Cellular Biology for Engineers PDF
Document Details
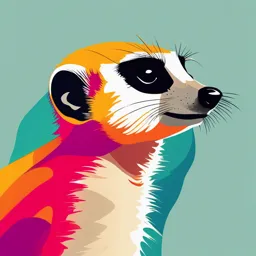
Uploaded by CoolestMarimba
Deakin University
2008
K. Khoshmanesh, A.Z. Kouzani, S. Nahavandi, S. Baratchi, J.R. Kanwar
Tags
Summary
This textbook provides an overview of cellular biology for engineers. It explains the structure and function of different cell components using analogies like "micro-plants" forming "micro-bio-factories." The text also demonstrates complex cell functions such as energy generation and protein synthesis through simple flow diagrams, emphasizing the interaction between cell components.
Full Transcript
Computational Biology and Chemistry 32 (2008) 315–331 Contents lists available at ScienceDirect Computational Bi...
Computational Biology and Chemistry 32 (2008) 315–331 Contents lists available at ScienceDirect Computational Biology and Chemistry journal homepage: www.elsevier.com/locate/compbiolchem Review At a glance: Cellular biology for engineers K. Khoshmanesh a,∗ , A.Z. Kouzani a , S. Nahavandi a , S. Baratchi b , J.R. Kanwar b a School of Engineering and IT, Deakin University, Geelong VIC 3217, Australia b Institute of Biotechnology (BioDeakin), Deakin University, Geelong VIC 3217, Australia a r t i c l e i n f o a b s t r a c t Article history: Engineering contributions have played an important role in the rise and evolution of cellular biology. Received 14 March 2008 Engineering technologies have helped biologists to explore the living organisms at cellular and molecular Accepted 6 July 2008 levels, and have created new opportunities to tackle the unsolved biological problems. There is now a growing demand to further expand the role of engineering in cellular biology research. For an engineer Keywords: to play an effective role in cellular biology, the first essential step is to understand the cells and their Cellular biology components. However, the stumbling block of this step is to comprehend the information given in the Cell organelles cellular biology literature because it best suits the readers with a biological background. This paper aims Micro-bio-factory Energy generation to overcome this bottleneck by describing the human cell components as micro-plants that form cells as Protein synthesis micro-bio-factories. This concept can accelerate the engineers’ comprehension of the subject. In this paper, first the structure and function of different cell components are described. In addition, the engineering attempts to mimic various cell components through numerical modelling or physical implementation are highlighted. Next, the interaction of different cell components that facilitate complicated chemical processes, such as energy generation and protein synthesis, are described. These complex interactions are translated into simple flow diagrams, generally used by engineers to represent multi-component processes. © 2008 Elsevier Ltd. All rights reserved. Contents 1. Introduction......................................................................................................................................... 316 2. The Classification of Microorganisms............................................................................................................... 316 3. Contribution of Engineering to the Evolution of Cellular Biology.................................................................................. 317 4. Description of Human Cell Organelles.............................................................................................................. 318 4.1. Cell Membrane............................................................................................................................... 319 4.2. Cytoplasm................................................................................................................................... 320 4.3. Endoplasmic Reticulum (ER)................................................................................................................ 321 4.4. Golgi Body................................................................................................................................... 322 4.5. Lysosomes................................................................................................................................... 322 4.6. Centrosome.................................................................................................................................. 323 4.7. Mitochondria................................................................................................................................ 323 4.8. Nucleus...................................................................................................................................... 324 4.8.1. Nuclear Envelope................................................................................................................... 324 4.8.2. Nucleoplasm....................................................................................................................... 324 4.8.3. Chromatin......................................................................................................................... 324 4.8.4. Nucleolus.......................................................................................................................... 325 4.9. Ribosome.................................................................................................................................... 325 ∗ Corresponding author. Tel.: +61 3 52272208; fax: +61 3 52272167. E-mail address: [email protected] (K. Khoshmanesh). 1476-9271/$ – see front matter © 2008 Elsevier Ltd. All rights reserved. doi:10.1016/j.compbiolchem.2008.07.010 316 K. Khoshmanesh et al. / Computational Biology and Chemistry 32 (2008) 315–331 5. Description of Cells’ Major Chemical Processes.................................................................................................... 325 5.1. Energy Generation........................................................................................................................... 325 5.2. Protein Synthesis............................................................................................................................ 327 6. Conclusion.......................................................................................................................................... 329 References.......................................................................................................................................... 329 1. Introduction 2. The Classification of Microorganisms In recent years, the fusion of engineering and biology has created Most engineers have heard about the terms such as virus, bac- innovative areas such as systems biology, synthetic biology, and teria, human cell and plant cell but do not know about their biomedical engineering (Andrianantoandr et al., 2006; Brent, 2004; biological classification and main specifications. In this section, Westerhoff and Palsson, 2004), and also promising technologies the basic elements of the biological organisms are classified and such as DNA microarrays, biosensors, and lab-on-a-chips (Alizadeh briefly described. Those elements are known as microorganisms as et al., 2000; Buerk, 1993; Weigl et al., 2003). These areas and tech- they are too small to be seen by human eye. The microorganisms nologies have enabled biologists to explore the living organisms are classified into three groups: non-living agents, prokaryotic cell, at cellular and molecular levels, and have opened up new oppor- and Eukaryotic cells as shown in Fig. 1. Some references exclude the tunities to tackle the unsolved biological problems. Advances in non-living agents from the microorganisms; however, it is assumed molecular and cell biology have revolutionised the healthcare, and in this paper that the microorganisms include both living and non- more importantly initiated new exciting interdisciplinary direc- living groups. tions in engineering. In this respect, there is an enormous demand The non-living group refers to the viruses because they are to expand the biology-related technologies and industries. unable to live independently. A virus penetrates a cell and takes Thanks to engineering devices, considerable research progress control of its biological system to flourish and reproduce itself and has been made in different branches of healthcare. For exam- later attack the other cells. The virus structure consists of a genetic ple, a variety of devices and technologies are being developed for material (DNA or RNA) enclosed by a protein coat called capsid. point-of-care detection, diagnostics, prognostics, and treatment of Some viruses have a more complex structure and may be equipped the most complicated diseases such as cancer. In contrast to the with an envelope around the capsid or a tail attached to the capsid. conventional methods of cancer diagnostics and prognostics that Some diseases caused by viruses