Biology 100 Study Guide.docx
Document Details
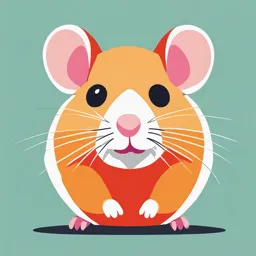
Uploaded by CohesiveAgate5503
Full Transcript
Biology 100 Study Guide Key: Words in this colour are extra information, probably not test material Bolded signify emphasis and importance Highlights stress importance of phrases/syntax 30 Aug (W) 1 Course Intro, Nature of Science “Scientific knowledge is a body of statements of varying degrees of...
Biology 100 Study Guide Key: Words in this colour are extra information, probably not test material Bolded signify emphasis and importance Highlights stress importance of phrases/syntax 30 Aug (W) 1 Course Intro, Nature of Science “Scientific knowledge is a body of statements of varying degrees of certainty -- some most unsure, some nearly sure, none absolutely certain.” - R.P. Feynman (1918 – 1988) Science serves as a structured approach aimed at assisting individuals in determining their existing understanding, uncovering fresh insights, and assessing the level of certainty they can have in their knowledge. The Scientific Method • The “scientific method” is used within science to investigate how things (in science it’s typically used for natural phenomena’s) work; to gain new knowledge/insight. The Scientific Method is a structured process consisting of several elements: Observation: The first step is to make an observation about a particular phenomenon or ask a question about it. This observation or question often arises from curiosity or prior knowledge. Research: Scientists then conduct background research to gather information and understand what is already known about the topic. This helps in forming hypotheses and designing experiments or studies. Hypothesis: A hypothesis is a testable explanation or educated guess based on the available information. It is a statement that predicts the outcome of an experiment or observation. Hypotheses should be clear, specific, and falsifiable. Experimentation: Scientists design experiments or conduct observations to test their hypotheses. This involves setting up controlled experiments, collecting data, and ensuring that the variables are manipulated and measured systematically. Data Collection: During the experiment, data is collected and recorded. This data should be both accurate and objective, ensuring that the results are reliable. Analysis: Scientists analyze the data using statistical and analytical methods to determine whether the results support or reject the hypothesis. They look for patterns, trends, and relationships in the data. Conclusion: Based on the analysis of the data, scientists draw conclusions. If the results support the hypothesis, it may become a theory or be incorporated into existing scientific knowledge. If the results do not support the hypothesis, scientists may revise their hypotheses and conduct further experiments. Communication: Scientists communicate their findings through research papers, presentations, or other means. This allows others to review and replicate the experiments, contributing to the collective knowledge in the field. Peer Review: The scientific community often relies on peer review to ensure the quality and validity of research. Other experts in the field review the work before publication to identify any flaws or areas for improvement. Repetition: Scientific findings are not considered conclusive until they have been independently verified through repetition by other scientists. This repetition helps confirm the reliability of the results. In Science; reasoning, logic and evidence are extremely important Philosophers of Science often Describe Two Types of Science: induction-based science (also called discovery science) Inductive Reasoning (Inductive Science) approach science through conclusions or general principles based on specific observations or evidence. The method involves making a series of observations to form a generalization or hypothesis. Example: Observation: A person observes that every morning for the past week, the sun has risen in the east. Pattern Recognition: After several days of consistent observations, the person notices a pattern that the sun consistently rises in the east. Hypothesis Formation: Based on this pattern, the person forms a hypothesis: "The sun always rises in the east." Testing and Confirmation: Over the course of the next few weeks or even months, the person continues to observe the sunrise, and each time, they find that the sun does indeed rise in the east. Generalization: After many observations and confirmations, the person may generalize the hypothesis into a more general principle: "The sun will always rises in the east." This generalization is based on the repeated observations and the apparent consistency of the phenomenon. hypothesis-based science (Deductive Reasoning) Gets most of its attention from science philosophers Basis of most formulations in “Scientific Method A hypothesis is an educated guess used to explain observed phenomena. It represents an informed speculation regarding an explanation, and it is sometimes referred to as a 'conjecture' Hypothetico-Deductive Method (Hypothesis based Science) The method involves the testing of hypothesis through the conduction of experiments The outcomes of experimentation are often predictable if the hypothesis is correct. (two outcomes: Accepted hypothesis or rejected/refuted hypothesis) The hypothesis is refuted if the prediction is wrong. If hypothesis is consistent with results of experimentation, then the hypothesis is supported (not necessarily prove it Support ≠ Proof) Two Kinds of Hypothesis: Testable Hypothesis: Hypothesis has potential to be shown wrong or incorrect (can be refuted/rejected/falsified) Example: “If people receive 8 hours of sleep per night, their cognitive performance on memory tasks will be significantly better than if they receive only 4 hours of sleep per night.” Non-testable Hypothesis: This type of hypothesis cannot provide testable predictions and cannot be show to be incorrect. The scientific method can’t deal with theses types of Hypotheses, but it’s not necessarily wrong. Example: “Love is the most powerful force in the universe.” All experiment always needs to have a control, without a control there is no way of telling the validity of the experiment Positive Controls: A known treatment that produces an effect similar/identical to what you predict your experimental treatment will produce. (Often part of experiments, but not always: Predominantly used in chemical analysis). Negative Control: Nothing Added (Mandatory for all experiments) “Old ideas are rejected; new ideas are tested” Hypothesis that attempts to explain a large number of phenomena of a large scope and are generally applicable, are known as theories. Theories attempt to explain so much, they only become accepted if they are supported by a large body of evidence. Example: Darwin’s theory in evolution and natural selection is widely accepted because of the accumulation of a very large body of evident to support it. *Larger Sample Sizes Reinforce the validity of the experiment* The Experiment must be repeatable and results must be consistently reproducible by anyone, anywhere and any given time. Occam’s Razor If Several explanations are compatible with the evidence at hand. The simplest should be considered the most likely 1 Sep (F) 2 Basic Chemistry Atoms are the basic building blocks of matter It is composed of neutrons, protons