BIOL2301 Lecture 02 - Biological Molecules PDF
Document Details
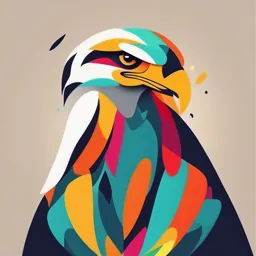
Uploaded by AffordableModernism361
University of Houston
Tags
Summary
This document is a lecture on biological molecules, covering their structures, functions, and properties. It details various classes of molecules including lipids, proteins, carbohydrates, and nucleic acids.
Full Transcript
Biological molecules Create the molecular players, structures & organelles of the cell Distinct structures Distinct biochemical properties Most are ma...
Biological molecules Create the molecular players, structures & organelles of the cell Distinct structures Distinct biochemical properties Most are made up of smaller repeating subunits called monomers Polymers: nucleic acids, proteins, & carbohydrates Not a polymer: lipids Condensation & hydrolysis reactions Polymers are molecules that are made up of smaller repeating subunits called monomers. Polymers are formed from monomers in condensation/dehydration reactions Monomers are joined together by covalent bonds Water is removed and energy is added Polymers are broken into monomers in hydrolysis reactions Covalent bonds are broken and energy is released hydro, “water” +lysis “break”; a water is broken and the H+ & OH- are added to the two “broken” ends Lipids Diverse group of fatty, water-insoluble compounds Contain C, H, O (like carbohydrates), but have much fewer O. Function as Storage of nutrients Components of cellular membranes Hormones Four classes Acylglycerides (neutral fats) Phospholipids Steroids waxes Lipids: Triacylglycerides Known as fats (solid) and oils (liquid) Composed of glycerol (an alcohol) and three fatty acids (hydrocarbon) Glycerol backbone is always the same Fatty acids can vary in length (14-20 carbons) Variable in the number of double bonds Saturated = no double bonds Unsaturated = one double bond Polyunsaturated = two or more double bonds Double bonds prevent fatty acid chains from aligning close together The more unsaturated chains, the more liquid the fat Lipids: Triacylglycerides Functions Used as long-term energy storage in adipose tissue Structural support Cushions Insulation Lipogenesis – condensation reaction to form triglycerides from glyceride and fatty acids Lipolysis – hydrolysis reaction breaking a triglyceride into the glycerol and the free fatty acids Lipids: Phospholipids Modified triglycerides Polar (hydrophilic) head: diglyceride with a phosphorous-containing group Nonpolar (hydrophobic) tail: 2 fatty acids chains Amphipathic In an aqueous environment, phospholipids arrange themselves in such a way that the hydrophobic tails are hidden from water and the hydrophilic heads are able to interact with water. Form a lipid bilayer Primary component of the cell membranes Lipids: Steroids Flat molecules consisting of four interlocking hydrocarbon rings Cholesterol is the basic/backbone for all steroids Synthesized in the liver Also absorbed from diet from milk, eggs, and animal fats Found in cell membranes Necessary for hormones & Vitamin D production Lipids: Eicosanoids Diverse family of 20-carbon fatty acids derived from arachidonic acid Four classes Prostaglandins Prostacyclins Thromboxanes Leukotrienes Functionally are signaling molecules Inflammation Blood clotting Labor contractions Lipids: Waxes Composed of fatty acid & long- chain alcohol Condensation reaction to form ester bond Ester bond Used to provide protective barrier Cerumen Ester bond fatty acid chain long-chain alcohol Carbohydrates Simple sugars and sugar polymers Classified according to size Monosaccharides: simple sugars Disaccharides: two simple sugars linked by covalent bonds Polysaccharides: many monosaccharides Contain C, H, O Monosaccharides have a fixed ratio (CH2O)n Most have 3 to 7 carbons Polymerization due to condensation reactions Carbohydrates Functions To provide a ready, easy source of cellular “food” (energy) Monosaccharides and disaccharides are metabolized to release energy (ATP) Monomers can be stored as polymer Glucoses can be linked together to form long polymers called glycogen Serves as the backbone of genetic material Tagging other biomolecules for identification purposes Glycosylation refers to tagging of biomolecules with carbohydrate chains Nucleic acids Largest molecule in the body Store and transfer genetic information in cells Two major classes DNA (deoxyribonucleic acid) RNA (ribonucleic acid) Composed of nucleotide monomers. 3 components Covalent linkage (by condensation) is called a phosphodiester bond Components of a Nucleotide A pentose sugar Difference in the functional group at 2- carbon RNA has –OH DNA has -H A phosphate group Attached to carbon-5 A nitrogenous base Single-ringed, Pyrimidines Cytosine, Thymine (DNA), Uracil (RNA) Double-ringed, Purines Adenine, Guanine RNA –OH DNA –H ATP: the battery of the cell Molecule that “stores” chemical energy for cellular purposes Structure: Adenine bound to Ribose sugar with 3 phosphate groups Energy is stored in the bonds between the phosphate groups Very unstable molecule because the phosphate groups repel one another DNA Long, double stranded polymer A double chain of nucleotides The “backbone” of DNA (and RNA) consists of the sugars and phosphate groups, bonded by phosphodiester linkages. The phosphate groups link carbon 3′ in one sugar to carbon 5′ in another sugar. The two strands of DNA run in opposite directions (“antiparallel”). Purines pair to pyramidines by hydrogen bonds Paired spiral – “double helix” Complementary pairing of nucleotides A always pairs with T C always pairs with G RNA Single stranded chain of nucleotides U replaces T (found in DNA), otherwise bases are the same Different functional roles, but mostly responsible for protein production issued