include, HIV, Hepatitis-B and flu rely on the invasive isolation of cancer tumors and visual inspec- (Becker et al., 2006; Clark, 2005). tion of cell morphology, the emerging technologies facilitate the The prokaryotic cell group refers to bacteria that live as single- non-invasive scanning of tumor responses such as changes in the cell microorganisms. The structure of the bacteria is rather simple activation or inactivation of specific proteins or expression of spe- with a genetic material (DNA) suspended in a jelly-like material cific genes. These approaches realise the profiling of tumors and called cytoplasm. The cytoplasm is equipped with some tiny par- produce their molecular signatures (Liotta and Petricoin, 2000). ticles known as ribosomes that produce the needed proteins. The Most of the new emerging technologies deal with cells as the whole structure is enclosed by the cell envelope that is made up basic structural components of living organisms. For an engineer of two to three layers: a cytoplasmic membrane, a cell wall, and an to play an effective role in these fields, the first essential step is to outer capsule in some species of bacteria. Some bacteria are also understand the basic elements and complexities of cellular biol- equipped with hair-like structures called flagella that provide loco- ogy. However, the main bottleneck in accomplishing this step is motion. The bacteria exist almost everywhere, in soil, water, air to understand the detailed information given in academic cellular and even our bodies. They can adapt themselves with the harshest biology literature. The issue is that the material covered in the liter- environments of the world such as the acidic hot springs, or in the ature best suits the readers with a prior exposure to biology. On the deepest locations of the earth’s crust. Bacteria could be harmless other hand, there exist some resources that describe cellular biol- (non-pathogenic) or harmful (pathogenic). The E. coli bacteria that ogy in layman’s terms. But those resources deal with the subject in a exist in the large intestine of our bodies are normally harmless and manner that does not provide the reader with the necessary details. produce vitamin K. On the other hand, the Bacillus bacteria that The objective of this paper is to bring the basic principles of exist in soil or water are harmful and cause the fatal tuberculosis cellular biology under one umbrella, and explain the structure, (Becker et al., 2006; Clark, 2005). function, and interaction of human cell organelles in a way that The eukaryotic cell group refers to the cells that make up the can benefit engineers interested in biology-related researches. In organs of humans, animals, plants, and fungi. The structure and this paper, the cell is presented from engineering perspective as a function of eukaryotic cells are more complex in comparison to micro-bio-factory with several process plants. The inlets and out- those of prokaryotic cells. The genetic material (DNA) is enclosed lets of each plant as well as the plant’s role are discussed through within a membrane called cell nucleus. Eukaryotic cells also have a number of flow diagrams. other specialised structures suspended in the cytoplasm. The main The paper is organised as follows. Section 2 presents the classifi- structures are endoplasmic reticulum, Golgi body, Lysosomes, cen- cation of the microorganisms to identify the position of the human trosome, mitochondria, and ribosome. These are discussed in the cell among other cells. Section 3 gives the discovery timeline of following sections of this paper. The whole structure is protected the human cell organelles emphasising on the role of engineering by the cell membrane (Becker et al., 2006; Clark, 2005). instruments and interdisciplinary research. Section 4 describes the The cells of humans and animals are basically the same. How- following organelles of the human cell: cell membrane, cytoplasm, ever, the plant cells differ from the human/animal cells because: endoplasmic reticulum, Golgi body, Lysosomes, centrosome, (i) the membrane of the plant cells is covered with a cellulose wall mitochondria, nucleus and ribosome. Section 5 explains two major that stiffens the cell’s structure and allows the plant to grow tall, (ii) chemical processes occurring in the cell: energy generation and most of the space within the plant’s cell is occupied by a large vac- protein synthesis. Finally, concluding remarks are given in Section uole that stores water, ions and toxins, and (iii) plant cells contain 6. chloroplast that is a pigment which determines the colour of the K. Khoshmanesh et al. / Computational Biology and Chemistry 32 (2008) 315–331 317 Fig. 1. Classification of microorganisms. plant, and eases the conversion of carbon dioxide and water into gists T. Schwann and M. Schleiden. The cell theory states that all glucose and oxygen by absorbing the sunlight (Becker et al., 2006; organisms are composed of similar units of elements called cells. Clark, 2005). The idea looks simple today, but it established the foundation of Fungi are more likely to be plants however the fungi cells differ modern biology (Mazzarello, 1999). The emergence of cell theory from the plant cells because: (i) the fungi cells lack chloroplast and and the invention of more advanced compound microscopes led therefore absorbing food from their surrounding, and (ii) the cell the biologists to discover five cell organelles: cell nucleus, mito- wall of the fungi cells is made of a nitrogenous substance known as chondria, centrosome, cell membrane, and Golgi body during the chitin. Yeast and mold are among the most famous fungi (Becker et years 1833–1897. al., 2006; Clark, 2005). The invention of electron microscopes by German engineers As the microorganisms become more complex, their size and E. Ruska and M. Knoll in 1931 pushed forward the role of micro- the number of their ingredient genes increase. A virus is typically scopes in cellular biology. Electron microscopes were developed 0.01–0.1 m across and has around 200 genes, a bacterium is typ- due to the fact that the magnification of light microscopes was ically 1–10 m across and has around 4000 genes, and a human limited to 1000×. The electron microscope uses a beam of highly cell is typically 10–100 m across and poses 25,000 genes (Clark, energetic electrons to illuminate and create an image of a sam- 2005). ple that can provide magnifications of up to 2,000,000× (Ruska, 1986). Taking advantage of electron microscopes, biologists dis- covered two more cell organelles in 1945: endoplasmic reticulum 3. Contribution of Engineering to the Evolution of Cellular and microtubules. They could also identify the traces of ribosomes Biology on the rough endoplasmic reticulum and inside the cytoplasm by 1958. Cellular biology deals with the cells as the basic elements of The introduction of X-ray imaging by German physicist W. the living organisms. Cellular biology could be called modern biol- Röntgen in 1895 advanced the boundaries of the modern biology. ogy as it is younger that the traditional biology which focuses on Basically X-rays are a type of electromagnetic radiation with wave- the tissues as the structural elements of living organisms. Due lengths of around 10−10 m (Gray and Orton, 2000). Although the to its characteristics, the rise and evolution of cellular biology X-ray imaging was vastly used to detect the bone defects, the sci- has been more interwoven with two factors: (i) the invention entists applied this method to take the images of the DNA in 1952. of engineering instruments, and (ii) the application of interdisci- Using those images, Watson and Crick put forward the current plinary approaches. Those two factors have led the biologists to double-helix model of DNA by 1953 (Watson and Crick, 1953). discover the cell organelles, and also to develop cell models for- The development of differential centrifugation technique by mulating the complicated interactions between them as shown in the Belgian biologist A. Claude in the late 1930s helped the biol- Fig. 2. ogists to sort the cell organelles and discover new components. In this section, the evolution of cellular biology is reviewed Using this technique, Claude discovered the ribosome particles in with more emphasis on the role of the invention of engineering 1938 (Claude, 1946). The technique was further utilised by Belgian instruments and the application of interdisciplinary approaches. cytologist and biochemist C.R. Duve to discover the Lysosomes in With regard to the engineering instruments, the contribution 1956. of compound microscopes, electron microscopes, X-ray imag- ing, and differential centrifuge in the growth of cellular biology is reviewed. With respect to interdisciplinary approaches, the role of biochemistry in the advancement of cellular biology is reviewed. The birth of cellular biology was facilitated by the development of microscopes. This is due to the fact that cells are below the limit of resolution of the human eye and can only be visualised by means of proper instruments such as microscopes. The microscope has con- tributed more than any other instrument to the development of biology as a science. In 1590, the first compound microscope was produced by the Dutch spectacle-maker Z. Janssen (Croft, 2006). In 1663, the English scientist R. Hooke, discovered cells in a piece of cork, which he examined under his primitive microscope (Ash, 1998). The value of this discovery was not appreciated until the Fig. 2. The evolution of cellular biology was facilitated by engineering instruments 1839, when the cell theory was introduced by German physiolo- and taking interdisciplinary approaches. 