2 and H2O during oxidative phosphorylation inside mitochondria. Source of energy The liver is the major site of amino acid oxidation. However, most tissues can oxidize the branched-chain amino acids (leucine, isoleucine, and valine). Most of the aa carbons are converted to: pyruvate (gluconeogenesis) intermediates of the tricarboxylic acid cycle (anaplerotic rxns) acetyl coenzyme A (oxidation, ketogenesis, lipogenesis) Protein Digestion The enzymes that digest proteins are produced by stomach and pancreas as inactive precursors (zymogens) being secreted into the lumen of the digestive tract, where they are cleaved to smaller forms that have proteolytic activity. Proteases break down dietary proteins into their constituent amino acids in the stomach and the intestine. Endopeptidases vs Exopeptidases (see figure 35.3) Stomach: pepsin begins the digestion of proteins by hydrolyzing them to smaller polypeptides. Small intestine: pancreatic proteases (trypsin, chymotrypsin, elastase, and the carboxypeptidases) cleave the polypeptides into oligopeptides and amino acids. Further cleavage of the oligopeptides to amino acids is accomplished by aminopeptidases on the brush border. Digestion in Stomach Chief cells secrete pepsinogen (a zymogen) Parietal cells secrete hydrochloric acid (HCl) HCl activates autocatalytic conversion of pepsinogen into pepsin Dietary proteins are denatured at gastric low pH (Benefit?) Who activates the secretion of digestive enzymes (incl. proteases) by pancreatic acinar cells? Digestion in Small Intestine Duodenum: pancreatic juice adds proteases Brush-border cells secrete enteropeptidase, which then cleaves trypsinogen into trypsin Trypsin produces active chymotrypsin, elastase, and carboxypeptidase Smaller peptides formed by the action of endopeptidases (trypsin, chymotrypsin, and elastase) are attacked by exopeptidases (carboxypeptidases and aminopeptidases) Absorption by IECs Amino acids are absorbed from the intestinal lumen through secondary active sodium(Na+)-dependent transport and into blood through facilitated diffusion. As with glucose transport, the Na+ -dependent carriers of the apical membrane of the intestinal epithelial cells are also present in the renal epithelium. During starvation, the intestinal epithelia, like other cells, take up amino acids from the blood to use as an energy source. Thus, amino acid transport across the serosal membrane is bidirectional. Amino Acid Pool Replenishment Proteins are recycled within cells. Examples of proteins that undergo extensive synthesis and degradation (high turnover) are hemoglobin, muscle proteins, and digestive enzymes. Adults cannot increase the amount of muscle or other body proteins by eating an excess amount of protein. Why? If dietary protein is consumed in excess of our needs, it is converted to glycogen and TGs, which are then stored. Protein Turnover Lysosomal proteases (cathepsins) degrade proteins that enter lysosomes. Cytoplasmic proteins targeted for turnover are covalently linked to the small protein ubiquitin (a marker), which then interacts with a large protein complex, the proteasome, for degradation of the target protein. The amino acids released from proteins during turnover can then be used for the synthesis of new proteins or for energy generation. Mechanisms of protein degradation: Autophagy - cytoplasm is sequestered into vesicles and delivered to the lysosomes where degradation occurs by lysosomal enzymes (cathepsins) The recycled amino acids can then leave the lysosome and rejoin the intracellular amino acid pool. Ubiquitin–Proteasome Pathway - Ubiquitin-tagged proteins interact with the proteasome, which degrades proteins in an ATP- dependent process Nitrogen Metabolism Before the carbon skeletons of amino acids are oxidized, the nitrogen must be removed. Branched-chain amino acids can be oxidized in many tissues, but the nitrogen must always travel to the liver for disposal. Amino acid nitrogen forms ammonia, which is toxic to the body. In the liver, ammonia and the amino groups from amino acids are converted to urea, which is nontoxic, water-soluble, and readily excreted in the urine. This is known as the urea cycle. Uric acid is the degradation product of the purine bases. Creatinine is produced from metabolized creatine phosphate (a.k.a. phosphocreatine = storage form of phosphate in muscle). Ammonia is released from glutamine, particularly by the kidney, where it helps to buffer the urine by reacting with protons to form ammonium ions (NH4+). These compounds are excreted mainly in the urine, but substantial amounts are also lost in the feces and through the skin. Small amounts of nitrogen-containing metabolites (formed from the degradation of neurotransmitters and hormones) are excreted in the urine. Some of these degradation products, such as bilirubin (formed from the degradation of heme), are excreted mainly in the feces. N I T R O G E N M E TA B O L I S M Clinical Note Kwashiorkor, a common problem of children in Third World countries, is caused by a deficiency of protein in a diet that is adequate in