Female Reproductive System - Biology Textbook
Document Details
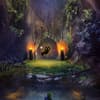
Uploaded by iiScholar
Arizona State University
Tags
Summary
This document is a section from a biology textbook covering the female reproductive system. Key topics include female reproductive anatomy, oogenesis (the creation of egg cells), and processes like fertilization. The content is designed to provide a detailed understanding of human reproductive biology, including the roles of key organs like ovaries and the uterus.
Full Transcript
**Female Reproductive System** Introduction The female reproductive system participates in human reproduction by generating haploid (1*n*) gametes known as **oocytes** and providing a supportive environment for fertilization and growth of offspring. The concepts in this lesson provide an overview...
**Female Reproductive System** Introduction The female reproductive system participates in human reproduction by generating haploid (1*n*) gametes known as **oocytes** and providing a supportive environment for fertilization and growth of offspring. The concepts in this lesson provide an overview of female reproductive anatomy, the process of gamete formation via **oogenesis**, and hormonal regulation of the female reproductive system. 9.3.01 Female Reproductive Anatomy The internal and external structures of the female reproductive system are responsible for producing gametes (ie, oocytes), receiving sperm, and providing a supportive environment for fertilization, gestation, and nourishment of offspring. As depicted in Figure 9.10, the following structures are associated with the female reproductive system: **Ovaries** are reproductive gonads that secrete sex hormones (eg, estrogens, progesterone) and serve as the sites of oogenesis. The **uterine (fallopian) tubes** are a pair of muscular tubes originating from the uterus and extending laterally toward each ovary. Although the open ends of the uterine tubes are near the ovaries, there is not a direct connection between the ovary and uterine tube. The open ends of uterine tubes are surrounded by **fimbriae**, fingerlike projections that facilitate direction of the oocyte from the abdominal cavity into the uterine tubes. Ciliated cells line the interior of the uterine tubes and help propel the oocyte toward the uterus. Fertilization typically takes place in the uterine tubes. The **uterus** is a muscular organ responsible for protecting and nourishing the embryo and fetus. The inner lining of the uterus, called the **endometrium**, undergoes cyclical changes in thickness at different points in the menstrual cycle. The uterus also contains a thick layer of smooth muscle called the **myometrium**, which contracts during menstruation and childbirth. The **cervix** is the most inferior portion of the uterus and serves as the opening into the vagina. The **vagina** is a muscular tube that functions in elimination of menstrual fluids during the menstrual cycle, in reception of the penis during sexual intercourse, and as the final segment of the birth canal during childbirth. **Female external genitalia (vulva)** include the folds of the **labia majora** (singular: **labium majus**) and **labia minora** (singular: **labium minus**), which protect the external opening of the vagina and the **clitoris**. The external portion of the clitoris lies at the junction of the labia minora folds. Stimulation of the densely innervated clitoris triggers genital changes (eg, lubrication, pH changes) that facilitate reproductive success. **Mammary glands** are accessory glands located within the chest wall that become fully developed during pregnancy to facilitate **lactation** (ie, the synthesis and secretion of breast milk) and nursing. Chapter 9: Reproductive System 329 **Figure 9.10** Structures of the female reproductive system. The ovaries are a pair of female gonads homologous (ie, possess the same embryonic origin) to testes. Each ovary is surrounded by an outer fibrous capsule covered by epithelial cells. Within an ovary, the cortex contains many **ovarian follicles** in various stages of maturation. Each follicle contains an immature primary oocyte surrounded by supporting epithelial cells (Figure 9.11). In the early stages of follicle maturation, the supporting follicular cells proliferate and mature into **granulosa cells**. Granulosa cells support developing oocytes by secreting sex steroid hormones such as estrogens (eg, estradiol) during the ovarian cycle. A diagram of a person\'s uterus AI-generated content may be incorrect. Chapter 9: Reproductive System 330 **Figure 9.11** Ovary structure. 