318 K. Khoshmanesh et al. / Computational Biology and Chemistry 32 (2008) 315–331 Table 1 The timeline for the first wave of cellular biology evolution with respect to the invention of tools and techniques and the development of cell models Invention of tools and Discovery of cell organelles Development of cell models techniques Compound microscope invented by Janssen (1590) Cell discovered by Hooke (1633) The biochemistry was pioneered by Wöhler (1828) Cell nucleus reported by Brown (1833) Cell theory proposed by Schwann and Schleiden (1839) Mitochondria discovered by Kölliker (1857) DNA isolated by Miescher (1869) The term ‘centrosome’ used by Boveri (1888) X-ray imaging invented by Cell membrane distinguished by Overton Röntgen (1895) (1895) Golgi body discovered by Golgi (1897) Electron microscope built by Ruska and Knoll (1931) Differential centrifugation Krebs cycle of mitochondria identified by Krebs (1937) technique was developed by Claude (1930s) Cytoplasm discussed by Just (1938) Ribosome discovered by Claude (1938) The role of RNA in protein synthesis discovered by Caspersson, Brachet and Schultz (1939) Endoplasmic reticulum and microtubules identified by Porter, Claude and Fullam (1945) The role of ribosome in protein synthesis put forwarded by Claude (1946) The current model of DNA structure suggested by Watson and Crick, based on the X-ray images taken by Franklin (1953) Lysosomes discovered by Duvé (1956) The location of ribosomes in endoplasmic reticulum and cytoplasm noticed by Palade (1958) Mitochondria ATP synthesis proposed by Mitchell (1961) The fluid mosaic model of cell membrane proposed by Singer and Nicolson (1972) The biochemistry was one of the first interdisciplinary (identification of all human genes and their sequences) by 2003 approaches to discover the world of cells. The biochemistry was pio- (Liotta and Petricoin, 2000). neered by the German chemist F. Wöhler, who published a paper Mapping the complete human genome led the biologists to on the synthesis of urea in 1828 (Morrison and Weiss, 2006). It evolve the third wave of innovations that is known as genomics. was further developed with the discovery of the first enzyme in Whilst the genetics deals with the single gene to understand its 1833. The evolution of biochemistry led the biologists to isolate the function and response to external factors, the genomics deals genetic material (DNA) in 1869 (Dahm, 2008). More experiments with the all genes to understand their interaction and combined helped the biologists to uncover two enigmatic processes that take response to external factors (Liotta and Petricoin, 2000). place in the cell: protein synthesis and energy production. The role The fourth wave of innovations targeted the proteins and of genetic information carriers (RNA) and ribosomes in protein syn- therefore is called proteomics. The DNA can be regarded as the thesis was uncovered in a series of experiments by 1946. In about information and instruction database of the cells while the pro- the same period, the mitochondrial Krebs cycle and the electron teins can be considered as the task forces or agents of the cell. The chain transport were discovered to reveal the energy production proteins are produced from the genetic instructions stored in DNA secret of mitochondria by 1961 (Scheffler, 2000). and then sent throughout the cell performing specific functions Table 1 summarises the evolution of cellular biology from the through biochemical reactions. Formulating the behaviour of the invention of microscope through the introduction of the current proteins is much more complicated than that of the genes. This is model of cell membrane. because the genes are stable and common amongst all cells of liv- The table does not cover the advancements beyond 1972. The ing organisms, while the proteins are variable in response to the main reason is that the first wave of the innovations in the cellu- conditions influencing the cell (Liotta and Petricoin, 2000). lar biology that tended to the discovery of major cell organelles and the development of cell models was completed by that 4. Description of Human Cell Organelles time. After that milestone, most of the conducted research was This section presents the structure and function of human focused on exploring the behaviour of DNA. This second wave cell organelles including: cell membrane, cytoplasm, endoplas- of innovations is known as molecular genetics because it deals mic reticulum, Golgi body, Lysosomes, centrosome, mitochondria, with genes as the basic elements of DNA. Molecular genetics was nucleus and ribosome. These organelles act as the structural and flourished by developing DNA sequencing (determination of gene functional elements of the cell, facilitating the activities of differ- sequence along DNA polymer) and DNA amplification (produc- ent human tissues and organs. Fig. 3 depicts the physical shape ing multiple copies of specific genes) methods. Invention of DNA and location of the cell organelles as perceived by biologists. On microarrays that analyse several thousand genes at the same time the other hand, Fig. 4 depicts the cell organelles as perceived by enabled the scientists to accomplish the human genome project engineers. Using appropriate symbols, the cell organelles are repre- K. Khoshmanesh et al. / Computational Biology and Chemistry 32 (2008) 315–331 319 Fig. 3. The configuration of different human cell organelles. sented as micro-process plants that form the cell as a micro-factory. in Fig. 5. The phospholipids are made of a phosphate head and a The figure can help engineer readers to easier comprehend the pair of fatty-acid (lipid) tails. The phosphate head is polar while description of the cell organelles presented below. the lipid tail is non-polar. The polar molecules are water-soluble and often called hydrophilic or ‘water-loving’ molecules. In con- 4.1. Cell Membrane trast, the non-polar molecules are water-insoluble and often called hydrophobic or ‘water-fearing’ molecules. The interior and exterior The cell membrane is a dynamic structure that surrounds the cell environments of the cell contain a lot of water, which directs the and isolates it from the exterior environment. The cell membrane polar phosphate heads outward and the non-polar lipid tails inward is composed of two layers of phospholipid molecules as shown (Alberts et al., 2008; Becker et al., 2006; Karp, 2007; Lodish et al., Fig. 4. The cell organelles are shown as the micro-plants of a micro-bio-factory. The cell membrane is shown as the wall of the factory with some gates to import and export the goods. The cytoplasm is shown as the interior of the factory. The rough endoplasmic reticulum, smooth endoplasmic reticulum and Golgi body are shown as process plants. The nucleolus, ribosome and centrosome are shown as assembly lines. The vesicles and lysosome are shown as trucks that transport the goods. The chromatin that holds the DNA is shown as the database of the factory. The mitochondria is shown as the power station of the factory. 