BIOL 2306 Notes PDF
Document Details
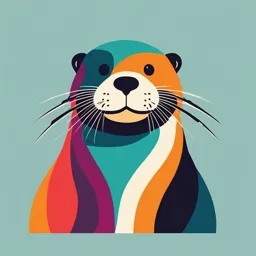
Uploaded by CrispSequence1315
The University of Hong Kong
Tags
Summary
These are lecture notes covering the history of evolution, from theories put forward before Darwin and natural selection, to the Darwin-Wallace theory and the importance of ecology.
Full Transcript
L1 Overview: Evolution & Placing Human in Nature Knowledge systems “Sophisticated & detailed knowledge of the biophysical environment is not confined to science.” Place of humans in nature - theories of life - Homo sapiens: Lived as hunter-gathers - Aristotle: Populations remain stable...
L1 Overview: Evolution & Placing Human in Nature Knowledge systems “Sophisticated & detailed knowledge of the biophysical environment is not confined to science.” Place of humans in nature - theories of life - Homo sapiens: Lived as hunter-gathers - Aristotle: Populations remain stable aside from occasional plagues of pests (e.g. locus) caused by God. → Natural harmony and the ‘balance of nature’ were maintained on Earth after an act of special creation Explanation for life: Pre-Darwin - Aristotle: Ordered organisms in a linear sequence from least to most complex (humans are placed on the top of the ladder) - Species have fixed essences & the world is eternal & unchanging - Christians: order in the living world proved that it was created by an intelligent designer - 1650 Archbishop James Ussher : ‘Young Earth’ idea - George Louis Leclerc Compte de Buffon: Father of evolution & natural history Questioned the ‘young earth’ idea Saw human as part of nature - Paleontology: evidence that life has changed Georges Cuvier : Fossils resemble modern species Many past species are extinct Mary Anning : Discovered several species of extinct marine reptiles - Thomas Malthus: Essay on the Principle of Population → potential for exponential population increase v.s. arithmetic growth of resources → If special creation and the balance of nature are true, then conditions should be stable and unchanging but: Crowding leads to competition & Species had become extinct (fossil record) - The Diluvial Theory (or Catastrophism): → fossils in the rocks are animals that perished in the biblical flood → The Earth has been shaped by series of catastrophic events (e.g. biblical flood) - James Hutton: Uniformitarianism → denied special creation or any supernatural forces → Earth’s landscapes were formed by the same (natural) processes acting today as had acted very slowly over a very long time → Earth was very very old - The Linnaean System → Putting nature in order → Binomial nomenclature (genus and species names) → based on biological features (e.g. morphology) - Early Natural historians benefited from colonization and slavery Uniformitarianism v.s. Darwin’s theory of evolution: → gradual change caused by natural processes acting over a long time Background and introduction to the theory of evolution Lamarck: 1st articulated theory of evolution — Theory of Acquired Characters (INCORRECT) - Organisms continually arise by spontaneous generation - “Nervous fluid” acts to move each species up the “great chain of being” - Organisms develop adaptations to changing environments through the use and disuse of organs. (Heavy use attracts more “nervous fluid”.) - Acquired characteristics are inherited Problems: No evidence of i. spontaneous generation ii. innate drive toward complexity iii. inheritance of acquired characteristics Darwin - Populations evolve over time through a process called natural selection - Diversity of life arose by “common descent” through a branching pattern of evolution Wallace - Theory of evolution by natural selection The DARWIN-WALLACE THEORY - Individuals within a species show variation - Some of this variation is heritable (offspring resemble their parents more than other members of the population) - Populations have capacity for exponential increase in numbers, but numbers remain fairly constant over time because of competition for limited resources - As a result of successful competition, some individuals (= variants) leave more offspring than others; these offspring inherit characteristics that made their parents successful and later pass them on to their own offspring. (= ‘natural selection’) - Because of natural selection, individuals change and so populations and species change gradually from generation to generation. They become better adapted to their environments (e.g. better at finding food and mates, and avoiding enemies ∴ Requirements for natural selection: Variation, differential reproduction, heredity Darwin Wallace “Natural Selection” “Adaptation”, “Survival of the Fittest” considered sexual selection as an believed it as a form of natural selection independent phenomenon believed in the inheritance of acquired