and electrons The smallest atom is the hydrogen atom, which consists of 1 proton (positively charged), 1 electron (negatively charged) – no neutron Deuterium – Hydrogen isotope with a neutron in the nucleus If a H atom loses an electron, only the proton remains – this is shown as H+. An Atom is mostly empty space Nucleus – contains protons and neutrons The # of protons defines the type of atom The atomic # of a chemical indicates how much protons it has (not the mass #) Example: Hydrogen has an atomic # of 1 = 1 proton in nucleus Mass Number = # of protons + # of neutrons (the sum of particles in the nucleus) Isotopes: elements with the same protons (atomic #), but different # of neutrons (different mass #) Isotopes can be stable or radioactive Bonds between Atoms are Based on Electrons • There are possibly single, double or triple bonds between two atoms. (Quadruple bonds don’t exist) Carbon always has 4 bonds, at minimum must have bonds to at least two other atoms/molecules Ions - Atoms or molecules in which the number of protons ≠ the number of electrons. There are two types of ions: Cations and Anions Cations – Positively charged ions, in which there are more protons (+1) than electrons (-1). Anions – Negatively charged ions in which there are fewer protons (+1) than electrons (-1). Chemical bonds between atoms are based on electrons in outer orbitals. Chemical bonds are always in pairs; with both molecules involved in the bonding providing one electron each A single bond consists of a two electrons (one pair), a double bond consists of 4 electrons (two pairs) and a triple bond consists of 6 electrons (three pairs). Bonding of two atoms of the same type is (most of the time) non-polar covalent bonds Covalent means that the electrons in the bonds are shared. Non-polar implies that they are shared equally. (No bonding is shared perfectly) Non-polar means that there are no partial charges on the atoms in the bonds Electronegativity - is the ability of an atom to attract electrons to itself A higher EN means a greater ability to attract electrons. Bonds between like atoms (X-X, X=X etc) are thus bonds between atoms that have the same EN. (non-polar covalent) Polar Covalent Bonds - the electrons in the bond are shared unequally, with the electrons shifted toward the atom with the higher EN – Happens to atoms with a moderate difference in EN “Polar” refers to the fact that partial charges (δ-, δ+) are present due to unequal electron sharing. negative charge is shifted towards the atom with higher EN and away from the atom with lower EN leads to partial charges (given the symbol δ- for partial negative and δ+ for partial positive; δ is the lower case version of the Greek letter “delta”). Ionic Bonds - happens if there is a very large difference in EN between atoms in a bond the electrons in the bond are not shared, but actually taken by the atom with larger EN; ionic is the transfer of electrons not sharing (due to very large differences in EN) Non-polar covalent bonds, polar covalent bonds and ionic bonds are all considered to be “strong” bonds Hydrogen Bonds (H bonds) are Weak Bonds They are constantly breaking and reforming Strong bonds are: non-polar covalent, polar covalent, ionic. H bonds: weak, transient bonds that are a consequence of polar covalent bonds containing H. 6 Sep (W) 3 Water, Carbon Water is fundamental to life Cells are approx. 80% water Often described as the solvent of life Water is not a universal solvent, there are no universal solvents The extensive H-bonding in water causes it to be a liquid at room temperature. Other molecules of similar low molecular mass all are gases room temperature. Water exhibits cohesion – the tendency of molecules to stick to or be attracted to identical molecules Example: water molecules are attracted to each other – explains liquid state/nature of water at room temp Water also exhibits adhesion – tendency to stick to/attracted to molecules that are large polar/charged molecules Hydrophilic molecules may be soluble in water, if they are not too large. the solubility of polar molecules decreases as the molecules get larger Hydrophobic Molecules – Non-Polar covalent bond containing molecules Cations are generally hydrophilic Hydrophobic molecules are composed largely of non-polar covalent bonds. Non-polar covalent bonds do not have partial charges, or have relatively few partial charges. Important non polar covalent bonds: C – C / C – H Hydrophilic Molecules – polar covalent bond containing molecules Diagram displays the partial charges attracting to opposite charges (opposites attract) Dissolved ions are surrounded by three-dimensional hydration shells. Very large polar molecules are not water-soluble, despite having partial charges (and being hydrophilic). Example: Starch A strong Acid would have a high amount of Hydrogen Cations Carbon: Life is carbon based If water is removed from an organism, mainly carbon is whats left All biological molecules are C-based C-based molecules play: 1) structural roles in cells 2) play metabolic roles in cells Carbon Atoms Always Have Four Bonds • Could be four single bonds, two singles and a double, two double bonds, or a single and a triple bond. Carbon also has a moderate to low EN; similar to hydrogen Carbon often plays the back bone in organic molecules C atoms always have four bonds, and are always bonded to at least two other atoms. • C atoms can form single, double and triple bonds: C-based molecules may exhibit isomerism (same formula different structure) Bonds with Carbon are arranged in three dimensions Enantiomers – occur when a C atom has 4 different things attached enantiomers have the same molecular formula but are non-superimposable (can’t overlap when place on top of each other) mirror images of each other Mirror images of each other 8 Sep (F) 4 Large Biological Molecules I Macromolecules – Large biological molecules; they are polymers composed of many monomer subunits Macromolecules are synthesized through condensation synthesis (water is a product) Carbohydrates, proteins and nucleic acids are examples of macromolecules Lipids are also considered large biological molecules, but not as large as or considered as one of the “macromolecules” (due to lipid not being a polymer – doesn’t have monomer subunits) Monomer – “Mono” = one or singular Can exist on their own The building blocks or subunits of polymers (monomers linked together to produce a polymer Polymer – “Poly” = many Polymers consists of many linked monomers; monomers linked by covalent bonds Could be a couple of linked monomers, or might be 100s to 1000s. Example of a Non-biological Polymer: Polystyrene (a common plastic) is a polymer of repeating, linked monomer styrene units Condensation Reaction – The formation of a covalent bond through the loss of a water molecule (reaction produces a water molecule as a byproduct) Condensation is what links together monomers to construct a polymer Many rounds of condensation reactions occur to synthesize a “larger” biological molecule (more rounds = larger molecule/macromolecule) Hydrolysis – The reverse/opposite reaction of condensation; breaks apart a bond through the addition of water (uses up a water molecule, water as a reactant) The process adds a water molecule back in to break a bond Breaks down the polymer and release the monomers Hydrolysis is done to remove damaged or no longer needed macromolecules: Monomer subunits can be