320 K. Khoshmanesh et al. / Computational Biology and Chemistry 32 (2008) 315–331 the hormones circulating within the blood. The hormones act as information signals within the body and trigger correspond- ing reactions in the cell. For example, the metabolic hormones that control the digestion and storage of food may trigger the corresponding cells to secret digestive enzymes (Alberts et al., 2008; Becker et al., 2006; Karp, 2007; Lodish et al., 2008; Tobin and Morel, 1997; Voet et al., 2006; Walker and Rapley, 2000). vi. The membrane is equipped with cholesterol molecules. The cholesterol stabilises the fluidity of membrane against tempera- ture variations. At high temperatures, cholesterol decreases the movement of the lipids, making the membrane less fluid and reducing its permeability. At low temperatures, on the other hand, cholesterol increases the movement of the lipids, main- Fig. 5. Structure and different components of the cell membrane. tains its fluidity preventing membrane from freezing (Alberts et al., 2008; Becker et al., 2006; Karp, 2007; Lodish et al., 2008; 2008; Tobin and Morel, 1997; Voet et al., 2006; Walker and Rapley, Tobin and Morel, 1997; Voet et al., 2006; Walker and Rapley, 2000). Several models have been proposed to explain and formu- 2000). More research is conducted to address the remained late the cell membrane structure. The basic fluid mosaic model questions about the structure, dynamic behaviour and functions of the cell membrane is discussed in Singer and Nicolson (1972) of the cell membrane as discussed in Dopico and Tigyi (2007), while the evolution of the proposed models is reviewed by Edidin Fielding (2007), Heimburg (2007), Kusumi et al. (2005), Marguet (2003). The main functions and features of the cell membrane can et al. (2006), Ritchie et al. (2005), Weber et al. (2006) and Wu et be summarised as follows: al. (2004). i. The membrane separates the cell organelles from the exterior The engineering aspects of the cell membrane have been studied environment and also acts as a selectively preamble membrane. in different works. The elastic deformation of the cell membrane Small molecules of water, oxygen, and carbon dioxide can pass is modelled in Du et al. (2005). The selective permeability of the through the membrane, while the big molecules of glucose and cell membrane is taken as a template to synthesise ion-selective amino acids cannot do so. For the big molecules, some other supramolecular polymers (Percec and Bera, 2002). Based on the mechanisms have been predicted according to their polarities. bilayer structure of the cell membrane, cylindrical nanofibers These mechanisms are described in ii and iii (Alberts et al., 2008; are synthesised to facilitate the self-assembly of proteins within Becker et al., 2006; Karp, 2007; Lodish et al., 2008; Tobin and the damaged tissues. This innovation leads to the development Morel, 1997; Voet et al., 2006; Walker and Rapley, 2000). of regenerative medicines as discussed in Guler et al. (2006), ii. For the big non-polar molecules such as the nutrition particles Hartgerink et al. (2002) and Stupp et al. (1997). Nanoparticles with or cell waste products, the membrane applies the endocyto- biodegradable shells are developed to penetrate the cell membrane sis and exocytosis mechanisms. In the endocytosis mechanism according to the endocytosis mechanism and deliver drugs to the that is applied to incoming nutrition particles, the particles are organelles. This technique can revolutionise the therapeutic and wrapped within a lipid cover called the vesicle and then pushed diagnostic phases of enigmatic diseases such as cancer (Maysinger, through it. On the other hand, in the exocytosis mechanism that 2007; Vasir and Labhasetwar, 2007; L. Zhang et al., 2007; B. Zhang is applied to outgoing waste products, the vesicle surrounding et al., 2007). the particles is fused with membrane and then pushed through it (Alberts et al., 2008; Becker et al., 2006; Karp, 2007; Lodish et 4.2. Cytoplasm al., 2008; Tobin and Morel, 1997; Voet et al., 2006; Walker and Rapley, 2000). The nucleus of all eukaryotic cells is surrounded by cytoplasm. iii. For the big polar molecules such as glucose, the membrane is The cytoplasm is a jelly-like material that holds together the equipped with some transport proteins interspersed within the cell organelles. The area of the cytoplasm outside the individual phospholipid layers. The transport proteins have a flexible struc- organelles is called cytosol. The cytosol is made of mostly water. It ture and are reshaped in response to the particle’s shape to grab also contains some proteins, enzymes, carbohydrates, salts, sugars, and swallow it (Alberts et al., 2008; Becker et al., 2006; Karp, lipids, nucleotides, and amino acids (Alberts et al., 2008; Becker et 2007; Lodish et al., 2008; Tobin and Morel, 1997; Voet et al., al., 2006; Karp, 2007; Lodish et al., 2008; Tobin and Morel, 1997; 2006; Walker and Rapley, 2000). Voet et al., 2006; Walker and Rapley, 2000). The main functions of iv. The movement of the particles through the membrane is driven the cytoplasm can be summarised as follows: by the concentration gradient over the two sides of the mem- brane that is known as diffusion mechanism. However, if the i. The molecules that enter the cell membrane should pass through particles should travel against the concentration gradient, some the cytosol before entering their final destinations. Therefore, energy is consumed to pump them in or out (Alberts et al., 2008; the cytosol acts as a medium between the cell membrane and Becker et al., 2006; Karp, 2007; Lodish et al., 2008; Tobin and different cell organelles. In this sense, it may act as a primary Morel, 1997; Voet et al., 2006; Walker and Rapley, 2000). reactor to undertake some chemical processes on the passing v. Apart from the transport proteins, the membrane also takes molecules. For example, the cytosol takes the incoming 6-carbon advantage of marker proteins and receptor proteins. The marker glucose molecules and split them into two 3-carbon molecules proteins or glycoproteins are made of protein and carbohydrate in a process called the glycolysis (Alberts et al., 2008; Becker et elements. The protein end is anchored in the membrane while al., 2006; Karp, 2007; Lodish et al., 2008; Tobin and Morel, 1997; the carbohydrate end lays on the external surface of the mem- Voet et al., 2006; Walker and Rapley, 2000). The reaction of the brane to help the immune system identifying the cell as a friend. cytoplasm after the invasion of infectious viruses or bacteria is Similarly, the receptor proteins lay on the external surface of discussed in Yoneyama and Fujita (2007). The cytosol acts as a the membrane. The receptor protein is responsible to receive big reservoir for the materials needed for different processes. K. Khoshmanesh et al. / Computational Biology and Chemistry 32 (2008) 315–331 321 For example, in order to produce a protein molecule, the amino acids that are submerged in the cytosol are collected and laid in a special arrangement in a process called the protein synthe- sis (Alberts et al., 2008; Becker et al., 2006; Karp, 2007; Lodish et al., 2008; Tobin and Morel, 1997; Voet et al., 2006; Walker and Rapley, 2000). The behaviour and associated mechanisms of cytoplasm during the cancer development can be found in Germain and Frank (2007), Hanahan and Weinberg (2000) and Ifergan et al. (2005). ii. The cytosol contains a skeletal structure, called the cytoskele- ton. The cytoskeleton is a dynamic three-dimensional structure of protein fibres. The cytoskeleton has three major elements: microfilaments, microtubules, and intermediate filaments. The microfilaments are thin filaments of protein accumulated under the cell membrane. They stiffen the cell structure and facilitate Fig. 6. The structure of the endoplasmic reticulum. tiny cellular movements including gliding and contraction. On the other hand, the microtubules are hollow tubes consisting of several protein filaments distributed under the microfilaments. ii. The endoplasmic reticulum (ER) is equipped with chaperones The microtubules act as a scaffold to determine the cell shape and folding enzymes that facilitate the folding of protein chains. and divide the organelles. They also provide a set of tracks for The chaperones control the quality of the folded proteins to the movement of organelles and vesicles within the cell. Finally, ensure that only the correctly folded proteins are exported from the intermediate filaments that are made of several protein the ER. The misfolded proteins are separated and later degraded. filaments coil around each other to form a rope. The name ‘inter- The amount of proteins that are processed by the ER is set mediate’ comes from the fact that the diameter of these elements by specific signalling pathways and effector mechanisms. The is bigger than the diameter of microfilaments and smaller than upstream signal that activates these pathways is called ER stress the diameter of microtubules. The intermediate filaments pro- and indicates the difference between the amount of the pro- vide tensile strength for the cell and help it withstand against duced protein chains and the ER capacity (Alberts et al., 2008; the mechanical stresses (Alberts et al., 2008; Becker et al., 2006; Becker et al., 2006; Karp, 2007; Lodish et al., 2008; Tobin and Karp, 2007; Lodish et al., 2008; Tobin and Morel, 1997; Voet et Morel, 1997; Voet et al., 2006; Walker and Rapley, 2000). More al., 2006; Walker and Rapley, 2000). details about the ER functions including signalling pathways, The composite structure of the cytoskeletons and cytosol has folding and quality control of proteins are discussed in Hollien been of great interest to engineering researchers. The mechani- and Weissman (2006), Kleizen and Braakman (2004), Marciniak cal characteristics of the cytoplasm are comprehensively reviewed and Ron (2006) and Ron (2002). The disruption of the ER during in Alt et al. (1997) and Zhu et al. (2000). The elastic deformations the cancer development that leads to the misfolding of proteins and the propagation of mechanical stresses through the