9.3.02 Oogenesis **Oogenesis** begins during embryogenesis, as **oogonia** (stem cells) within developing ovaries undergo continuous rounds of [mitotic division](javascript:void(0)) to yield identical diploid (2*n*) daughter cells. Unlike spermatogonia, which initiate [meiosis I](javascript:void(0)) beginning at puberty, oogonia initiate meiosis I to form **primary oocytes** during the fetal period. Primary oocytes are arrested (ie, paused) in late prophase I and do not resume the stages of meiosis I again until the beginning of puberty, in response to hormonal changes (see Concept 9.3.03). At the onset of puberty, these primary oocytes are surrounded by follicular support cells in the ovary, forming **ovarian follicles**. Hormonal changes during each menstrual cycle typically result in a single ovarian follicle being selected to continue meiosis and proceed with **ovulation**. At the completion of meiosis I, unequal cell division of the chosen primary oocyte results in cells of two different sizes: a larger haploid (1*n*) **secondary oocyte** and a smaller haploid cell called the **first polar body** (Figure 9.12). In humans, the first polar body typically undergoes [apoptosis](javascript:void(0)) and degenerates but may complete meiosis in some cases. The secondary oocyte initiates [meiosis II](javascript:void(0)) but arrests at metaphase II until fertilization.  Chapter 9: Reproductive System 331 **Figure 9.12** Oogenesis. A diagram of a diagram of a person\'s life cycle AI-generated content may be incorrect. Chapter 9: Reproductive System 332 During ovulation, the ovarian follicle ruptures and the secondary oocyte, surrounded by a thick glycoprotein matrix known as the **zona pellucida** and a group of granulosa cells known as the **corona radiata**, is released into the abdominal cavity. The secondary oocyte is drawn into a **uterine (fallopian) tube**, where fertilization by a sperm cell can occur. In the event of successful fertilization, the secondary oocyte then completes meiosis II to form one large **ovum** (ie, fully mature gamete) and a small **second polar body** that degenerates (Figure 9.12). After fertilization is complete, the male and female **pronuclei** are fused (see Concept 10.1.01) to form a diploid **zygote**. During oogenesis, each oogonium yields one haploid gamete (ovum) and two to three haploid polar bodies, as opposed to the four haploid gametes (sperm) generated during spermatogenesis. Oogenesis begins before birth and can result in mature gametes 13--50 years later. As levels of sex hormones (eg, estrogens, progesterone) decline with age during a state known as **menopause**, oogenesis ceases. Unlike spermatogenesis, which typically occurs throughout the lifespan after the onset of puberty, it is thought that a finite number of primary oocytes are formed during the fetal period that are not typically replenished later in life. 9.3.03 Hormonal Control of the Female Reproductive System During the early weeks of embryogenesis, an XY embryo typically begins to develop male reproductive organs initiated by *SRY* gene expression (see Concept 9.2.03). In XX embryos, *SRY* is absent and development of testes typically does not occur. As a result, **anti-Müllerian hormone (AMH)**, is not produced and the development of female reproductive structures derived from **Müllerian (paramesonephric) ducts** is promoted. Because testes are not formed, **Wolffian (mesonephric) ducts** degenerate due to a lack of testosterone. The first stage of oogenesis begins during the fetal period with the production of follicles containing primary oocytes, as discussed in Concept 9.3.02. During infancy and childhood, sex hormone levels are low and progression of oogenesis is repressed; therefore, sexual development remains dormant until the onset of puberty. At puberty, the secretion of **estrogens** and **progesterone** (ie, sex steroid hormones) promotes the growth and maturation of female reproductive organs, development of secondary sex characteristics, resumption of oogenesis, and initiation of the menstrual cycle. The **hypothalamic-pituitary-gonadal (HPG) axis** regulates a series of cyclical changes that make reproduction possible (Figure 9.13). The female reproductive cycle includes the **ovarian cycle** (ie, events that occur during the maturation of a primary oocyte) and the **uterine (menstrual) cycle** (ie, events that occur in the uterus to prepare for pregnancy). The ovarian and uterine cycles occur concurrently, with a single female reproductive cycle lasting an average of 28 days. Synchronization of the ovarian and uterine cycles provides optimal conditions for the support of fertilization and early pregnancy. Chapter 9: Reproductive System 333 **Figure 9.13** Regulation of the female reproductive system. The ovarian cycle can be divided into three phases (Figure 9.14): **Follicular phase** (days 1--13): Stimulation of the HPG axis results in the release of **gonadotropin-releasing hormone (GnRH)** from the hypothalamus, stimulating the anterior pituitary gland to release small amounts of **follicle-stimulating hormone (FSH)** and **luteinizing hormone (LH)**. FSH and LH stimulate developing ovarian follicles to release estrogens. During the early follicular phase, low to moderate estrogen levels exert negative feedback on the HPG axis, and only one developing  Chapter 9: Reproductive System 