calories. Children with kwashiorkor suffer from muscle wasting and decreased concentration of plasma proteins, particularly albumin. The result is an increase in interstitial fluid that causes edema and a distended abdomen, which makes the children appear “plump”. References Lieberman, M. A., Peet, A. (2018). Mark’s Basic Medical Biochemistry. A Clinical Approach (5th ed.). Wolters Kluwer: Philadelphia, PA. NMDP 734 Biochemistry II: The Urea Cycle Dr. Carla Ortiz, ND Naturopathic Medicine Program REVIEW During fasting, muscle protein is cleaved to aa. Some of the aa are partially oxidized to produce energy. Some of the aa are first converted to alanine and glutamine. Alanine and glutamine, along with other aa, are released into the blood. Alanine and the other aa travel to the liver, where their carbons are converted to glucose and ketone bodies, and the nitrogen is converted to urea. Glutamine is oxidized by various tissues, including the lymphocytes, gut, and kidney, which convert it to alanine. In the liver, kidney, or intestines, a glutaminase will remove the nitrogen from glutamine to form glutamate & ammonia. *Will be discussed later in detail. In the kidney, the release of ammonia leads to the formation of ammonium ion (NH?), which serves to form salts with metabolic acids in the urine. In the intestine, glutamine is used as a fuel. Clinical Note #1 During fasting, the liver maintains blood glucose levels. Amino acids from muscle protein are a major carbon source for the production of glucose by the pathway of gluconeogenesis. As amino acid carbons are converted to glucose, the nitrogens are converted to urea. Thus, the urinary excretion of urea is high during fasting. Aspartate Transamination, done by transaminases transaminase a.k.a. or aminotransferases, is the major Aspartate aminotransferase process for removing nitrogen from aa. (AST) In a transamination reaction, an amino group from one aa becomes the amino group of a second aa. Pyridoxal phosphate (PLP), derived from vitamin B6, is required as a cofactor. Alanine transaminase a.k.a. Alanine aminotransferase alanine (ALT) pyruvate alpha-ketoglutarate glutamate Example: Transamination of Alanine Transamination reactions are readily reversible, so they can be used to: ✓ remove nitrogen from amino acids ✓ transfer nitrogen to α-keto acids to form amino acids Thus, they are involved both in amino acid degradation and in amino acid synthesis. All amino acids except lysine and threonine undergo transamination reactions. When amino acids are degraded, glutamate collects the nitrogen through transamination. Pyruvate is available in muscle (from glycolysis) where it can serve as the precursor for alanine production. When amino acids are degraded and urea is formed, glutamate collects nitrogen from other amino acids through transamination. L I V E R Glutamate can release ammonia through the glutamate dehydrogenase reaction. *GDH can use either NAD+ or NADP+ This process provides one source of the ammonia that enters the urea cycle. Glutamine is synthesized from glutamate by the fixation of ammonia, requiring energy (ATP) and the enzyme glutamine synthetase. Clinical Note #2 Six amino acids are metabolized in resting muscle: leucine, isoleucine, valine, asparagine, aspartate and glutamate. BCAA oxidation has been estimated to supply a maximum of 20% of the ATP supply of resting muscle. Oxidation of BCAAs in muscle serves two functions. The first is the generation of ATP, and the second is the synthesis of glutamine, which effluxes from the muscle. The highest rates of BCAA oxidation occur under conditions of acidosis, in which there is a higher demand for glutamine to transfer ammonia to the kidney. Protein turnover and amino acid degradation occur in all tissues; however, the urea-cycle enzymes are active primarily in the liver (the intestine expresses low levels of activity of these enzymes). Alanine and glutamine are the major carriers of nitrogen in the blood. There are three mammalian enzymes that can “fix” ammonia into organic molecules: 1. Glutamate dehydrogenase (GDH) 2. Glutamine synthetase 3. Carbamoyl phosphate synthetase I (CPSI) The Urea Cycle Inside hepatic mitochondria: 1. (CPSI) Carbamoyl phosphate synthase I 2. (OTC) Ornithine transcarbamoylase Hepatic cytosol: 3. Argininosuccinate synthetase 4. Argininosuccinate lyase 5. Arginase Bacterial production of NH4+ To some extent, humans excrete urea into the gut and saliva. Urea is not cleaved by human enzymes. Bacteria in the human digestive tract, can cleave urea to ammonia and CO2 , using the enzyme urease. This ammonia, produced by our microbiota, is absorbed into the hepatic portal vein and extracted by the liver to be converted to urea. Approximately one fourth of the total urea released by the liver each day is recycled by bacteria. Regulation of The Urea Cycle Allosteric activation of CPSI by N-acetylglutamate (NAG) NAG is formed specifically to activate CPSI; it has no other known function in mammals. The synthesis of NAG from acetyl coenzyme A (acetyl- CoA) and glutamate is stimulated by