cytoplasm is discussed in Carracedo et al. (2006), Teiten et al. (2003) and are analysed in Gladilin et al. (2007), Hu et al. (2005) and Tracqui Zong et al. (2003). and Ohayon (2004). The hydrodynamic analysis of the cytoplasm is iii. The space enclosed by the RER membrane is called lumen. The given in Alt and Dembo (1999) and Yoneda and Nagai (1988). proteins travel through the lumen to enter the SER. The RER offers energy-efficient pathways for the transportation and dif- 4.3. Endoplasmic Reticulum (ER) fusion of proteins (Alberts et al., 2008; Becker et al., 2006; Karp, 2007; Lodish et al., 2008; Tobin and Morel, 1997; Voet et al., The term ‘endo’ means within and the term ‘reticulum’ means 2006; Walker and Rapley, 2000). Recent studies introduce the network, thus the endoplasmic reticulum (ER) means a network ER network of neurons as the conveyor and integrator of signals within the cytoplasm. There are two types of ER within the cell: that activate the dendrites (Park et al., 2008). rough endoplasmic reticulum (RER) and smooth endoplasmic retic- ulum (SER) (Alberts et al., 2008; Becker et al., 2006; Karp, 2007; The SER is the extension of RER network. The main structural Lodish et al., 2008; Tobin and Morel, 1997; Voet et al., 2006; Walker features of the SER include its network of tubular-like sacs, and also and Rapley, 2000). the lack of ribosomes on its membrane surfaces as shown in Fig. 6. With regard to the RER, the membrane of the cell nucleus is Due to those specifications the functionality of the SER differs from extended at some spots. At those points, the extended membrane the RER as described below: forms a series of flattened sacs or sheet-like structures that are called RER. The sacs are interconnected and spread throughout the cell as shown in Fig. 6. They provide enough extended surface to i. Once the protein leaves the RER, it is sent to the SER to be pack- interact with the cytoplasm. Due to the specific characteristics of aged via vesicle and released within the cytoplasm (Alberts et RER, its main functions can be summarised as follows: al., 2008; Karp, 2007; Lodish et al., 2008; Tobin and Morel, 1997; Voet et al., 2006; Walker and Rapley, 2000). i. The RER plays a vital role in the process of protein production. ii. The SER is not involved in protein production, but its large Some of the ribosomes employ the extended surface of RER extended surface facilitates other chemical reactions in the as their unloading docks to discharge their produced proteins. cytoplasm. Some of the major chemical reactions that are Thousands of ribosomes might bind to the RER at the same time, realised by the SER include: (a) the synthesis of phosolipids therefore its surface looks bumpy or so called ‘rough’ under the that is the essential part of cell membrane, (b) the metabolism microscope. The other key players of protein synthesis process of cholesterol that is a part of cell membrane and also plays a are introduced in Section 5.2 (Alberts et al., 2008; Becker et al., key role in the regulation of some hormones and digestion of 2006; Karp, 2007; Lodish et al., 2008; Tobin and Morel, 1997; fats, and (c) the breakdown of glycogen, that is the stored form Voet et al., 2006; Walker and Rapley, 2000). of glucose, to release energy during the peak times (Lodish et 322 K. Khoshmanesh et al. / Computational Biology and Chemistry 32 (2008) 315–331 ii. The lysosomal protein that serves as the degrading enzyme within the cell is modified by adding phosphate links to the protein. Its function is described in Section 4.5 (Alberts et al., 2008; Becker et al., 2006; Karp, 2007; Lodish et al., 2008; Tobin and Morel, 1997; Voet et al., 2006; Walker and Rapley, 2000). iii. The ceramide that belongs to the lipid family is synthesised by the Golgi body. The ceramides, phospholipids, and choles- terols are the three lipids that are produced within the ER. The Golgi enzymes add carbohydrate links to the cearmides to make glycolipids. Alternatively, they add phosphate links to the cearmides to make sphingomyelin. Both of the glycolipids and sphingomyelins are further incorporated in the cell membrane (Becker et al., 2006; Karp, 2007; Lodish et al., 2008; Tobin and Morel, 1997; Voet et al., 2006; Walker and Rapley, 2000). Fig. 7. The structure and different components of Golgi body. The proteins and lipids then enter the trans-Golgi, where they are sorted and packaged within different vesicles as follows: al., 2008; Tobin and Morel, 1997; Voet et al., 2006; Walker and i. The lysosome vesicles carry the lysosomal proteins and remain Rapley, 2000). within the cytosol. Their function is described in Section 4.5 iii. The SER detoxifies the drugs and chemical agents by adding OH (Alberts et al., 2008; Karp, 2007; Lodish et al., 2008; Tobin and groups to them so that they can be dissolved and flushed out Morel, 1997; Voet et al., 2006; Walker and Rapley, 2000). of the body easier (Becker et al., 2006; Karp, 2007; Lodish et ii. The membrane vesicles carry the proteins and lipids that are al., 2008; Tobin and Morel, 1997; Voet et al., 2006; Walker and applied in the structure of the cell membrane. The proteins such Rapley, 2000). as transport, marker, and receptor proteins as well as the lipids such as cholesterol, glycolipid, and sphingomyelin use this path- The SER provides necessary space for the storage of the synthe- way to reach the cell membrane. The phospholipid that is the sised materials and minerals such as calcium ions that are needed to main structural element of the cell membrane is provided by the trigger muscle contractions (Alberts et al., 2008; Karp, 2007; Lodish vesicles as they fuse with the membrane (Becker et al., 2006; et al., 2008; Tobin and Morel, 1997; Voet et al., 2006; Walker and Karp, 2007; Lodish et al., 2008; Tobin and Morel, 1997; Voet et Rapley, 2000). al., 2006; Walker and Rapley, 2000). iii. The secretory vesicles carry the proteins and lipids that are 4.4. Golgi Body secreted to the extracellular environment. These vesicles are divided into constitutive secretion and regulated secretion The Golgi body, also known as Golgi apparatus or Golgi complex, groups. The constitutive secretion vesicles move toward the is an arc-shaped organelle that is found nearby the SER. It consists cell membrane and secret their contents as soon as they leave of five to eight flattened sacs that are covered by lipid membranes the trans-Golgi. For example, the liver vesicles that contain the as shown in Fig. 7. The Golgi sacs can be divided into three regions serum albumin proteins continuously secret their proteins into according to their roles: cis-Golgi, medial-Golgi, and trans-Golgi. the blood vessels. On the other hand, the regulated vesicles are The cis-Golgi is oriented toward the ER and is responsible for receiv- stored in the cytosol and are activated when are required. For ing the vesicles that are released by the ER. These vesicles carry the example, the pancreas vesicles that contain digestive enzymes proteins and lipids that are synthesised within the RER and SER. are secreted only after the stomach is filled with food (Alberts et The vesicles fuse with the cis-Golgi membrane, and their contents al., 2008; Karp, 2007; Tobin and Morel, 1997; Voet et al., 2006; are consequently released in the sacs (Becker et al., 2006; Farquhar Walker and Rapley, 2000). The role of the Golgi body in the and Palade, 1981; Karp, 2007; Lodish et al., 2008; Tobin and Morel, development of the Alzheimer disease is explained in Dal Canto 1997; Voet et al., 2006; Walker and Rapley, 2000). The formation (1996) and Hu et al. (2007). and assembly of the Golgi body as well as the hypothesis around the dynamic behaviour of its structural proteins are discussed in Barr 4.5. Lysosomes (2002), Jiang et al. (2006) and Puri and Linstedt (2003). The proteins and lipids then enter the medial-Golgi, where they are processed The lysosomes are small spherical vesicles immersed in the cyto- and modified. To do so, the medial-Golgi is equipped with differ- plasm. The vesicles contain some digestive enzymes known as acid ent enzymes that add carbohydrates or phosphate supplementaries hydrolysis. The enzymes have a normal pH level of 4.8, which makes to incoming molecules. Some recent works on the structural and them effective for selectively dissolving the macromolecules into functional characteristics of the Golgi body as well as the trans- several parts. In this sense, the lysosomes act as the cell garbage portation of proteins are given in Berger and Roth (1997), Cao et al. disposal system. The acidic enzymes are produced in the SER and (2000), Cosson et al. (2005) and Hermo and Smith (1998). The main packaged within the vesicles in the Golgi body. As a safety matter, functions of the Golgi body can be summarised as follows: when the membrane of lysosome is damaged, the acidic enzymes are disabled (Alberts et al., 2008; Becker et al., 2006; Karp, 2007; i. The glycoprotein that serves as the marker protein