334 follicle typically survives. The surviving (dominant) follicle secretes **inhibin**, which acts on the anterior pituitary to inhibit FSH, repressing maturation of additional follicles during the same ovarian cycle. Although estrogen initially inhibits the HPG axis, the emergence of a dominant follicle stimulates the secretion of high levels of estrogen, exerting a *stimulatory* effect on the HPG axis during the late follicular phase. This effect results in a surge of LH and, to a lesser extent, FSH. **Ovulation** (day 14): Soon after the LH surge, the mature ovarian follicle ruptures, and a secondary oocyte is released into the abdominal cavity, entering a nearby uterine tube. **Luteal phase** (days 15--28): LH stimulates the conversion of the ruptured follicle into a structure known as the **corpus luteum**, which secretes high levels of progesterone and estrogens, exerting negative feedback on the HPG axis. Lower FSH and LH levels during the luteal phase prevent maturation of additional follicles. If fertilization does not occur, the corpus luteum degenerates, causing a sharp decline in progesterone and estrogens. This decline relieves the inhibition of FSH and LH, allowing the next ovarian cycle to begin. If the secondary oocyte is fertilized following ovulation, the corpus luteum persists and continues to secrete progesterone and estrogens. The continued presence of progesterone and estrogens keeps FSH and LH levels low, promoting the pregnancy and preventing a new ovarian cycle from being initiated during pregnancy. **Figure 9.14** The events of the ovarian and uterine cycles. A diagram of a diagram of the ovulation cycle AI-generated content may be incorrect. Chapter 9: Reproductive System 335 The uterine (menstrual) cycle occurs concurrently with the ovarian cycle and is also divided into three phases (Figure 9.14): **Menstrual phase** (days 1--4): If fertilization of a secondary oocyte does not occur in the prior uterine cycle, **menstruation** begins. During **menses**, the uterus sheds the majority of its endometrial (ie, inner) layer. The detached tissue and blood pass out of the body through the vagina. Toward the end of this phase, ovarian follicles are stimulated to grow and produce estrogens, resulting in the cessation of blood flow. **Proliferative phase** (days 5--14): The endometrial layer proliferates, doubling in thickness. Endometrial glands develop and vascularization of the endometrium is increased to prepare for embryo implantation. **Secretory phase** (days 15--28): Increased progesterone and estrogen secretion from the corpus luteum triggers the further thickening and development of the endometrium. These changes result in secretion of nutrients from endometrial glands and the creation of an environment that can sustain a developing embryo in the event of fertilization and implantation. **Concept Check 9.2** Ovarian function declines naturally with age, resulting in the cessation of the ovarian and menstrual cycles (ie, menopause) around age 45--55 for most individuals. As menopause advances, the number of functional ovarian follicles decreases, and less estrogen is produced by the ovaries. What effect would this decrease in estrogen levels have on the regulation of the HPG axis in a menopausal individual? [**Solution**](javascript:void(0))  **Pre-Implantation Development** Introduction Embryonic development begins when male and female gametes join to form a zygote (ie, fertilization). Although fertilization typically takes place in a uterine tube within 12--24 hours after ovulation, the developing embryo must then travel to the uterine cavity and implant for a viable pregnancy to occur. The developmental steps between fertilization and implantation take place within 12 days after ovulation. This lesson covers **pre-implantation development** from the time of fertilization until the completion of implantation, as well as development of the **placenta** (ie, transient organ which mediates fetal-maternal gas and nutrient exchange). 10.1.01 Fertilization and Blastulation **Fertilization** (ie, joining of male and female gametes to form a zygote) becomes possible with the release of the secondary oocyte from the ovary (ie, **ovulation**). Upon ovulation, the oocyte travels into the abdominal cavity and is drawn into the **uterine (Fallopian) tube** by densely ciliated projections known as **fimbriae**. The ciliated lining of the uterine tube helps propel the oocyte into the uterine cavity. Sperm typically make contact with the oocyte within the uterine tube. Successful fertilization requires the following steps, as outlined in Figure 10.1: 1.**Capacitation:** To reach the oocyte, sperm must undergo a final maturation step in the uterine tube; this step is known as capacitation. Female reproductive tract secretions induce changes in plasma membrane permeability at the sperm head and trigger increased flagellar movement (ie, motility). 2.