recycled to produce new macromolecules Condensation and Hydrolysis are tightly-regulated at the cellular level, if it wasn’t regulated it would result in chaos (probably die) Four major groups of large biological molecules/macromolecules Carbohydrates – monosaccharides and small polymers of carbohydrates are also classified as a carbohydrate (example glucose) Carbohydrate monomers are classified as monosaccharides (simple or singular sugars – glucose, galactose, fructose) Proteins Polymers of amino acids (amino acid – monomer subunits) Lipids – Still large biological molecules, but not classified as a macromolecule (was considered a macromolecule, but demoted like the planet Pluto) Not a macromolecule due to it not being composed of monomer subunits Nucleic Acids – the polymers of nucleotides (thymine, guanine, adenosine, cytosine and uracil - rna DNA and RNA Monosaccharides – Carbon based, primarily has 5-6 carbon atoms as the “backbone”, but may contain 3-7 C atoms Highly water soluble (small polar molecules) Contains 2 or more hydroxyl groups They exist in equilibrium between linear and ring forms when ring forms; side chain it closes on is locked into an alpha or beta position Oligosaccharides – Short chain of 2 or more sugar monomers (not considered as a large biological molecule) Oligo = few (can go up to 16-18 linked monosaccharides) Chain formed via condensation synthesis Disaccharides – are a classification of an oligosaccharide (“di” meaning two sugars) Examples: Sucrose (glucose-fructose), Maltose (Glucose-Glucose), Lactose (glucose galactose) Oligosaccharides are short chains of 2 or more sugar monomers, formed by condensation synthesis. Disaccharides like sucrose have 2 subunits. Oligosaccharides can include longer chains up to 16-18 monosaccharide monomers. Shorter chains are very water-soluble, while longer chains often attach to proteins or lipids. Polysaccharides are large macromolecules with hundreds to thousands of linked monomer subunits formed through condensation synthesis. They are poorly water-soluble but hydrophilic, attracting water. Glucose-based polysaccharides include starch, glycogen, and cellulose. Starch and glycogen contain α-1,4 bonds amylose is a straight-chain starch with exclusively α-1,4 bonds. Amylopectin and glycogen contain α-1,6 bonds Glycogen is more highly branched than amylopectin and serves as the primary energy reserve carbohydrate in mammals. Cellulose is a glucose polymer with β-1,4 bonds and is a crucial part of plant cell walls. Unlike α-1,4 bonds in starch and glycogen, the human digestive system cannot break β-1,4 bonds (can’t break it, so humans can’t access the glucose of cellulose dude to beta 1,4 bonds). Therefore, cellulose cannot serve as an energy source for humans but instead is a component of dietary fiber, known as "insoluble fiber," in human diets. Lipids are large biological molecules that are typically not soluble in water due to their abundant C-C and C-H bonds (which are non-polar covalent bonds), which create non-polar covalent bonds. Lipid subgroups include: Fats (including oils) - hydrophobic Phospholipids - amphipathic (having both hydrophobic and hydrophilic parts) Steroids - hydrophobic Fats, also known as triglycerides or triacylglycerols, consist of three fatty acids covalently attached to a glycerol (3 carbon backbone). They are extremely hydrophobic and do not dissolve in water but can dissolve in less polar solvents like acetone. Fats are primarily used as energy storage molecules and have a higher energy density compared to carbohydrates. Three condensation reactions • Three molecules of water are released in the process. Saturated fatty acids have only single bonds (C-C) in their hydrocarbon chains, while unsaturated fatty acids have one or more double bonds (C=C). These double bonds can be in either cis or trans configurations. In the cis configuration, both hydrogens are on the same side of the hydrocarbon chain, causing a kink in the chain. In the trans configuration, the hydrogens are on opposite sides. Fatty acids can be categorized as: Saturated fatty acids Unsaturated fatty acids Unsaturated fatty acids can further be classified as: Monounsaturated (1 C=C double bond) Polyunsaturated (2 or 3 C=C double bonds) The degree of unsaturation impacts the fluidity of fats. C=C double bonds in the cis orientation introduce kinks or bends into the fatty acid chain, promoting fluidity. This makes it more challenging for fat molecules to closely pack together, which is necessary for solidification. Therefore: Saturated fats (predominantly saturated fatty acids) are solid at room temperature. Examples: Butter, Cholesterol, lard, Polyunsaturated fats (containing polyunsaturated fatty acids) are liquid at room temperature. Examples: Oils (Seed Oils; canola oil, olive oil, vegetable oil/general cooking oils), omega 3’s Phospholipids are amphipathic, meaning they have both polar (hydrophilic) and nonpolar (hydrophobic) regions. They are essential components of biological membranes, contributing to their structural integrity rather than serving as an energy storage molecule. A phospholipid consists of a glycerol backbone, two fatty acids, and a hydrophilic head group. The head group contains a charged phosphate group (PO4^3-) and an attached polar or charged molecule. The hydrophilic head group is represented by "R" in diagrams. Phosphate (PO4^3-) plays a significant role in cellular biology. A phospholipid is a molecule with two fatty acids and a modified phosphate group attached to a glycerol backbone. The charged or polar R group is hydrophilic. Top of Form Top of Form The phospholipid bilayer forms the primary structure of cellular membranes. In this bilayer, the hydrophilic head groups of the phospholipids interact with the surrounding aqueous (water) environment on both sides of the membrane, while the hydrophobic tails are nestled in the center of the bilayer away from water. Steroids are hydrophobic lipids not derived from fatty acids. They share a common structure consisting of four carbon-based rings. Different types of steroids have varying atoms attached to this basic structure. Steroids serve two primary functions: Hormonal roles: Many steroid-based hormones, such as estrogen and testosterone, play essential roles in regulating various physiological processes. Structural roles: Steroids are components of biological membranes and influence membrane fluidity, impacting their structural properties. This “steroid” is cholesterol (LMAO) 11 Sep (M) 5 Large Biological Molecules II Proteins serve diverse roles in living organisms: Structural proteins: Proteins like microtubules and collagen provide structural support. Microtubules are part of the cellular cytoskeleton, while collagen is abundant in the extracellular matrix of multicellular animals. Catalytic proteins: Enzymes, which are a subset of proteins, play a crucial role in cellular metabolism by catalyzing various biochemical reactions. Notably, all enzymes are proteins, but not all proteins are enzymes. Transport proteins: Cell membranes contain specific transport proteins that facilitate the movement of molecules across the membrane. These proteins play a critical role in various cellular processes Proteins are macromolecules composed of amino acid monomers. There are 20 protein amino acids, in addition to some nonprotein amino acids. Each protein has a unique linear sequence of amino acids, and this