rejected Lamarck’s idea traits stressed that competition was the emphasized that environmental cause of natural selection pressures forced species to adapt to their surroundings Darwin’s importance: - Made the science of ecology possible ∵ he placed interactions between organisms & environment as the centre of our understanding of nature ∵ Emphasized the fact that nature is dynamic Ecology = the scientific study of the interactions that determine the distribution and abundance of organisms L2 The Scientific Approach 1: How do ecologist investigate problems? Science = product & process = a way of thinking /investigating /asking questions about the world History of Scientific Method: - Observational Astronomy (Galileo) - Rationalism (Descartes) - Reductionism = use Maths to represent nature - Empiricism (Bacon) = learning from experiments & observations Hypothesis → Predictions → (Repeated) Experiments → Theories * Scientific ideas are tested empirically (i.e. with objective measurements during experiments); these have to be repeatable because scientific truth must be the same for all observers Proximate v.s. Ultimate causes Proximate: driver that has immediate effect on organisms e.g. understand HOW this works Ultimate: evolutionary explanation of the present day ecological phenomena e.g. understand WHY this behavior exists 4 main types of activity to answer an ecological question 1. Description and observation: discovering a pattern 2. Explanation: hypothesis formation 3. Prediction derived from the hypothesis; then an experiment used to test the prediction 4. Management: application of understanding to influence the original pattern e.g. Government measurements Level of complexity: Communities —> Populations → Individuals Reductionism: reducing the object of study to the sum of its parts by seeking explanations from the lower levels of complexity → But there may be special features that arise from the complex interactions of lower levels Experimental design(* 新pts): - Large enough random sample to capture reality - Confounding variables minimised - Replication is required to increase our confidence that the results are correct. = Repeatable - Interpretation – how do your results fit in with what we already know? What if the data are inconclusive or surprising? Sometimes experiments are difficult/ impractical → Comparison & observation to help Cofounding factors = inconsistent change that affects the dependent variable e.g season, storms Ecology = the study of how organisms interact with each other and with their physical environment Why study Ecology? To avoid global collapse. 9 earth systems: 1. Climate change 2. Biodiversity loss 3. Nitrogen cycling 4. Land-use change 5. Atmospheric aerosol 6. Chemical pollution 7. Ocean acidification 8. Ozone depletion 9. Freshwater use *which the planetary limits have already been exceeded Ecological hierarchy: Organism: A single living thing Population: Same species sharing a habitat Community: Different populations interacting in a particular area Ecosystem: living community + physical environment Landscape: energy & matter exchange between ecosystems (Gov. policy) Global(Biosphere): Total area of Earth where living things are found AVOID Unfalsifiable hypothesis Testing hypothesis: Falsification → Science advances by disapproving hypothesis ∵ impossible to prove hypothesis Hypothesis only has scientific value if it is possible L3 The Scientific Approach 2: Doing Ecological Experiments Set up Null hypothesis — Try to falsify or reject the idea H0 = null hypothesis (either reject or accept): there is no effect H1 = alternative hypothesis: there is an effect Sample = smaller subset of a wider population → systematic sampling → random sampling → No universal correct number on how many samples do we need (Rule of 10?--- get 10 replicates for each variable you are testing) Alpha diversity: the diversity of species in one location Beta diversity: changes in ecosystem/ the change in species as one moves across habitats, communities, or ecosystems → The differences in species composition between multiple ecosystems How can we decide if competition is occurring? Comparisons of distributions of species Identification of a common (shared) species Demonstration that resource is limiting Manipulation of population sizes (or resource) Biases and limitations in ecological research: Positive results or ones that show something interesting or novel → much more likely to be published → negative results are not published often Taxonomic biases *Generality of the experimental results → results may only apply to one microhabitat(=tree), in one forest, in one country, in one season of the year… Consider What is suitable for the questions you want to address What is the scope of your research? What will you understand