of the cell Lodish et al., 2008; Tobin and Morel, 1997; Voet et al., 2006; Walker membrane is modified while passing through the Golgi body. and Rapley, 2000). The functions of the lysosomes include the fol- The glycoprotein is produced within the ER by adding a car- lowing: bohydrate link to the protein. The Golgi enzymes modify the carbohydrate link by adding and removing some sugar com- i. They digest the defective organelles such as mitochondria in a pounds (Karp, 2007; Lodish et al., 2008; Tobin and Morel, 1997; process called autophagy. For this, a section of the RER wraps Voet et al., 2006; Walker and Rapley, 2000). around the old organelle as a big vesicle. The lysosomes fuse K. Khoshmanesh et al. / Computational Biology and Chemistry 32 (2008) 315–331 323 Fig. 9. The structure and different components of the mitochondria. Fig. 8. The structure of the centrosome. sides of the nucleus. Then, the microtubules that surround each centrosome are configured as a tail toward the nucleus. Next, with the formed vesicle, releasing their digestive enzymes to the overlapping tails from the opposite centrosomes create a degrade the worn out organelle. Thus, the nutrients are recy- bipolar spindle. As the nucleus breaks down, the spindle acts cled to the cytoplasm and later used within the cell (Alberts as a track to divide equal values of chromosomes for each half et al., 2008; Karp, 2007; Kroemer and Jäättelä, 2005; Lodish of the nucleus (Doxsey et al., 2005; Marshall, 2007; Urbani and et al., 2008; Tobin and Morel, 1997; Voet et al., 2006; Walker Stearns, 1999). More progress about the molecular mechanism and Rapley, 2000). The malfunction or absence of specific diges- of centrosome duplication is discussed in Rodrigues-Martins tive enzymes leads to the accumulation of specific substances et al. (2007), Stearns (2001) and Tsou and Stearns (2006). This within the cytoplasm and impairing of the cell. The group of dis- process is simulated mathematically in Mogilner et al. (2006). eases originated from this problem are called lysosomal storage iii. It may also provide some additional functions. For example, diseases (Futerman and van Meer, 2004; Rohrbach and Clarke, some researchers have proposed that each blade of the cen- 2007; Settembre et al., 2008). triole carries a photoreceptor. The light source is only visible ii. They degrade the enemies such as bacteria that enter the cell. to one of the photoreceptors at a time because the other eight The enemy particle is trapped within a vesicle. The lysosomes photoreceptors are blocked by the shadows of their associated attack the vesicle, releasing their acidic enzymes to degrade the blades. Therefore, the centrioles are able to map the direc- particle (Alberts et al., 2008; Becker et al., 2006; Karp, 2007; tion of the light sources. While the first centriole measures the Lodish et al., 2008; Tobin and Morel, 1997; Voet et al., 2006; ‘longitude’ of the source, the second centriole, that is perpen- Walker and Rapley, 2000). dicular to the first one, measures the ‘latitude’ of the source iii. They are involved in the programmed cell death that is essen- (Albrecht-Buehler, 1992). The function of centrosome may be tial for the development of different structures and is known as hampered by several viruses such as HIV-1. Those deficiencies autolysis. To do so, the contents of the lysosomes are deliberately that may lead to genetic instability are discussed in Afonso et al. released to the cytoplasm and the cell digests itself (Becker et (2007). al., 2006; Holt et al., 2006; Luzio et al., 2007). iv. They provide a membrane patch to repair the damaged parts of 4.7. Mitochondria the cell membrane (McNeil and Kirchhausen, 2005). Role of the lysosomes in the development and therapy of cancer are dis- Mitochondria are bean-shaped organelles that immerse in the cussed in Fehrenbacher and Jättelä (2005), Glunde et al. (2003) cytoplasm. They are bounded by a double membrane, each com- and Maclean et al. (2008). posed of two layers of phospholipids and embedded proteins just like the cell membrane. The outer membrane is smooth while the 4.6. Centrosome inner membrane has many folds. The double membranes divide the mitochondria into two parts: intermembrane space and mito- The centrosome consists of two short cylindrical parts that are chondrial matrix as shown in Fig. 9. The intermembrane space oriented perpendicular to each other. Each part is called centriole. A is a narrow part between the two membranes while the mito- centriole is composed of nine blades, each including three intercon- chondrial matrix is a part enclosed by the inner membrane. The nected microtubule polymers as shown in Fig. 8 (Marshall, 2007; folds of the inner membrane increase the contact surface area Urbani and Stearns, 1999). The functions that are performed by the between the two parts enhancing the performance of mitochon- centrosome include: dria. The mitochondria act as the power generator of the cell, burning carbon and oxygen to release energy (Alberts et al., 2008; i. It produces microtubules that are a major part of the cytoskele- Becker et al., 2006; Karp, 2007; Lodish et al., 2008; Pon and ton. It is surrounded by pericentriolar material, in which there Schon, 2007; Tobin and Morel, 1997; Voet et al., 2006; Walker exists a high concentration of tubulin proteins. The dense mate- and Rapley, 2000). This process is later described in Section 5.1. rial increases the chance of proteins to join. The cylindrical Inside the matrix, there are some DNA polymers that are called structure of the centrosome provides a template for the joined mitochondrial DNA (mtDNA). They are responsible for copying proteins to form the microtubule polymers (Doxsey et al., 2005; some of the proteins that are used within the mitochondria. This Marshall, 2007; Urbani and Stearns, 1999). process is later described in Section 5.2. More information about ii. It facilitates the duplication of the nucleus during the cell divi- the mtDNA can be found in Pakendorf and Stoneking (2005) and sion. First, the two centrioles separate from each other, and then Taylor and Turnbull (2005). The role of the mitochondria in the duplicate. Next, the duplicated centrosomes move to opposite development of cancer is discussed in Brandon et al. (2006), 324 K. Khoshmanesh et al. / Computational Biology and Chemistry 32 (2008) 315–331 Fig. 10. The structure and different components of the cell nucleus. Gulbins et al. (2003), Kroemer (2006) and Paul and Mukhopadhyay (2007). 4.8. Nucleus The nucleus is a small organelle that serves as the headquarter of the cell. It is composed of four main parts: nuclear envelope, nucleoplasm, chromatin, and nucleolus as shown in Fig. 10. The Fig. 11. The structure of the DNA. evolution of Nucleolar compartments are discussed in Thirya and Lafontaine (2005). The structure and functions of these parts are discussed in the following. 4.8.3. Chromatin The term ‘chromatin’, which means the coloured substance, 4.8.1. Nuclear Envelope refers to the DNA polymers that are immersed in the nucleoplasm. The nuclear envelope is a membrane that surrounds the nucleus. However, during the cell division, the freely DNA polymers are Similar to the cell membrane, it is composed of two layers of phos- packed forming chromosomes. In this process, the long DNA poly- pholipid molecules. It protects the nucleus contents, including the mers are interwoven by the histone proteins that are found in the DNA, against the damaging chemical reactions that occur in the nucleoplasm. This packing process is necessary to ensure that all cytoplasm. However, since the nucleus is the cell headquarter, it genes are duplicated during the cell division. It resembles the ‘zip- needs to communicate with the cytoplasm. This function is facil- ping’ of the information stored on a computer before being copied itated by the nuclear pores that are distributed on the nuclear to another computer (Alberts et al., 2008; Becker et al., 2006; Karp, envelope. The pores are the protein complexes that control the pas- 2007; Lodish et al., 2008; Tobin and Morel, 1997; Voet et al., 2006; sage of molecules, just like the transport proteins that are found on Walker and Rapley, 2000). More details about the structure and the cell membrane. The pores let the raw materials (proteins, car- specifications of the chromatin are given in Cremer and Cremer bohydrates, and nucleotides), fuel (ATP), and signals (hormones) to (2005), Cremer et al. (2004) and Qumsiyeh (1999). enter the nucleus while letting the products (RNA and ribosomes) As described earlier, the nucleus acts as the headquar- and waste products (ADP) to leave the nucleus (Alberts et al., 2008; ter of the cell. It analyses the situation in the extracellular Becker et al., 2006; Karp, 2007; Lodish et al., 2008; Tobin and Morel, environment through the hormones and then commands the 1997; Voet et al., 2006; Walker and Rapley, 2000). Using confo- cell organelles to take proper