arginine. Clinical Note #3 Disorders of the urea cycle are dangerous because of the accumulation of ammonia in the circulation, which is toxic to the nervous system. Ammonia toxicity will lead to brain swelling, caused, in part, by an osmotic imbalance owing to high levels of both ammonia and glutamine in the astrocytes. Also, ammonia toxicity can decrease glutamate levels which leads to lethargy and reduced nervous system activity. The most common urea- cycle defect is OTC deficiency, which is an X-linked disorder. Amino Acid Metabolism https://www.youtube.com/watch?v=0M-B2dOfcUo Metabolism | Urea Cycle https://www.youtube.com/watch?v=XZEJ5PNvjkk References Lieberman, M. A., Peet, A. (2018). Mark’s Basic Medical Biochemistry. A Clinical Approach (5th ed.). Wolters Kluwer: Philadelphia, PA. NMDP 734 Biochemistry II: Amino Acid Metabolism Dr. Carla Ortiz, ND Naturopathic Medicine Program Source: Hinz, Marty & Stein, Alvin & Cole, Ted. (2014). Parkinson's disease-associated melanin steal. Neuropsychiatric disease and treatment. 10. 2331-7. 10.2147/NDT.S74952. Location: Melanocytes Source: Cichorek, Miroslawa & Wachulska, Malgorzata & Skoniecka, Aneta. (2013). Heterogeneity of neural crest- derived melanocytes. Central European Journal of Biology. 8. 315-330. 10.2478/s11535-013-0141-1. Location: Neurons Thyroid-hormone Biosynthesis Thyroglobulin (Tgb) carries tyrosine (Tyr) amino acids Iodination of Tyr by thyroxine peroxidase (TPO) produces monoiodotyrosine (MIT) and diiodotyrosine (DIT) Coupling by TPO produces T4 (tetraiodothyronine, a.k.a. thyroxine) T3 (triiodothyronine, the most active form) Tgb with the MITs and DITs enter thyroid follicular cells When Tgb is cleaved by a lysosomal protease, the MIT and DIT are deiodinated and the iodine recycled T4 and T3 are released into the blood where they are carried by TBG Tyr and thyroglobulin are recycled https://doi.org/10.3389/fendo.2019.00158 Clinical Note Risk of serotonin syndrome with incorrect tryptophan supplementation: Nitrogen Metabolism: Synthesis and Degradation of Amino Acids Because each of the 20 protein amino acids has a unique structure, their metabolic pathways differ. Some generalities apply to both synthesis and degradation: Only the liver has all the pathways for aa synthesis/degradation. Amino acid metabolism uses, to a large extent, three main cofactors: 1. Pyridoxal phosphate (PLP), derived from vitamin B6, is required for transamination reactions 2. Tetrahydrobiopterin (BH4) is required for ring hydroxylation reactions 3. Tetrahydrofolate (FH4), derived from folate, is required to either accept or donate a one-carbon group Amino Acid Metabolism: Synthesis Pathways The non-essential amino acids can be synthesized from: Glycolytic intermediates (serine, glycine, cysteine*, and alanine) TCA-cycle intermediates (aspartate, asparagine, glutamate, glutamine, proline, arginine, and ornithine) Existing amino acids (tyrosine and cysteine*) tyrosine from phenylalanine * cysteine from methionine + serine Amino Acid Metabolism: Degradation Pathways In the liver during fasting, aa degradation produces: glucose (via gluconeogenesis) Glucogenic – alanine, serine, glycine, cysteine, aspartate, asparagine, glutamate, glutamine, proline, arginine, histidine, methionine, valine, isoleucine, tryptophan, threonine, tyrosine, phenylalanine ketone bodies (via ketogenesis) Ketogenic – lysine, leucine, isoleucine, tryptophan, threonine, tyrosine, phenylalanine CO2 (via oxidative phosphorylation) Which aa are both glucogenic and ketogenic? In the fed state, the liver can convert intermediates of aa metabolism to glycogen and triacylglycerols (source: excess glucose) Which aa are strictly ketogenic? Main degradation pathway of proline. Ascorbate acts as a reducing agent in the conversion of proline to hydroxyproline, which is required for collagen formation. Image from: http://web.campbell.edu/faculty/nemecz/323_lect/proteins/prot_chapter.html Clinical Note Note: Lysine and leucine are the only purely ketogenic amino acids, as they are degraded into the precursors for ketone body synthesis, acetyl-CoA and acetoacetate. Clinical Note Clinical Note Clinical Note Clinical Note Cystinuria is caused by a defect in the transport protein that carries cystine, lysine, arginine, and ornithine into enterocytes and that permits resorption of these aa by renal tubular cells. Cystine (two cysteines bound through a disulfide bond), which is not very soluble in the urine, forms renal calculi (stones). Clinical Note Cystinosis is a rare disorder caused by a defective carrier that normally transports cystine across the lysosomal membrane from lysosomal vesicles to the cytosol. Cystine accumulates in the lysosomes in many tissues and forms crystals, leading to a depletion of intracellular cysteine levels. Children with this disorder develop renal failure by 6 to 12 years of age, through a mechanism that has not yet been fully elucidated. Clinical Note References Lieberman, M. A., Peet, A. (2018). Mark’s Basic Medical Biochemistry. A Clinical Approach (5th ed.). Wolters Kluwer: Philadelphia, PA.