sequence varies among different proteins. Plants can synthesize all 20 protein amino acids, while humans and other mammals cannot and need to obtain essential amino acids from their diet. Essential amino acid requirements may vary slightly among mammal species; due to the varying amount or type produced by the animal, amino acid production in mammals vary Amino acids feature a central chiral carbon to which an amino group, a carboxyl group, a hydrogen atom, and an R group (side chain) are attached. The R group (= side chain) defines the amino acid. Proteins consist of 20 commonly found amino acids, each characterized by a distinct R group (side chain) that defines its chemical properties. *Memorize names of a few but not the structure* Proteins are linked by peptide bonds that form through a condensation (dehydration synthesis) reaction. In this process, the carboxyl group of one amino acid connects to the amino group of the next amino acid, resulting in the release of a water molecule. It's important to note that R groups (side chains) are not involved in the formation of peptide bonds. A protein is the result of many condensation reactions (the more condensation happening = the larger the polymer) Proteins are a linear chain with an -N-C-C- backbone: Proteins exhibit multiple levels of structure: Primary structure (1°): This is the linear sequence of amino acids, resembling an unbranched chain of amino acids. Secondary structure (2°): Proteins can also have secondary structures, such as alpha-helices and beta-sheets (beta-pleated sheets), which arise from interactions between nearby amino acids. These structures are primarily formed through hydrogen bonds between hydrogen and oxygen atoms in different amino acids along the polypeptide chain (backbone). The R groups (side chains) of the amino acids are not directly involved in the formation of α-helices and β-pleated sheets. Tertiary structure (3°): The overall 3D folding of the protein, determined by interactions among more distant amino acids, gives rise to its tertiary structure. These unique interactions are what specifies the function of the protein based on its structure. due to four types of chemical bonds (interactions) between the R groups on different parts of a protein (don’t need to know the four types of bonds). The four interactions are: hydrophobic interactions, ionic bonding, hydrogen bonding, disulfide linkages Quaternary structure (4°): Some proteins consist of multiple polypeptide chains, and their arrangement and interactions form the quaternary structure. All proteins have primary, secondary, and tertiary structure, while quaternary structure is observed in some proteins. All proteins exhibit primary (1°), secondary (2°), and tertiary (3°) structure. Quaternary (4°) structure is only observed in proteins that are composed of two or more subunits (polypeptides) interacting to form a functional protein. Proteins can exhibit quaternary (4°) structure when they are composed of two or more polypeptide subunits. A polypeptide is a chain of amino acids, and for many proteins, a single polypeptide chain serves as the functional protein. However, in some cases, two or more polypeptide chains interact to form the functional protein, and this arrangement is referred to as quaternary structure. Quaternary structure represents the three-dimensional arrangement of these subunits and describes how they interact with each other in space. Each polypeptide subunit within a protein with quaternary structure also possesses its own primary (1°), secondary (2°), and tertiary (3°) structure. Example: Hemoglobin, the oxygen-carrying molecule in red blood cells, possesses quaternary (4°) structure. It consists of four distinct polypeptides, with two being of one type (shown as red) and the other two being of a different type (shown as blue). Some proteins can have numerous polypeptide subunits, such as the photosynthetic enzyme Rubisco, which contains 16 subunits of two different types. A single amino acid alteration or substitution within a protein can have a profound impact on its structure and function. Example: Sickle Cell Anemia, where a single amino acid change in the beta subunit of hemoglobin results in a different shape for hemoglobin, causing distortion in red blood cells and the potential to block small blood vessels, leading to various health complications. In normal hemoglobin (which consist of 147 amino acid residues in length) a single substitution can have significant impact, the amino acid at position seven is glutamate, whereas in sickle cell hemoglobin, it is replaced by valine. This minor change leads to the structural alteration of hemoglobin and the development of sickle cell anemia. Protein terminology Polypeptide: Definition #1: A subunit of a protein that has quaternary (4°) structure when multiple subunits are present (protein composed of 2 or more subunits). Definition #2: Also used to refer to a string of 10 or more linked amino acids, typically a signaling molecule in an organism. Smaller than the subunit of a protein (as per definition #1). Peptide: A chain consisting of 2 to 10 linked amino acids, often functioning as a signaling molecule (e.g., peptide hormones). Smaller than the definition #2 of polypeptide. Oligopeptide: A chain of 10 or more linked amino acids, typically serving as a signaling molecule in an organism. Equivalent to definition #2 of polypeptide. The term "oligo" means few, emphasizing the relatively small size of the molecule The native conformation of a protein refers to its three-dimensional shape in its functional state. It encompasses the sum of secondary (2°), tertiary (3°), and quaternary (4°, if present) structures but does not include the linear primary (1°) structure of the protein. Proteins can adopt other conformations besides their native one, but these alternative conformations are not considered the native conformation. The native conformation of a protein relies on it being in the correct environment, including factors such as pH, metal ion concentration, and whether it's in a water or lipid-based environment. Changing these environmental conditions can lead to alterations in the protein's conformation, a process known as protein denaturation. Depending on the specific circumstances, denaturation may or may not be reversible, meaning the protein can or cannot return to its native conformation. Protein Denaturation: A change in conformation away from the native conformation (results in the protein functioning differently or losing function) Depending on the situation, some protein denaturation may or may not be reversible if returned back into its correct environment. Example: High temperatures (cooking) typically results in irreversible protein denaturation Nucleic acids are essential molecules that store genetic information and perform various roles related to information processing. They are polymers, true macromolecules, and their monomer subunits are nucleotides. There are two primary types of nucleic acids: RNA (ribonucleic acid): RNA includes several types that serve various functions, such as: Messenger RNA (mRNA): Acts as information intermediates. (transcription) Ribosomal RNA (rRNA): Plays a structural role in ribosomes, the protein-building machinery of cells. Transfer RNA (tRNA): Manages amino acids for protein synthesis. Several other types that bio 100 isn’t going to cover DNA (deoxyribonucleic acid): DNA