from it? Is your sample size big enough? Do you have proper replication? L4 The Scientific Approach 3: How do we deal with environmental complexity? Experiments = any test of a prediction or controlled observation Mensurative or observational experiments: measurements of change in dependent variable over space (place) or time Manipulative experiments: two or more treatments are compared over time; goal is comparison between the experimental treatment(s) and the control treatment (usually unaltered) Formal manipulative experimental procedure: Set up a null hypothesis → reject null hypothesis (= control & treatment group are different) Types of Ecological experiments: 1. Laboratory experiments (LE) 2. Field experiments (FE): manipulations in the field by researchers 3. Natural experiments (NE): take advantage of naturally occurring events Best type of experiment? - Control of environment variables and experimental treatments: LE>FE>NE - Scale (space or time): NE>FE>LE - # species included: NE>FE>LE - Generality of results: FE~NE>LE - Ease: LE>FE>NE - Cost: LE>NE>FE Dealing with environmental complexity: — environmental conditions that change alongside our independent variable that we are not directly testing in our experiment → conflicting explanations Solution: SI (Strong inference) — The use of experiments to decide which of 2 (or more) hypotheses is correct 1. Devise alternative hypothesis 2. Devise a crucial experiment with alternative possible outcomes, each of which exclude one or more of the hypothesis 3. Carry out the experiment 4. Repeat until all eliminated 👑 Arrangement of sample units in space: Interspersed , Random… Segregated… Pseudoreplication = dependent replication (e.g. not take mean) Type-1 error = false positive (often pseudoreplication/ clumping) Type-2 error = false negative (not enough samples? L5 What is evolution by natural selection? Evolution = cumulative change in the characteristics of organisms from generation to generation → gradual directional change → can happen in anywhere Darwin’s theory of evolution by natural selection - Variation is heritable - Limited resources → Competition → population size fairly constant - some variants leave more offspring than others → (natural selection) - Individual change from generation to generation → population changes 3 conditions necessary for the occurrence of evolution by natural selection: 1. Variability 2. Heritability 3. Influence of the environment on survival & reproduction* *i.e. some limitation on infinite population growth Time available for evolution: - Earth >5,000m y old - Oldest fossils are >3,500m - 3m y of evolution, Australopithecus afarensis → Homo sapiens → sometimes it can go a bit quicker Outcomes: 1. Macroevolution - occur in geological time (most apparent from fossil records) → result in new species (= speciation), genera, etc Types of Speciation: i) Allopatric: isolation ii) Peripatric: isolated SMALL pop iii) Parapatric: e.g. environmental gradient (spread over a large area) iv) Sympatric: same geographic location (e.g. sexual selection, habitat isolation) due to temporal/ behavioral isolation 2. Microevolution (precursor to macroevolution) - Changes within the population (over a few generations) NO new species - e.g. insecticide/herbicide/antibiotics resistance - e.g. industrial melanism in moths (cryptic color) → not fixed, can go into reverse Perceived problems with the theory of evolution by Natural Selection: 1. Randomness? Ans: Natural selection is a non-random survival, though mutations are random, the action of (gradual, cumulative) natural selection on mutation is non-random 2. Complexity? How? Ans: Very low chance 3. Too many gaps in the fossil record? Ans: - Fossilization is a rare event - Many still underground - Each fossil found is an independent test of our understanding of evolution → Completeness is not essential L6 What are the ecological outcomes of natural selection? Mutation → Variation → may lead to favorable, neutral or harmful changes Evolution — 2 stage process 1. Mutation randomly creates heritable variation (act on genotypes→ phenotypes) 2. Natural selection affects the way these variations are passed on and appear in future generation (act on phenotypes) *Natural selection act on individuals, NOT species (although their general appearance may change over time) ∴ Feature evolved are not for “good of the species”, but for that of individuals 3 Types of Natural Selection act on individuals in populations 1. Directional selection: only 1 extreme is selected → directional changes - Inc. artificial selection caused by humans e.g. oral jaws of African cichlids, fishing industry( ↓ in avg. body size) 2. Stabilizing selection: moderate(average) trait is selected - acts against change & maintain adaptations → uniformity of color