by the DNA RNA is single-stranded, but complementary base pairing occurs in the structure of some types of RNA. Nucleic acids DNA is an informational molecule: information is encoded in the sequences of bases. All DNA molecules have the same structure— variation is in the sequence of base pairs. DNA carries heritable information between generations. Because double helix is complimentary, each strand serves as a template for the other strand during DNA replication. Daughter cells receive identical copies of the original DNA RNA uses the information stored in DNA to determine the sequence of amino acids in proteins. Central dogma of genetics Encoded in the sequence of DNA is the information that programs all of the cell’s activity (genes) RNA is the specific instruction (information from a single gene) to create a specific protein Proteins are the tools / machinery of cellular function Proteins Basic structural material of the body Contain C, O, H, & N as well as S & P Comprised of long chains of amino acids Play vital roles in cell function Amino Acids Building blocks of proteins Twenty common types Two functional groups Amine group (-NH2) Carboxyl group (-COOH) One variable (R) group (side chain) that makes each amino acid unique Amino acids can be grouped based on side chains. Peptide Bonds The linking of two amino acids between the amine and carboxyl group Condensation reaction The polypeptide chain is like a sentence The “capital letter” is the amino group of the first amino acid—the N terminus Carboxyl Amine The “period” is the carboxyl group of the last amino acid—the C terminus The order (sequence) in which the amino acid are linked provide structure and function to the protein 20 amino acids = “protein alphabet” Proteins = “words” Different proteins are different combinations and numbers of amino acids Chaperone Proteins assist in protein folding Help proteins achieve their functional 3D structure Prevent accidental, premature or incorrect folding and association process Aid the proper or “desired” folding and association process Structure levels of proteins Primary structure: the unique linear sequence of amino acids Similar to the order of letters in a word Structure levels of proteins Secondary structure The polypeptide segments exhibiting structural patterns formed by the sequence of amino acid Dependent on interactions between R groups Tertiary structures is derived from secondary structure Structure levels of proteins: secondary structure The α-helix Right-handed coil resulting from hydrogen bonding Common in fibrous structural proteins Provides elasticity in fibrous proteins The β-pleated sheet Ribbon-like structures Two or more polypeptide chains are aligned Provides flexibility in globular proteins Structure Levels of Proteins Tertiary Structure Sum total of the secondary structures Shape of the whole polypeptide Maintained by: Covalent disulfide bonds Aggregation of hydrophobic side chain Ionic bonds van der Waals forces Hydrogen bonds The outer surfaces present functional groups that can interact with other molecules Structure levels of Proteins Quaternary Structure Aggregate of 2 or more polypeptide chains Results from the interaction of subunits by hydrophobic interactions, van der Waals forces, ionic bonds, and hydrogen bonds. May include prosthetic groups Nonprotein structures covalently bonded to the protein Denaturation Process that causes proteins to unfold and lose their shape pH Common causes are changes in High Temp pH, increases in temperature, and high concentration of polar substances Usually irreversible because amino acids that were initially hidden are now exposed and cause either a new structure or different molecules to bind Why is this important? 3° structure allows physiology function at protein active sites (sites that interact chemically with other molecules) Enzymes Catalysts speed up the rate of a reaction. The catalyst is not altered by the reactions. Ea changes the reactants into unstable forms; effectively lowers Ea The final equilibrium doesn’t change; the amount of energy consumed or released doesn’t change Most biological catalysts are enzymes (proteins) that act as a framework in which reactions can take place. Also RNA enzymes called ribozymes Enzymes Enzymes and ribozymes are highly specific. Some control a single chemical reaction Others are not substrate specific, but regulate related reactions Reactants are called substrates. Substrate molecules bind to the active site of the enzyme. Three-dimensional shape of the enzyme determines its specificity. Enzymes are named for the type of reaction catalyzed Hydroxylase: add H2O during hydrolysis Oxidase: Add Oxygen If it’s an “-ase” it’s an enzyme, but not all enzymes end with “-ase” Mechanism of enzyme actions E+S [ES] [EP] E+P The enzyme-substrate complex is held together by hydrogen bonds, electrical attraction, or covalent bonds. The enzyme may change when bound to the substrate, but returns to its original form. Enzyme active site must bind substrate (E + S) The Enzyme-Substrate complex (ES) undergoes internal rearrangements (termed induced fit) to form product (P) Enzyme releases product of the reaction Mechanism of enzyme actions E+S [ES] [EP] E+P Enzymes and Reaction rates Concentration Increasing either substrate or enzyme can increase the rate of reaction Saturation is the point where the [S] is so high that all enzymes are actively engaged and no further increase in reaction rates can occur Temperature Each enzyme has an optimal temperature range in which it functions Most enzymes in human body are most efficient between 95 and 104°C pH Each enzyme has an optimal pH range in which it functions Changes in pH = denaturation Most enzymes in human body are most efficient between pH of 6 and 8