represents genetic information, and genes are sequences of DNA that carry instructions for various cellular processes. There is only one type of DNA. Nucleic acids are crucial for storing, transmitting, and utilizing genetic information in living organisms. Genes serve as repositories of genetic information. The information contained in a gene can represent one of two main things: The primary (1°) structure, which refers to the amino acid sequence of proteins or polypeptides. This is the most common type of information stored in genes. The nucleotide sequence of RNA, which can include various types of RNA such as ribosomal RNA (rRNA) or transfer RNA (tRNA). In the context of genes specifying the primary structure of proteins, there is a flow of information: DNA in the gene carries the genetic code. This genetic code is transcribed into RNA (mRNA) through a process called transcription. The mRNA then serves as a template for protein synthesis in a process called translation. During translation, the sequence of nucleotides in mRNA is translated into the sequence of amino acids in the protein. Ultimately, this sequence of amino acids determines the primary structure of the protein, which is essential for its function. This flow of information, from DNA to RNA to protein, is a fundamental aspect of genetic expression and is central to the functioning of living organisms. A nucleotide consists of three essential components: A phosphate group. A 5-carbon (5C) sugar. A nitrogenous base. These three components together form the building blocks of nucleic acids like DNA and RNA. RNA and DNA nucleotides have some key differences: Sugar Component: RNA nucleotides contain a 5-carbon sugar called ribose. DNA nucleotides contain a related 5-carbon sugar called deoxyribose. The "deoxy" prefix indicates the absence of an oxygen atom at a specific position compared to ribose. Nitrogenous Bases: RNA nucleotides can have the nitrogenous bases Adenine (A), Uracil (U), Cytosine (C), and Guanine (G). DNA nucleotides can have the nitrogenous bases Adenine (A), Thymine (T), Cytosine (C), and Guanine (G). Thymine replaces Uracil in DNA. These differences in sugar and nitrogenous bases between RNA and DNA nucleotides are fundamental to their distinct roles and functions in cellular processes. Pyrimidines have one C-based ring; C, T, U. Purines have two C-based rings; A, G. Nucleic acid nucleotides are connected by bonds formed between the phosphate group of one nucleotide and the 5-carbon (5C) sugar of the adjacent nucleotide. The nitrogenous bases, such as adenine (A), thymine (T), cytosine (C), guanine (G), uracil (U) in RNA, do not directly participate in these bonds. The arrangement of phosphate and sugar groups creates an alternating sugar-phosphate backbone in the nucleic acid chain, providing structural stability to the molecule. This backbone is a key feature of the double helix structure in DNA, where the nitrogenous bases are positioned in the interior, forming base pairs. Hydrogen bonding happening between base pairs A = T / G ≡ C DNA is indeed organized into a double helix structure, which consists of two helical strands that are aligned in an antiparallel fashion, meaning they run in opposite directions (5’ 3’ or 3’ 5’). The nitrogenous bases, including adenine (A), thymine (T), cytosine (C), and guanine (G), are positioned inwards, facing each other. Hydrogen bonds form between complementary nitrogenous bases on opposite strands. Specifically, adenine pairs with thymine, and cytosine pairs with guanine. These hydrogen bonds are critical for the stability of the double helix and the specific base pairing in DNA. The DNA strands in the double helix are complementary, meaning if you know the sequence of one side/strand, by default the complementary/opposite strand is known. RNA (Ribonucleic Acid) Single-Stranded Structure: RNA molecules are typically single-stranded, although exceptions exist. Various Types of RNA: There are different types of RNA, with three main categories we will focus on: mRNA (messenger RNA), rRNA (ribosomal RNA), and tRNA (transfer RNA). Nitrogenous Bases: RNA uses the same four nitrogenous bases as DNA: Adenine (A), Cytosine (C), Guanine (G), and Uracil (U). Uracil replaces Thymine (T) found in DNA. Transcription: RNA is synthesized through a process called transcription, during which a DNA template is used to assemble the RNA molecule. The RNA sequence is complementary to the DNA sequence it is transcribed from. The rules for base pairing in RNA are the same as in DNA, with the exception that Uracil (U) replaces Thymine (T). So, in RNA, Adenine (A) pairs with Uracil (U), and Cytosine (C) pairs with Guanine (G). This complementary base pairing ensures the accurate transcription of genetic information from DNA to RNA. Example: The rna is mRNA and is complementary to the DNA 13 Sep (W) 6 Cell Structure I Two Broad Classes of Cells Prokaryotic – lacks a nucleus and membrane-delimited organelles Examples of Prokaryotes: Bacteria and Archaea (single celled organism - unicellular) Eukaryotic – have a nucleus and membrane-delimited organelles Examples of Eukaryotes: plants, fungi, animals, protists (a mostly unicellular organism) Microscopes, beginning in the 1600s, revealed the fundamental role of cells in biology. Advances in light microscopes and the introduction of electron microscopes in the 1950s provided increasingly detailed cell views. Early microscopes identified cellular compartments, referred to as "cellulae" (Latin for "small cells"). Initially, the universal significance of cells in biology was not fully recognized. Before cell biology was coined, the old idea of spontaneous generation was recognized The idea that living organism could be generated from non-living matter. Thought that many organisms could be generated without parental organisms Eventually became apparent that organisms come from organisms, cells come from cells and spontaneous generation is an impossibility Cell Theory – an example of inductive reasoning Many scientist that observed and were interested in cells made three generalizations All organisms are composed of 1 or more cells The cell is the basic living unit of organization All cells arise from pre-existing cells Generalities about Cells: All cells are surrounded by a membrane All cells contain dna There are two fundamentally different types of cells: eukaryotic and prokaryotic Cells are considered to be the fundamental unit of biological organizational; they are the lowest level of structure capable of performing all of the activities of life It’s possible to culture cells of multicellular organisms, but not possible to culture anything smaller than a cell. Prokaryotic cells have been around longer than eukaryotic (eukaryotic is thought to be evolved from prokaryotic) Eukaryotic cells have a nucleus and are compartmentalized = sub-divided; different parts of the cell are specialized for various functions Domain Eukarya includes: protists (algae, amoebae) plants, fungi and animals The first eukaryotes were protists Eukaryotes versus Prokaryotes • In general, eukaryotic cells are larger than prokaryotic cells. • Eukaryotes have lots of internal membranes, that enclose specialized spaces (that have specialized metabolic functions) Organelle definitions – different sources have different definitions • Definition #1: Membrane-delimited subcellular compartments. • Definition #2: Any subcellular structure (membrane not required) Ribosomes – not membrane bound, but are classified as an organelle Both eukaryotes and prokaryotes have ribosomes According to definition one its not an organelle, but definition 2 says it is In essence, eukaryotes have membrane-delimited subcellular organelles and prokaryotes do not. Membranes have a boundary functions The plasma membrane controls what goes in and out of the cell Some organelles have a double membrane (nucleus has 2, the nuclear envelope consist of an inner and outer layer) Terminology – Cytosol vs Cytoplasm Both terms often used interchangeably, but are actually not interchangeable Cytosol is the water-based gel like fluid that surrounds organelles Cytoplasm = all of the material to the inside of the plasma membrane, except for the nucleus; includes the cytosol and ribosomes Nucleus – delimited by double membrane (nuclear envelope) Has protein lined pores that allow traffic of molecules into and out of the nucleus Nucleus contains the majority of DNA in a cell; mitochondria also have some DNA, as do chloroplasts (plants) DNA is also present as part of chromatin which is a DNA-protein complex DNA represents information; the protein in the chromatin plays a structural role; the chromatin is organized into chromosomes Nucleolus is part of the nucleus specialized for ribosomal subunit synthesis (involved in transcribing DNA) Majority of the time – chromosomes are present in the non condensed state; they are tangled up with each other in the nucleus; only during cell division do the chromosomes condense and become distinct DNA stays in the nucleus, but the information represented by DNA is needed in the cytosol and mRNA is the molecule that transfers this information to the cytosol mRNA pass through the pores of nuclear envelope and other types of RNA also passes through the pores Ribosomes – subcellular structures found on both prokaryote and eukaryotes Is not membrane delimited Found in the cytosol of eukaryotic cells In eukaryotes – ribosomes may be free in the cytosol or attached to the cytosolic side of the endoplasmic reticulum (also known as rough ER) Ribosomes can also be found in mitochondria and chloroplasts Ribosomes are the protein building machines Composed of small subunit and large subunit. Both subunits being composed of a combinations of rRNA and ribosome specific proteins A functional ribosome contains both subunits and a piece of mRNA The ribosomal subunits are assembled in the nucleus and individuals leave through the pores of the nucleus Ribosomes use mRNA to construct proteins by reading and following the specified amino acid sequence. (exhibits structure 1) Most organelles, including the plasma membrane, are connected by membrane flow, allowing membranes to move between organelles and to/from the plasma membrane. These membranes are fluid, not solid or rigid. The endomembrane system is the group of membranes and organelles that work together to modify, package, and transport lipids and proteins The endomembrane system includes: 1) nuclear envelope 2) endoplasmic reticulum (ER) = the source of membrane for the system 3) Golgi bodies (Golgi apparatus) 4) lysosomes 5) plasma membrane (not really an “endo” membrane; because “endo” = “inside”) 6) various small vesicles/vacuoles Not part of the endomembrane system: 1) mitochondria 2) peroxisomes 3) chloroplasts Endoplasmic Reticulum The endoplasmic reticulum (ER) is a continuous membrane system in the cytosol, serving as the primary site for synthesizing membranes within the endomembrane system. It is also connected to the nuclear envelope and contains an interior space known as the lumen or cisternal space, a term commonly used in biology to denote an inner cavity (derived from Latin). ER without attached ribosomes is termed smooth ER, characterized by its tubular structure. The smooth ER is involved in membrane synthesis and carries out diverse functions, which can differ between cell types. For instance, in liver cells, ER tubules house detoxification enzymes. Remarkably, over half of the membranes within the endomembrane system are found in the ER. Golgi bodies consist of flattened sacs enclosed by membranes, making them membrane-delimited organelles. Typically, a single series of these sacs is referred to as a Golgi body, while the collection of all Golgi bodies within a cell, which is usually multiple, is known as the Golgi apparatus. Two main functions (summary) of the Golgi apparatus: 1) protein modification 2) protein sorting (sending proteins to their final destination) shipping side • Golgi bodies have directionality: there is receiving side and a shipping side. *draw diagrams for this* Proteins synthesized on rough ER enter the ER's lumen. From there, they are enclosed in transport vesicles composed of fluid membranes. These vesicles move to the Golgi apparatus, where they merge, releasing the protein into the Golgi. The protein traverses the Golgi, undergoing modifications such as the addition of oligosaccharide side chains. Subsequently, the Golgi repackages the protein into another vesicle, which carries it to its ultimate destination. The destinations of the packaged proteins from the Golgi apparatus can include the lysosome or be sent outside of the cell Membrane Flow path Amoebas are microscopic, single-celled organisms that feed through phagocytosis, which involves engulfing external particles. This process exemplifies membrane flow as it leads to the formation of a food vesicle (food vacuole) with a membrane originating from the plasma membrane. The food vesicle travels to the lysosome where it fuses with the lysosome and the food dumped into the lysosome is altered by a digestive enzyme (digestive enzyme received from the Golgi apparatus) Macrophages, immune system components, combat foreign particles, such as bacteria, by engulfing them via phagocytosis. This process involves the formation of a vesicle that subsequently fuses with a lysosome. Inside the lysosome, enzymes (discussed later) digest the engulfed particle Organelles not Part of the Endomembrane system: Peroxisomes are small, single-membrane-bound organelles with diverse functions that can vary between tissues, including roles in fat breakdown and alcohol detoxification in the liver. Mitochondria, both in singular (mitochondrion) and plural, are pivotal in cellular energy metabolism. Cells may contain hundreds of mitochondria. They house essential processes, including the citric acid cycle (also known as Kreb's or TCA cycle), the respiratory electron transport chain, and ATP synthase. Mitochondria possess a double membrane, with the outer membrane featuring proteins called porins that create pores, allowing specific molecules to diffuse through. The mitochondrial inner membrane lacks porins but has specialized transport proteins for precise molecule transport. It's extensively folded, forming structures known as cristae. The matrix, akin to the cytosol of the mitochondrion, is a water-based gel-like fluid where numerous biochemical processes occur. The region between the inner and outer membranes is called the intermembrane space (IMS). Additionally, mitochondria contain their own DNA and ribosomes. Chloroplasts are where photosynthesis occurs in eukaryotes. They are absent in animals. These organelles consist of a double membrane, known as the chloroplast envelope, and an internal membrane system called thylakoids. Some thylakoids are stacked into