patterns or other features e.g. 2 eyes, human birth weight, tall & short flower dies 3. Disruptive selection: both extremes are selected - e.g. the 2 sexes?, gamete sizes - produce dimorphism, trimorphism = apostatic selection - have potential to produce new species (in allopatry) e.g. Salmon, white/black rabbits, short/long beak hummingbirds Sexual selection: - Competition among males for females - Females choice of males leads to ornamentation ∴ Males are usually more colourful than females → Directional selection: male individuals → Disruptive selection: population level (sex dimorphism) 1. Inter- sexual competition: one sex choose which individuals they mate with → more attractive, higher evolutionary fitness → can select things with no other advantages (e.g. long plumage) 2. Intra- sexual competition: competition between males Natural Selection(宏觀地)has 2 types: 1. Individual selection - Direct consequences (increase individual fitness) 2. Kin selection - Indirect consequences (encourage helping or cooperative behavior that ↑ the fitness of related individuals who possess (& pass on) shared genes) Definition: selection acting on one or more individuals & influencing the survival or reproduction of relatives(other than offsprings) that possess the same genes by common descent(=shared inheritance) → increase the fitness of relatives → increase own fitness e.g. bees, Florida Scrub jays, termites, primates(grooming), Tiger salamander (cannibalistic form) Conditions: - All members of a group are closely related - family groups stay together - Cost of helping are low - Fitness benefits of helping are great - Individuals recognise each other & their relatedness → Inclusive fitness (refers to combined effect) = fitness gained by rearing own young + fitness gained by increasing breeding of relatives L7 What is biodiversity & why is it important? = biological diversity or biological variety Most commonly considered: Species level Anthropocene epoch → habitat destruction/degradation, biological invasions, pollution, over-exploitation, global environmental Δ → biodiversity decline Science = (re)-discovering new uses for biodiversity → relieve human suffering & improve conditions of life Scales & patterns: - Across latitudes: types of ecosystems, habitats & numbers of species differ - Among & within habitats/ecosystems: microhabitats differ in species richness - Among taxa: species richness varies among taxonomic groups e.g. insects have more species than all other organisms combined Large majority of species diversity is terrestrial - Within species (= among populations; different gene pools) - Wide size range of organisms: microscopic to giant Titanosaurs How many insect species are there on Earth? → collected beetles (Coleoptera) from rainforest tree canopies in Panama using insecticide fogging → 1,200 beetle (Coleoptera) species from 19 individuals of one tree species (Luehea seemannii) → 163 of the 1,200 beetle species (14%) estimated* to be host-specific specialists on Luehea seemannii → Beetles represent ~40%* of insect species richness in rainforest canopy, so there are 2.5 (or 100/40) x 163 specialist insects on Luehea → 405 specialist species → Estimated* that 2/3 of specialist insect species on Luehea seemannii are in canopy, so total for entire tree is 1.5 ( or 3/2) x 405 → 608 specialist species → There are ~50,000 species of tropical trees; if* each support same number of specialist species, tropical insect total is 608 x 50,000 → 30.4 million species ! Each * is an assumption affecting final total The diversity of life: ~2M described 80% of unknown species Most estimates around 8-10M species −1 18,000 species described 𝑦𝑟 How much biodiversity is being lost? 5 M ha of tropical forest lost/year >40% of the land that could support tropical rainforest (TRF) now lacks it; clearing continuing at a rate of ~0.52% area remaining per year; higher in Asia: ~0.91% (6% in some areas, e.g. Sumatra) TRF supports >50% of species on Earth Loss of species today (1 sp. per 1000 spp. /yr) 1000中1 V.S. Estimated pre-human rates of species loss: 0.1 sp. per million spp./yr (over 10000 times faster) million中0.1 Why should we care? - Extinction is forever → loss of species & genetic information is irreversible - Genetic resources (for pharmacological use & biotechnologies) → potential to benefit humanity (=option value) is lost - Consumptive & commodity value “Free” ecosystem services Agricultural services of pollination - Amenity value: improves our lives in non-material ways = educational, recreative or cultural value - Moral value: organisms have value in themselves for themselves - Heritage value for future generations - Ecosystem services(e.g. generate O2, CO2 fixing; maintain soil fertility; fisheries) = provisioning/regulating services (value) - Species