**Contact with oocyte:** The sperm moves past follicular cells of the oocyte\'s **corona radiata**, toward the **zona pellucida** (ie, thick matrix of glycoproteins between the corona radiata and plasma membrane) to reach the oocyte. Receptors located in the sperm head bind to glycoproteins in the zona pellucida. 3.**Acrosome reaction:** Located within the sperm head, the acrosome is a specialized vesicle filled with hydrolytic enzymes (see Concept 9.2.02). These enzymes are released near the oocyte, leading to zona pellucida degradation, thereby enabling the sperm to reach the oocyte\'s plasma membrane. 4.**Fusion:** Oocyte and sperm plasma membranes fuse, triggering depolarization of the oocyte\'s plasma membrane. 5.**Sperm contents entering the oocyte:** The sperm nucleus, mitochondria, and a pair of centrioles enter the oocyte. However, most sperm mitochondria are destroyed following oocyte entry (see Concept 7.3.06). 6.**Cortical reaction:** Upon sperm fusion, oocyte plasma membrane depolarization leads to increased intracellular calcium (Ca2+) levels, which triggers **cortical granules** (ie, secretory vesicles containing hydrolytic enzymes) to fuse with the oocyte\'s plasma membrane. Hydrolytic enzymes are released into the space between the plasma membrane and the zona pellucida, causing the zona pellucida to lift away from the oocyte and harden. The cortical reaction results in the formation of a protective envelope that blocks additional sperm from entering (ie, **polyspermy**). Chapter 10: Pregnancy, Development, and Aging 337 **Figure 10.1** Fertilization sequence. Following sperm and oocyte plasma membrane fusion, sperm contents can enter the oocyte. The secondary oocyte must then complete [meiosis II](javascript:void(0)), dividing to form a mature ovum and second polar body, which degenerates (see Concept 9.3.02). The nucleus of the mature ovum develops into a **female pronucleus**. Simultaneously, the **male pronucleus** (ie, sperm nucleus) is propelled toward the female pronucleus. Fusion of haploid (1*n*) pronuclei produces a diploid (2*n*) cell known as a **zygote**. Figure 10.2 provides an overview of the events of spermatogenesis and oogenesis, which culminate in fertilization and zygote production. Diagram of a cell cycle AI-generated content may be incorrect. Chapter 10: Pregnancy, Development, and Aging 338 **Figure 10.2** Overview of gametogenesis and fertilization. Immediately following zygote formation, epigenetic modifications (eg, [chromatin remodeling](javascript:void(0)), [DNA](javascript:void(0)) [methylation](javascript:void(0)) changes) occur and allow for developmental [totipotency](javascript:void(0)), the ability to give rise to all embryonic cell types. Notably, some parental epigenetic modifications (eg, imprinted genes) are maintained (see Concept 10.3.02). Within 24 hours after fertilization, the zygote begins a period of rapid [mitotic cell division](javascript:void(0)) known as embryonic **cleavage** (Figure 10.3). During cleavage, cell division proceeds without concurrent cell growth  Chapter 10: Pregnancy, Development, and Aging 339 (ie, daughter cells become progressively smaller compared to the zygote), forming a mass of identical cells known as **blastomeres**. After 3--4 days, the embryo enters the **morula** stage. The morula, roughly the same size as the original zygote, consists of 16--32 blastomeres. During the transition from zygote to morula, the developing embryo travels from the uterine tube towards the uterine cavity. By days 4--5, the rapidly dividing embryonic cells reorganize into a **blastocyst**, which contains a hollow, fluid-filled cavity known as the **blastocoel**. The blastocyst contains two distinct cell populations: an **inner cell mass**, which contains cells that develop into the embryo, and a **trophoblast**, the outer cell layer that develops into extraembryonic tissues (eg, placenta). **Figure 10.3** Early embryonic development from zygote to blastocyst. **Concept Check 10.1** For successful fertilization of the oocyte by the sperm, a series of steps must occur in sequence. Using the following list, provide a rationale for why unsuccessful completion of each step could lead to a failed fertilization sequence: 1.Capacitation 2.Acrosome reaction 3.Cortical reaction [**Solution**](javascript:void(0)) Diagram of a cell structure AI-generated content may be incorrect.  