actions. The commands are cal microscopy, recently researchers have managed to visulaise the the genetic instructions that are stored along the DNA poly- single nuclear pores (Kubitscheck et al., 1996). More details about mers. In this sense, the DNA acts as the information database the mechanisms associated with the nuclear envelope are given in of the cell. In order to send the commands to individual Stewart (2007) and Taddei (2007). The role of the nuclear enve- organelles, the commands are copied along the shorter polymers lope in human diseases including cancer is discussed in Kau et al. known as RNA. In this sense, the RNA acts as the informa- (2004) and Muchir and Worman (2004). The elastic properties of tion carriers throughout the cell. The enigmatic structure and the nuclear envelope are characterised in Rowat et al. (2005). features of the DNA and the RNA are described briefly as fol- lows. 4.8.2. Nucleoplasm The nucleoplasm is a viscous liquid that fills the nucleus, just 4.8.3.1. DNA. It is a long polymer of nucleotide molecules joined like the cytosol of the cell. It holds together the nucleus contents together as a double-helix structure as shown in Fig. 11. The and also contains the materials that are vital for nucleus functions. nucleotides are among the four basic units that are received by These materials include the nucleotides and enzymes that are used the cell to facilitate its functions. Each nucleotide molecule is com- to copy the genetic instructions from the DNA polymers, and the posed of three parts: a phosphate compound, a sugar compound, histone proteins that are used to fasten together the DNA polymers and a nitrogen compound known as the base. There are four types during the cell division. The nucleoplasm also contains a network of bases found in the DNA that are: adenine ‘A’, cytosine ‘C’, gua- of fibres known as nuclear matrix to maintain its rigidity. nine ‘G’, and thymine ‘T’. In fact, the nucleotides are distinguished K. Khoshmanesh et al. / Computational Biology and Chemistry 32 (2008) 315–331 325 through their bases. In order to create a DNA polymer, the sugar and DNA. The nucleolus takes the rRNA as well as the proteins that are phosphate compounds of nearby nucleotides attach to each other immersed in the nucleoplasm to make ribosomes. The ribosomes to form a single strand. The bases that are arranged along the single then leave the nucleus through the nuclear pores (recent researches strand then recall their pairs to form the second strand. The ‘A’ bases suggest that the nucleolus has some non-conventional functions pair with the ‘T’ bases while the ‘C’ bases pair with the ‘G’ bases. The such as the assembly of various kinds of ribonucleoprotein parti- two attached strands then are twisted around each other to form a cles, the modification of small RNAs, the control of the cell cycle double-helix structure known as the DNA. If the bases are regarded and the nuclear export processes; Boisvert et al., 2007; Schmidt- as genetic letters, then the three consequent bases make a genetic Zachmann, 2005). Moreover, the recent investigations indicate a word, and finally the neighbouring words make a genetic sentence possible connection between the malfunction of the nucleolus and or the so called gene. For example, the sequence of ATG TTA CTC GTA cell aging (Guarente, 2007; Scaffi di et al., 2005). bases can represent the gene that specifies the colour of the hair. The genes contain the genetic instructions that are used to control the 4.9. Ribosome cell activities (Alberts et al., 2008; Becker et al., 2006; Bloomfield et al., 2000; Friedland and Kedes, 1985; Hartl and Jones, 2005; Kool The ribosomes are small organelles that immerse within the et al., 2000; Korf, 2006; Lodish et al., 2008; Snustad and Simmons, cytoplasm. They are composed of two parts: the small subunit and 2006). Most of the human genes have been identified during the the large subunit. The two parts are suspended in the cytosol sepa- human genome project (International Human Genome Sequencing rately; however they join and behave as a single entity facilitating Consortium, 2001; The International HapMap Consortium, 2005). the protein synthesis. Each subunit consists of rRNA and protein. It Using DNA microarrays, the parallel examination of several genes is believed that the rRNA component handles the protein synthe- has become possible (Jordan, 2001; Rinaldis and Lahm, 2007) and sis while the protein component stabilises the ribosome structure therefore the molecular profiling of cancer cells has been realised (Alberts et al., 2008; Becker et al., 2006; Karp, 2007; Lodish et al., (Esteller, 2007). 2008; Tobin and Morel, 1997; Voet et al., 2006; Walker and Rapley, The unique helical shape of the DNA has inspired some engineer- 2000). ing studies as follows. The mechanical and structural characteristics The ribosomes have a major role in the protein synthesis as of the helical DNA are analysed in Bao and Suresh (2003), Marko described in Section 5.2. They hold the single amino acid molecules (2005) and Travers and Thompson (2004). A water turbine with and weld them together to form a chain of amino acids, or the helical blades is reported to harness the energy of gulf streams so called protein. The ribosomes can be divided into two groups (Gorlov, 1995, 1998). The unique capability of the DNA to store the according to their produced proteins: free ribosomes and bound genetic information has inspired the idea of molecular informa- ribosomes. The free ribosomes produce the ‘domestic’ proteins, tion storage and processing systems (Ezziane, 2006; Shapiro, 2006; which are consumed by the cell organelles to facilitate their func- Zauner, 2005). tions. These proteins are released within the cytoplasm without further processing. In contrast, the bound ribosomes produce the 4.8.3.2. RNA. It is copied from the DNA to facilitate the cellular ‘foreign export’ proteins, which are secreted to the extracellular operations. RNA is similar to the DNA in many ways. However, there environment via the cell membrane. These proteins need more are some differences between them as follows: (i) The RNA is much delicate packaging and therefore should undergo further process- shorter than the DNA as it carries only a small portion of the infor- ing. Once the bound ribosomes produce these proteins, they attach mation stored on the DNA. The DNA is as big as the hard disk while to the RER membrane. The proteins penetrate the RER interspace the RNA is as big as the flash memory. (ii) The RNA is single-stranded through the translocation proteins embedded on the RER mem- while the DNA is double-stranded. (iii) The RNA includes uracil ‘U’ brane. Then the proteins experience a long journey through RER, base while the DNA contains the thymine ‘T’ base. Conventionally, SER, and Golgi body before being secretion (Alberts et al., 2008; the RNA polymers are classified into three major groups: ribosomal Becker et al., 2006; Berk et al., 2006; Karp, 2007; Liljas, 2004; Lodish RNA (rRNA), transfer RNA (tRNA), and messenger RNA (mRNA). The et al., 2008; Stephens, 2005; Tobin and Morel, 1997; Voet et al., rRNA travels to the nucleolus to produce the ribosome particles, the 2006; Walker and Rapley, 2000). tRNA travels to the cytoplasm to gather the pair amino acids and transfer them to the ribosomes, and the mRNA travels to the cyto- 5. Description of Cells’ Major Chemical Processes plasm to produce the proteins (Bloomfield et al., 2000; Friedland and Kedes, 1985; Hartl and Jones, 2005; Kool et al., 2000; Korf, This section presents two major chemical processes that occur 2006; Snustad and Simmons, 2006). Recent studies about the func- within the cell. They are the energy generation process and the pro- tions of these RNAs can be found in Borukhov and Nudler (2008), tein synthesis process. Despite the comprehensive research work Köhler and Hurt (2007) and Mcnamara (2006). However, another devoted to exploring these enigmatic processes, there are many group of RNAs that is called microRNA (miRNA) was discovered questions yet to be answered. Different cell organelles, or different 15 years ago. The miRNA regulates protein production by binding parts of an individual cell organelle, interact to realise each pro- to the mRNA inside the cytoplasm. After binding, the process of cess. In the following, these complex interactions are translated protein production by the mRNA is inhibited and in some cases into simple flow diagrams, generally used by engineers to deal with the mRNA is degraded (Bushati and Cohen, 2007; Lee and Ambros, multi-component processes. 