structures called grana, connected by stromal thylakoids. The stroma is a water-based gel-like fluid with significant biochemical activity. Photosynthetic plant cells usually contain multiple chloroplasts. Chloroplasts also possess their own DNA and ribosomes. The cytoskeleton is a non-membrane-bound network of fiber-like proteins that traverse both the cell and the nucleus. In animal cells, it comprises three primary types of fibers: microtubules, microfilaments, and intermediate filaments (which are absent in plants). The cytoskeleton serves several essential functions, including: providing mechanical support for cell shape maintenance, anchoring and mobilizing organelles, and facilitating cell motility. Microtubules play a role in motility through cilia and flagella: Cilia are typically short and numerous, while flagella are long and few (usually 1-4 per cell). Although cilia and flagella extend from the cell surface, they are considered intracellular because they are enclosed by the plasma membrane. Cilia are mainly used to move substances across the cell's surface, whereas flagella are primarily involved in moving the entire cell. The extracellular matrix (ECM), also known as the glycocalyx, is a thin outer layer present on animal cells, located outside the plasma membrane. It is notably less rigid than the cell walls found in plants and fungi, and it forms a hydrated, gel-like system with a high-water content. ECM consists of a network of proteins and carbohydrates Proteoglycan is a combination of a protein backbone, with lots of carbohydrates attached (super hydrophilic component) ECM is the Shock absorber for cell. Has important roles in cell migration, signalling, tissue development, cell to cell adhesion and communication Collagen is a protein and makes up approximately 40% of all the proteins found in animals, particularly in the extracellular matrix. It forms a network with glycoproteins, known as proteoglycans, where "glycol" refers to sugar. Proteoglycan is a combination of sugar and protein. Other proteins involved include fibronectin and integrin. This entire complex is hydrophilic and saturated with water. Summary • Cells are the fundamental unit of organization in biology. • Eukaryotic cells have a nucleus and other membrane-delimited organelles. • The nuclear envelope has pores, and there is a lot of traffic of molecules through the pores. • Membranes have a boundary function: inside versus outside. • There is an endomembrane system, with membrane flow between components of the system. • Not all organelles are part of the endomembrane system. • Eukaryotic cells are compartmentalized; different parts of the cell are specialized for different purposes • Ribosomes are protein-building machines, and are largely cytosolic (free, and associated with rough ER), with some ribosomes also found in mitochondria and chloroplasts 18 Sep (M) 8 Membranes I Plasma Membrane – Fluid Mosaic Model: The membrane is composed of the phospholipid bilayer The bilayer is a fluid Water is present on both sides of the membrane Basically the membrane is a fluid that separates a fluid Bilayer: Consists of Hydrophilic heads and hydrophobic ends/tails Fluid Mosaic Model of Biological Membranes The fluid refers to the phospholipid bilayer of the membrane Phospholipids are able to diffuse from one part of the membrane to the other Mosaic refers to the protein associated/on the membrane, some of the proteins on the membrane slowly move around and change locations over time Frye Edidin experiment – showed that proteins move around the PM Done through labelling proteins and allowing them to intermingle and gradually diffuse with a fused cell Experiment showed that proteins intermingle and move rather than being locked to their area of the membrane The phospholipid bilayer is able to Rotationally diffuse (rotate in place) Transversal diffusion (membrane flip flops, hydrophilic heads must move through the hydrophobic tails – very rare to happen) Lateral diffusion (moving side to side – very common movement of the PB) Phospholipids both have hydrophobic and hydrophilic parts making them amphipathic Their amphipathic nature is what constitutes the phospholipid bilayer, which forms the fabric of the membrane Note that there is lots of water on both sides of membrane The kink in the hydrophobic unsaturated tails combined with the saturated fatty acid is what gives the membrane its fluidity and structure Biological membranes contain proteins May be integral/intrinsic or peripheral/extrinsic Integral proteins – imbedded or wedged into the phospholipid bilayer – harder to move (some are anchored in place by cytoskeleton) Integral proteins in the bilayer have hydrophobic amino acids and hydrophilic amino acids interacting with the respective regions – which is why they are able to stay wedged into the bilayer (prevents integral proteins from popping out of the membrane) Peripheral proteins – not embedded into the phospholipid bilayer (chills outside of the bilayer) or it can be loosely embedded Easier to remove from membranes compared to integral proteins Held in place via interactions with the phospholipids or with the integral proteins Typically outside of the PM Membranes are inherently fluid, and this fluidity is crucial for their proper function. Significant temperature fluctuations can disrupt membrane fluidity, leading to increased permeability and potentially cell death. In animal cell membranes, cholesterol plays a complex role in modulating fluidity. It enhances fluidity at low temperatures and reduces it at high temperatures, helping to maintain membrane stability. Proteins in the membrane have various types and functions Hormone receptors Enzymes Transport proteins Transport proteins are integral membrane proteins that span the membrane. They exhibit substrate-specificity, meaning they transport specific molecules and are not generalized transporters. There are two main classes of transport proteins: Carrier proteins. Channel proteins, which encompass aquaporins, specialized channels for water transport. Molecules move across membranes through various mechanisms: Transport proteins facilitate the process. Simple diffusion occurs without transport proteins. Endocytosis and exocytosis involve the engulfing and release of molecules via membrane vesicles. Different types of molecules cross membranes using various mechanisms: Gases: Oxygen (O2) and carbon dioxide (CO2) are biologically relevant gases. They are both small molecules and non-polar, allowing them to diffuse through the lipid bilayer. Water: Despite being polar, water molecules can cross membranes due to their small size and high abundance. Ions and Small Polar Molecules: These are repelled by the hydrophobic core of the phospholipid bilayer. Examples include glucose and many amino acids, which require specialized transport systems to cross the membrane. Large Molecules: Some large molecules can also cross membranes, but they typically rely on specialized transport systems for this purpose. Water and the gases can cross membranes via simple diffusion—a form of passive transport Biological membranes are selectively permeable (or semipermeable), meaning not all molecules easily cross them. Polar or charged molecules, such as ions, cross membranes very slowly in the absence of specific transport proteins. However, given enough time, nearly anything can cross a membrane, so it's more accurate to