Chapter 10: Pregnancy, Development, and Aging 340 10.1.02 Implantation and Placental Development For development to continue beyond the blastocyst stage, the blastocyst must implant into the uterine lining (Figure 10.4). By 6--7 days after fertilization, surges in progesterone and estrogen trigger the secretory phase of the uterine cycle, and the endometrial lining is primed to support **implantation** of the blastocyst (see Concept 9.3.03). Developing trophoblastic cells (ie, cells from the outer portion of the blastocyst) adhere to the endometrial surface, causing the blastocyst to burrow into the endometrial lining. Endometrial cells proliferate and surround the blastocyst, now known as the **embryo**, sealing it off from the uterine cavity. Until the placenta is completely formed, the endometrium supports and nourishes the developing embryo. **Figure 10.4** Blastocyst implantation. The process of implantation is usually complete by day 12. If fertilization does not occur, the corpus luteum typically degenerates, and declining progesterone and estrogen levels trigger menstruation. However, when successful implantation occurs, the developing trophoblastic cells begin to secrete the hormone **human chorionic gonadotropin (hCG)**, and the corpus luteum is maintained while the placenta develops. The **placenta** forms over the first 3 months of pregnancy to facilitate nutrient, gas, and waste exchange between the maternal circulation and the developing embryo. An extraembryonic membrane known as the **chorion** is derived from the trophoblast following implantation. **Chorionic villi**, fingerlike projections that invade the endometrium, form from the chorion and become vascularized. The chorion and chorionic villi form the bulk of the placenta (Figure 10.5). The corpus luteum continues to secrete estrogen and progesterone for approximately the first 9 weeks of pregnancy, effectively inhibiting the initiation of another menstrual cycle. However, the developing placenta gradually takes over secretion of hCG, estrogens, and progesterone to support the pregnancy, leading to corpus luteum degeneration. Diagram of a cell cycle AI-generated content may be incorrect. Chapter 10: Pregnancy, Development, and Aging 341 **Figure 10.5** Developing placenta and extraembryonic structures. The embryo is supported by several extraembryonic structures derived largely from the blastocyst inner cell mass before and during placenta formation (Figure 10.5): **Yolk sac:** a region where maternal-fetal nutrient and gas exchange occurs prior to placenta formation and the main site of embryonic blood cell production prior to the fetal period. **Allantois:** formed from the yolk sac, a structure that mediates fluid and waste exchange between the embryo and yolk sac. The umbilical cord is eventually formed from the yolk sac and allantois. **Amnion:** a tough membrane filled with amniotic fluid that surrounds the embryo. Once fully developed, the placenta receives blood from both the maternal and the fetal circulatory systems, although these two systems do not typically intermix (see Concept 10.4.02).  Chapter 10: Pregnancy, Development, and Aging 342 Lesson 10.2 **Post-Implantation Development** Introduction Successful implantation of the blastocyst signals the beginning of the embryonic period of development. **Organogenesis** (ie, the development of embryonic tissues and organs) begins with **gastrulation**, a period of rapid growth and reorganization of embryonic cells. By the end of gastrulation, the three primary germ layers are established, from which all embryonic tissues and organs are formed. Shortly thereafter, mesodermal tissue induces the overlying ectoderm to become neural tissue, in a process known as **neurulation**. By the end of the embryonic period, the development of all major body systems is well underway and continues throughout the remainder of the pregnancy. This lesson covers the major events of post-implantation development during the embryonic period. More information about the fetal period, along with other aspects of pregnancy and parturition, can be found in Lesson 10.4. 10.2.01 Gastrulation At the time of implantation, the blastocyst consists of two major cell types: trophoblast cells and the inner cell mass (ICM). Before gastrulation, the ICM cells are organized into two cell types: the **epiblast** (upper layer) and **hypoblast** (lower layer). A bilaminar (ie, two-layered) disk, consisting of a portion of the epiblast and the hypoblast, eventually becomes the embryo proper. The cells of the epiblast form the embryo and amnion, and the cells of the hypoblast form a portion of the chorion and the yolk sac, structures that are discussed in more detail in Concept 10.1.02. During week three of development, ICM cells undergo a period of rapid growth, differentiation, and cellular rearrangement known as **gastrulation**, establishing the three **primary germ layers** from which all embryonic tissues and organs arise: ectoderm, mesoderm, and endoderm. Gastrulation begins with the formation of a groove known as the **primitive streak** within the epiblast which establishes the anterior-posterior axis of the embryo. Along the primitive streak, cells that will form the internal organs migrate into the interior space of the embryo, and cells that will form the skin and nervous system migrate along the outer surface. The process of gastrulation is illustrated in Figure 10.6. Chapter 10: Pregnancy, Development, and Aging 343 **Figure 10.6** Gastrulation. The **blastopore**, or first point of invagination during gastrulation, forms as endodermal cells invaginate at the posterior end of the primitive streak. In mammals, the