2001; Pillai, 2005; Kent and Mendell, 2006; Kosik, 2006; L. Zhang et al., 2007; B. Zhang et al., 2007). The function of the described 5.1. Energy Generation RNA groups is described in Section 5.2, where the protein synthesis is discussed. Energy generation is performed by mitochondria. Like any other engine, it burns carbon and oxygen to release energy. The carbon 4.8.4. Nucleolus is mainly carried to the cell as glucose, a 6-carbon sugar with the Nucleolus is a spherical body within the nucleus. It is membrane chemical formula of C6 H12 O2 while the air is carried to the cell via less and surrounded by the chromatins. The main function of the the blood vessels. The products of the burning process are carbon nucleolus is to produce the ribosome particles. This function is initi- dioxide and water molecules. These are consumed within the cell, ated by be copying of the ribosomal RNA (rRNA) polymers from the or leave the cell via blood vessels. The energy that is released during 326 K. Khoshmanesh et al. / Computational Biology and Chemistry 32 (2008) 315–331 this reaction is stored as adenosine triphosphate (ATP) molecule, a The overall reaction of Krebs cycle can be summarised as fol- heavy compound of adenine, ribose and phosphate with the chem- lows: ical formula of C10 H16 N5 O13 P3. When energy is needed, each ATP molecule loses one of its phosphates to form adenosine diphos- phate (ADP) molecule and release 7.3 kcal of energy as follows (Berg et al., 2007; Champe et al., 2005; Garrett and Grisham, 2007; Mathews et al., 2000; Nelson and Cox, 2005; Pratt and Cornely, 2004): C6 H12 O2 + 6O2 → 6CO2 + 6H2 O + 8ATP (1) (4) ATP + H2 O → ADP + PO4 3− + 7.3 kcal (2) iii. The electron transport chain reactions occur along the inner The process of energy generation in the cell is not as simple membrane of the mitochondria. The route of the inner mem- as described above. It occurs in a series of biochemical reactions brane is windy to provide a large surface area, and moreover is known as glycolysis, Krebs cycle, and electron transport chain that equipped with protein complexes and electron shuttles to facil- are explained below: itate its performance. The reactions can be divided into six steps as follows (Berg et al., 2007; Champe et al., 2005; Garrett and Grisham, 2007; Mathews et al., 2000; Nelson and Cox, 2005; i. The glycolysis reaction occurs within the cytosol. During this Pratt and Cornely, 2004): reaction, the 6-carbon glucose molecule is broken into two (a) The reactions are initiated by the coenzymes such as NADH halves and converted to two 3-carbon molecules known as pyru- and FADH2 that were produced through the glycolysis and vate (C3 H4 O3 ). To facilitate this process, the cytoplasm employs Krebs cycle reactions. To do so, the coenzymes donate their two special chemical compounds known as cytoplasm enzymes electrons to the protein complexes of the inner membrane. and cytoplasm coenzymes. The cytoplasm enzymes are the large (b) The electrons pass through the protein complexes and protein molecules that act as catalysers and speed up the reac- release some of their internal energy. This energy triggers tions. On the other hand, the cytoplasm coenzymes are the the protein complexes to pump out some H+ ions from the non-protein molecules that assist the enzymes. The vitamins matrix to the intermembrane space of the mitochondria. are among the most well known coenzymes. They often serve (c) The electrons travel between the protein complexes via the as intermediate carriers of electrons, specific atoms or func- electron shuttles. The process of pumping out H+ ions con- tional groups that are exchanged during the overall reaction. The tinues until the energy level of the electrons drops below a contributing coenzyme involved in the process is nicotinamide certain value. At that point the electrons return to the matrix. adenine dinucleotide (NAD+ ), which resumes its role in Krebs The electrons donated by NADH can pass through three pro- cycle, as well as electron transport chain. The overall reaction tein complexes while the electrons donated by FADH2 can of glycolysis can be summarised as follows (Berg et al., 2007; only pass through two protein complexes. Champe et al., 2005; Garrett and Grisham, 2007; Mathews et (d) Once the electrons return to the matrix, they are absorbed al., 2000; Nelson and Cox, 2005; Pratt and Cornely, 2004): by the oxygen molecules. The oxygen molecules become charged and the H+ ions of the matrix gather around them to receive the electrons. A chemical reaction occurs between H+ and oxygen that results in the formation of H2 O. The reaction is associated with releasing some energy that is consumed (3) to pump out more H+ ions from the matrix. (e) The consequent pumping out of H+ ions increases the con- ii. The Krebs cycle reactions occur in the matrix of the mitochon- centration gradient of positive ions over the two sides of the dria. The pyruvate molecules that were produced during the inner membrane. The gradient then pumps in the H+ ions glycolysis enter the matrix to feed the cycle. The cycle involves to the matrix through some specific proteins know as ATP the periodic composition and decomposition of 6-, 5- and 4- synthesis complexes. carbon molecules, which results in the gradual releasing of (f) The ATP complexes act as hydroelectric turbines. They har- pyruvate energy content. The cycle reactions can be divided into ness the energy of the flowing H+ ions and consume it to four steps as follows (Berg et al., 2007; Champe et al., 2005; weld the ADP molecules and organic phosphates that exist Garrett and Grisham, 2007; Mathews et al., 2000; Nelson and within the matrix. The result is the ATP molecule that acts as Cox, 2005; Pratt and Cornely, 2004): the energy carrier throughout the cell. (a) To initiate the cycle, the 3-carbon pyruvate molecule loses a carbon dioxide and is converted into a 2-carbon molecule The overall reaction of electron transport chain be summarised of acetyl group. The acetyl reacts with a matrix coenzyme as follows (Berg et al., 2007; Champe et al., 2005; Garrett and that is called coenzyme-A to form acetyl coenzyme-A. Grisham, 2007; Mathews et al., 2000; Nelson and Cox, 2005; Pratt (b) The acetyl coenzyme-A reacts with a 4-carbon molecule of and Cornely, 2004): oxaloacetate that exists in the matrix to form a 6-carbon molecule of citrate. (c) The citrate loses a carbon dioxide to form a 5-carbon molecule of ␣-ketoglutarate. (d) The ␣-ketoglutarate loses the last carbon dioxide to repro- duce the 4-carbon molecule of oxaloacetate and restart the cycle. The contributing coenzymes involved in the process are NAD+ and flavin adenine dinucleotide (FAD). (5) K. Khoshmanesh et al. / Computational Biology and Chemistry 32 (2008) 315–331 327 Fig. 12. Chemical reactions of the mitochondria that tend to energy generation within the cell. Fig. 12 integrates the chemical reactions that occur in glycolysis, plasm, and put them in a row. The ‘C’ bases pair with the ‘G’ bases Krebs cycle, and electron transport chain and result in the energy while the ‘A’ bases prefer to pair with the ‘U’ bases (Alberts et al., generation by the cell. 2008; Becker et al., 2006; Karp, 2007; Lodish et al., 2008; Tobin Several models have been developed to simulate the biochem- and Morel, 1997; Voet et al., 2006; Walker and Rapley, 2000). ical reactions happening within the cytoplasm and mitochondria iii. The process continues until the enzyme reaches the termination (Banaji, 2006; Bertrama et al., 2006; Loebl and Matthai, 2004; sequence of the DNA, where it leaves the DNA (Alberts et al., Moser et al., 2006; Peterson et al., 2007; Wu et al., 2007). From 2008; Becker et al., 2006; Karp, 2007; Lodish et al., 2008; Tobin engineering perspective, the mitochondria is an enzymatic bio- and Morel, 1997; Voet et al., 2006; Walker and Rapley, 2000). fuel cell that generates electric power from the burning of glucose. iv. The outcome of this process is the formation of a messen- Design and fabrication of biofuel cells that mimic the mitochon- ger RNA (mRNA) polymer that serves as an information carrier dria is reported in Barrière et al. (2006), Furukawa et al. (2006), throughout the cell. The mRNA migrates from the nucleus to Gao et al. (2007), Moore et al. (2005), Togo et al. (2007) and Zhang the cytoplasm. The mRNA is similar to DNA but is shorter, single et al. (2006). The simplicity and biocompatibility of these systems stranded, and contains the uracil ‘U’ bases instead of the thymine make them good candidates to serve as the power source of the ‘T’ bases. The sequence of each three neighbouring bases of the in vivo bioelectronic devices such as micropumps, pacemakers and mRNA is called the codon. There are twenty different types of neuromorphic circuits. amino acids available within the cytoplasm. The codon indicates 5.2. Protein Synthesis The process of protein production by the cell is called protein synthesis. A protein is a long chain of amino acids that are attached by peptide bonds. In order to produce a protein, three elements are needed: (i) a genetic template to recall the appropriate amino acid subunits from the cytoplasm, (ii) the amino acid subunits that form the chain, and (iii) a machine to join and weld the amino acids to make a peptide bond. The first element is provided trough the