linked in a complex web of ecological interactions - Consequences of loss of species are unpredictable, some may be ‘drivers’ or keystone species (雖然有啲可能係functionally redundant ‘passenger’) - Precautionary principle Anticipation & prevention is better than reaction & repair Biodiversity in HK HK terrestrial life: >21,000 species estimated (most unknown) HK marine life: 5943 species (6500 estimated) Rich in biodiversity with endemic & globally endangered species L8 How do adaptations evolve? Genes code for phenotype which, through interaction with the environment, allow more or fewer copies of the genes to be passed on to the next generation Successful genotypes are those coding for phenotypes that pass more copies of the genes (genotype) to the next generation Key point 1: Natural selection acts on individuals (phenotypes), but genes are selected - bodies are like “survival machines” for genes - "An organism (= phenotype) is a gene's way of making more genes“ Key point 2: The success of a phenotype (at promoting gene transmission) is determined by its interactions with the environment, which depend on its adaptations Natural selection (defined) = non-random & differential reproduction of different genotypes, acting to preserve certain variants (well-adapted or fit phenotypes) & eliminate others Fitness: (defined) = the proportionate contribution* of individuals to future generations *viable offspring is relative to others, not absolute Adaptation = any feature of an organism (morphology, physiology, behavior, etc.) that increases survival & reproductive success → greater fitness 1. Adaptive Radiation: - accumulation of different adaptations - defined as “evolution of a single ancestral species to produce several descendant species with specialized adaptations & lifestyles” 1 generalized ancestral species → many specialized descendant species - Or “ …evolutionary divergence of an ancestral species into a series of different niches” (= lifestyles) e.g. Darwin’s finches - Outcome: - Descendants live in a narrower range of habitats than ancestors - Species in different environments have different adaptations (even if related) ∵ different selective pressure - Species in the same environment show similar adaptations (even if unrelated) ∵ same selective pressure *唔等於organisms are perfectly adapted to their environment → Adaptations are imperfect Why? 1. Evolution may lag behind 2. Resources are limited, so all adaptations cannot be 'perfected' simultaneously: e.g. egg size & egg number cannot both be maximized. Plus adaptations must be seen in the 'whole animal' context (e.g. large offspring → birth difficulties) 3. Genetic variability may be limited, not all possible ‘adaptive solutions’ are attainable, mutations may be absent Complications: 1. There may be several adaptive solutions to the same problem → may not have ‘best adaption’ 2. Phenotypic plasticity: Some phenotypic differences are induced (caused) by the environment e.g. ‘Fatness’ is induced by diet, & a parent’s body weight is not inherited by its offspring. (BUT the ability to put on weight (= potential for such phenotypic plasticity) is inherited) 3. Acclimation vs Adaptation Acclimation: a shift in an individual’s range of physiological tolerances. It is useful in response to seasonal and other persistent changes in conditions - may take days to weeks, is reversible - The reaction norm: ”phenotypic expression of a single genotype across a range of environments” L9 Species Interaction (In community ecology level: interaction between and within populations and environment) Structure: Food webs As you go further up the food web, species become more generalist, and eat other species from a wider range of habitats ‘Pyramid of numbers’ – number, weight or energy of individuals in each trophic level Trophic level – position of an organism in a food web Ecological role — functional roles and what organisms eat Niche – biotic and abiotic conditions required for a species to exist and the role a species plays in an ecosystem n-dimensional hypervolume — useful in quantifying and comparing species's niches Fundamental niche – possible env. a species could occupy Realized niche – actual env. a species lives in due to species interactions Trophic ‘pyramid’ Incorporated energy transfer using the Cedar Bog Lake as a model system (both biotic and abiotic) - Described pathways of energy flow and nutrient cycling - Related E flow and ecosystem structure to ecosystem function - ‘Rule of ten’:when energy is passed in an ecosystem from one trophic level to the next, only ten percent of the energy will be passed on Food web indicates trophic relationships among species within a community Species interactions Predation and herbivory (+/-0) - Predator usually kills prey - Herbivory doesn’t necessarily