blastopore eventually becomes the anal opening of the embryo. As development continues, further migration of endodermal cells creates a primitive gut cavity called the **archenteron**. As the three primary germ layers are formed, the relatively flat embryonic disk also undergoes significant changes in size and shape. Because of asynchronous rates of growth and cell movement, the embryo folds into a three-dimensional form in which ectodermal cells are largely found on the exterior of the embryo, endodermal cells are found on the interior, and mesodermal cells are found in between (Figure 10.6). Gastrulation results in a three-layered embryo called a **gastrula**. Following gastrulation, the three primary germ layers have different fates, as depicted in Figure 10.7: The **ectoderm** develops into the skin and nervous system. The **mesoderm** gives rise to muscle, bone, and other connective tissues. The **endoderm** forms the lining of the digestive tract and other internal organs. A diagram of the stages of a stage AI-generated content may be incorrect. Chapter 10: Pregnancy, Development, and Aging 344 **Figure 10.7** Germ layer derivatives. A more comprehensive list of germ layer derivatives is included in Table 10.1. Note: Although it is unlikely that every germ layer derivative must be memorized, past exams have included highly specific questions regarding germ layer derivatives, with particular attention to counterintuitive examples (eg, adrenal medulla is derived from ectoderm, whereas adrenal cortex is derived from mesoderm).  Chapter 10: Pregnancy, Development, and Aging 345 **Table 10.1** Detailed derivatives of the three primary germ layers. 10.2.02 Organogenesis and Neurulation The formation of specific [tissues](javascript:void(0)) and organ systems begins after primary germ layer establishment. During **organogenesis**, progressive cell differentiation turns the relatively undifferentiated primary germ layers into functional organs and organ systems. Exposure to signaling molecules from an underlying mesodermal structure called the **notochord** induces overlying ectodermal cells to form neural tissues in a process called **neurulation**. Three ectodermal cell types arise during neurulation: **epidermal** cells (eg, the outer layer of the [skin](javascript:void(0))), **neural crest** cells (eg, precursor cells of the [peripheral nervous system](javascript:void(0)) \[PNS\], [melanocytes](javascript:void(0))) and **neural plate** cells (eg, precursor cells of the central nervous system \[CNS\]). At this stage of embryogenesis, the embryo is called a **neurula**. Once ectodermal cell types are specified, neurulation continues in the neural plate via the following steps (Figure 10.8): 1.Thickening and elongation of the neural plate in response to additional signals from the underlying mesoderm. 2.Upward movement of the edges of the neural plate to form **neural folds** on either side of a central **neural groove**. 3.Migration of lateral edges of the neural folds toward the midline of the embryo, fusing to form a **neural tube** that sits inferior to an overlying ectodermal layer. Neural crest cells **delaminate** (ie, detach) from the neural tube as the tube closes and these cells migrate to various regions throughout the embryo. A white sheet with black text AI-generated content may be incorrect. Chapter 10: Pregnancy, Development, and Aging 346 **Figure 10.8** Neurulation. Neural crest cells differentiate into a number of diverse cell types, most notably the neurons and [glia](javascript:void(0)) of the PNS (eg, sensory and autonomic ganglia, adrenal medulla, Schwann cells), melanocytes (pigment cells), [calcitonin-producing thyroid cells](javascript:void(0)), and facial connective tissue. After neural tube formation, organogenesis continues with the segmentation of mesodermal tissues adjacent to the neural tube into **somites**, clusters of muscle and connective tissue precursor cells found in the back (eg, precursors to vertebrae, ribs, dermis). Concurrently, tissues derived from nearby mesoderm and endoderm give rise to the remaining organs and organ systems. See Concept 10.2.01 for more details about the structures derived from each germ layer. A progression of the stages of pre- and post-implantation development is shown in Figure 10.9.  Chapter 10: Pregnancy, Development, and Aging 347 **Figure 10.9** Summary of pre- and post-implantation development from fertilization to neurulation. **Concept Check 10.2** Sort the following tissues by the germ layer of origin (ectoderm, mesoderm, or endoderm): Adrenal medulla Dermis Rib cartilage Alveoli of lung Oral epithelium Schwann cells Anterior pituitary gland Red blood cells Sympathetic ganglia Capillary endothelium Reproductive tract epithelium Urinary bladder epithelium [**Solution**](javascript:void(0)) A diagram of a gastrointestinal tract AI-generated content may be incorrect.  **Have you mastered this Lesson?** Mark this lesson as complete and continue to the next. Mark as Complete