describe biological membranes as differentially permeable. Mechanisms for the Movement of Molecules Across Membranes include: Diffusion: a) Simple diffusion, b) Facilitated diffusion, which involves i) channel proteins and ii) carrier proteins. Active Transport: Also known as primary active transport. Co-Transport: Also known as secondary active transport. Exo- and Endocytosis: Processes involving the engulfing and releasing of molecules via membrane vesicles Simple diffusion also happens in the absence of a membrane Diffusion is the result of random molecular motion driven by kinetic energy. It can occur in both liquid and gas phases, but we'll focus on the liquid phase, particularly water as the solvent within cells. In diffusion, both solutes (dissolved substances) and solvents (the dissolving substances, usually water in cellular contexts) exhibit movement. Solutes diffuse from regions of higher concentration to lower concentration, following a solute concentration gradient (ΔC). However, the diffusion of water occurs in the opposite direction: from areas of lower solute concentration to higher solute concentration. This creates a balance where water and solutes diffuse in opposite directions. “Δ” always means a comparison, change or gradient. (Difference in) The diffusion of solutes involves the movement from areas of higher concentration to lower concentration, following a solute concentration gradient (ΔC). When equilibrium is reached, ΔC equals zero, indicating a uniform concentration of solute throughout the system. At this point, there is no net movement of solutes, but individual solute and solvent molecules continue to move, colliding and bouncing off each other within the system. Water, like other solvents, also undergoes diffusion. However, it diffuses in the opposite direction to the diffusion of solutes, moving from areas of lower solute concentration to higher solute concentration. This phenomenon is known as osmosis and represents a specialized case of water diffusion across selectively permeable membranes. Water can diffuse across biological membranes through two mechanisms: Water is a small molecule, so despite being polar, some water molecules can diffuse through the hydrophobic core of a phospholipid bilayer. Aquaporins are membrane-spanning (integral) channel proteins that serve as water pores, allowing water to bypass the hydrophobic core of the membrane and facilitate its rapid transport. (passive facilitated transport) Hypertonic Solution – due to the higher solute concentration outside, water moves out and the cell is dehydrated causing it to shrivel up Isotonic Solution – Solute concentrations are equal inside and outside the cell (best conditions for cells) Hypotonic Solution – higher solute concentration is higher inside the cell, so therefore water diffuses in the cell putting the cell in risk of bursting (excess water may cause the cell to burst) Simple diffusion is not effective for polar molecules or ions because they have hydration shells and are repelled by the hydrophobic interior of membranes. In these cases, the presence of a concentration gradient (ΔC) alone is insufficient for effective diffusion. Instead; specialized transport proteins are required, a process known as facilitated diffusion. There are two main types of facilitated diffusion proteins: channel proteins carrier proteins which aid in the transport of polar molecules and ions across membranes. Channel proteins exhibit specificity its selective channels can be opened or closed (allow diffusion as needed) Down a concentration gradient (diffuses of solutes always happens from higher to lower) Carrier proteins, also known as uniporters, play a crucial role in facilitating the movement of specific molecules across cell membranes: Change in Shape: Carrier proteins change their shape during the transport process. Substrate Binding Site: These proteins have a substrate binding site that exhibits specificity for the transported molecule. Conformational Changes: When a substrate binds, it induces a conformational change in the carrier protein. This change allows the substrate to be released on the other side of the membrane. Direction of Transport: Carrier proteins enable the movement of molecules down their concentration gradient (ΔC), meaning the diffusion of solutes through carrier proteins always occurs from areas of higher concentration to lower concentration. Diffusion of solutes always occurs down a concentration gradient (ΔC). Simple diffusion doesn't involve a transport protein. Facilitated diffusion, on the other hand, relies on transport proteins, such as channel proteins and carrier proteins (uniporters). There are no general transport proteins; specificity is key. At equilibrium, ΔC = 0, but there is still molecular movement, although no net movement. Water: Water diffusion takes place from areas of lower solute concentration to higher solute concentration. Water diffusion can occur through two mechanisms: 1) simple diffusion and 2) facilitated diffusion, facilitated by aquaporins, which are a type of channel protein. Transporting molecules against their concentration gradient (ΔC) is not a form of diffusion. It requires the expenditure of cellular energy (ATP), essentially performing cellular work. In this process, a transport protein is essential, and these proteins have substrate binding sites, ensuring specificity. There are two main systems for transporting molecules against ΔC: Active Transport (Primary Active Transport): This process directly utilizes ATP as an energy source. Co-Transport (Secondary Active Transport): It indirectly utilizes ATP, often by using the energy created from primary active transport processes. ATP – the main energy currency of the cell, the energy is accessed through a hydrolysis reaction where a phosphate group is removed ATP + water ADP + P diffusion of solutes naturally occurs down a concentration gradient (from areas of higher concentration to lower concentration) without the need for energy input, much like riding a bicycle downhill where gravity does the work. In contrast, active transport and co-transport systems move solutes against their concentration gradient (from areas of lower concentration to higher concentration), and this process demands energy input, similar to the effort required to ride a bicycle uphill against gravity. ATPases – Enzymes/proteins that hydrolyze or spend ATP Active transport is executed by specialized transport proteins known as ion pumps or, more precisely, cation-translocating ATPases. These pumps actively move cations (positively charged ions) across a membrane against their concentration gradient, requiring the expenditure of ATP. Moving anything against a gradient, whether ions or other molecules, demands energy input. In many cases, active transport results in a net export of positive charge, although some ion pumps are considered "electroneutral," meaning they do not cause a net change in charge across the membrane. It's important to note that there are no ATPases responsible for actively transporting anions (negatively charged ions) or non-charged molecules. Cutoff 22 Sep (F) 10 Metabolism & Bioenergetics I Spontaneous Work VS Non-Spontaneous Spontaneous Process/Reaction – A process or reaction that can maintain itself without energy once started Non-Spontaneous Process/Reaction – A process or reaction that can’t maintain itself without energy once started