result in killing the plant, but reduce its fitness (growth, fecundity) - Predator or herbivore preferences – proportion of prey in the diet of a predator vs proportion in the environment (optimal foraging strategy) Parasitism (+/-) - Parasite consumes all or part of host - Different to predation because the host doesn’t necessarily die - E.g. malaria, parasitic wasps, lice, leeches, mistletoe Competition (-/-) (interspecific) - When individuals of one species suffer a reduction in fitness from a second species due to their shared use of limiting resources (both biotic and abiotic) - Exploitation competition of shared resources or interference competition by restricting access (direct action) - Competitive exclusion: Exploitation competition Some species will be better at exploiting resources than others - Resource partitioning: no two species will occupy the exact same niche in the same location Mutualism (+/+) - Reciprocally positive interaction between species - E.g. Seed dispersal, pollination, mitochondria Commensalism (+/0) one species benefits - Any interaction in which at least one species benefits – generally in close proximity - “An interaction in which the presence of one species alters the environment in a way that enhances growth, survival, or reproduction of a second, neighboring species” - Ecosystem engineers - e.g. nitrogen fixing plants that benefit surrounding plants Termites - ecosystem engineers Important for: - Decomposition - Retaining soil moisture (sheeting) - Soil nutrient heterogeneity Others: Ants: Certain ant species modify soil structure and nutrient availability through their nesting and foraging activities. Beavers: They create wetlands by building dams, which alter water flow and create habitats for various organisms. Why are food webs shaped the way they are? Depends on many interacting factors across time and space Biogeographic history Climate and microclimate Host plant range Environmental stability Population dynamics and survival processes Keystone species — species that plays an important role in an ecosystem → Shape the function and diversity of the rest of the ecosystem e.g. sea star: control number of mussels; sea otter: control no. of sea urchin →maintain kelp population Trophic cascades – when one part of food web is suppressed, influencing other parts Biocontrol: applying species interactions to ecological problem → use of species to reduce pest species Agricultural & natural ecosystems Releasing large numbers into a habitat – often predators, pathogens, parasites Importing enemies of pest or invasive species/increasing effectiveness of native enemies can be long or short term Methods: - Find a range of potential natural enemies and evaluate their potential effects - Carry out small-scale experimental controlled releases - Monitor through time - Scale-up releases - Introduced species can adapt, disperse, change host preference – need to monitor carefully (which often is not done) Successful stories: - prickly pear became invasive Australia → Cactoblastis Moth was introduced → destroyed the infestations Failed: - Cane toad initially introduced to Australia to kill sugarcane pest beetles → outcompete & predate on species that are native & not adapted to their presence + didn’t reduce beetles L10 The mating game: conflict or cooperation between the sexes? Monogamous (♂ & ♀ form a pair bond) or Polygamous (each sex may have >1 mate)* Polygamy: - Polygyny: 1 male & many females (each F mate with 1 M & responsible for parental care) - Polyandry: 1 female & many males (each M mate with 1 F & responsible for parental care) What percentage of animals are monogamous? 1. Birds are mostly monogamous: - Female: has to take part in parental care ∵ this ↑ her fitness to look after her (expensive) eggs → cannot take risk having them abandoned by male - Male: usually invest in parental care → yield higher potential fitness gains than leaving the first F to find another female Why yield higher gains? i. eggs must be incubated until they hatch which requires both F&M ii. Altricial* nestlings (can’t look after themselves) have high demand for food & their chances of survival are much greater with presence of both parents iii. Females have seasonal synchronization of reproductive cycles (by day length); male has little chance of mating again if he leaves female *Opposite is precocial (e.g. chickens, ducks) BUT F tolerate sharing M with our F under certain circumstances - If male bird holds a high-quality territory that he defends for exclusive use of females (+ offspring male has fathered), then sharing is tolerated by females. - Easier for female to rear young without help on a good-quality territory than with help of male on a poor- quality territory - More likely to happen when young are precocial 2. Mammals are generally polygynous - F are limited resources → adv. for M to mate many times 5% of mammals are monogamous - usually territorial species &/or predators: males contribute by defending territory for females & offspring, &/or providing growing offspring with food (= prey) Maternal parental care Maternal parental care is more common than paternal care. 1. result of internal fertilization and the delay between mating and birth (gestation). 2. relative costs to the two sexes of being the caregiver. For males there is uncertainty about paternity, which will reduce the benefit to cost ratio of engaging in parenting. + for males when there are opportunities to mate with multiple females, males that give up that opportunity to engage in parental care will pay too high a price. Paternal care (either with the female or alone) would be selected for only when the payoff is sufficient to outweigh the costs. Maternal care: Membracinae (treehoppers) — 3 independent origins of female parental care (egg guarding), none of male parental care Paternal Care 1. fishes - common paternal mouth brood eggs or care for eggs in nest - Costs of parental care in these cases seem to be lower for males than for females. E.g. because females prefer males that engage in parental care or because males can take care of several egg clutches. e.g. St. Peter's fish 2. male water bugs - guard and moisten eggs above the water (Lethocerus) or carry eggs on back (Abedus, Belostoma) - Abedus eggs do not develop unless aerated by male - water bugs are predatory insects (catching fish, frogs and tadpoles) → they are large and consequently their eggs are too. This is why oxygenation is necessary. - Why only male care? 1. Male water bugs with one clutch of eggs sometimes attract a second female. 2. costs of parental care may be disproportionately great for females in terms of lost fecundity. Evidences of cost & trade-off of parental care 1. Great tits — produce 2 broods each year → examined effects of changing size of 1st brood (by -4, -2, 0, +2 & +4 eggs from original number) on 2nd brood. - Results: i. more fledglings in enlarged broods than control ii. they are underweight with poor survival; fledglings in reduced broods were heavier & survived better iii. Few adults with enlarged broods produced 2nd brood, vice versa 2. Coots Chick color affects parental care in mixed broods of coots → Control broods were unaltered (orange) or had orange feathers trimmed (black). Experimental broods had 1/2 orange, 1/2 black chicks - Chick colour likely indicates offspring health 3. Belastoma flumineum (Order Heteroptera) Waterbug - No significant difference in snail group; significant diff. in fish grp. Discriminating prenatal care - e.g. Mexican free-tailed bats They use vocal and olfactory cues to identify their offspring from among thousands in the creche The bats do occasionally make mistakes but the benefits of leaving a baby in a creche (mainly thermoregulatory) appear to outweigh the cost (accuracy from allozyme data: 80%). - e.g. Cliff swallows Often nest in large colonies & their young produce much more variable calls than do Barn swallows & distinguish calls from barn swallows Adoption - In some instance may be beneficial to the adopter e.g. Goldeneye duck Dilution effect → save own youngs Conflict of interest between sexes “Battle of the sexes” - Males: maximize the number of mates & offspring fertilized - Females: restricting the number of mates of each male, & involving him in parental care → Results in males compete for females & ‘sneaky’/’parasitic’ behaviors Extra-pair copulations (EPCs): mate 'secretly' with other paired females → increase males’ fitness (even in monogamous birds) → Males in EPCs parasitize the parental effort of other males e.g. Zebra finches (Taeniopygia guttata): 8% of young result from EPCs; up to 35% in some bird species Swallows (Hirundo rustica): 26% of young result from EPCs, but EPCs only 7% of copulations seen (= 'sneaky') Sexual cannibalism - only evolve in predators - Female praying mantis gains fitness by eating her mate - Should a male allow a female to eat him? a) Number of additional young male would father if female eats him (NB: assumes assurance of paternity) b) Number of times male can expect to mate. If it is low, male might allow female to eat him – e.g. if females are rare, or if males have trouble finding them ‘Sneaking' can also occur in instances of courtship where the male exposes himself to risk from predators in order to attract females e.g. Large male frogs call to attract females; smaller “satellite” males wait near them hoping to encounter a female on the way to a calling male (might only call if large males are not present) → Males will become ‘parasites’ upon loudspeakers broadcasting exceptionally loud calls. M+M ‘parasitism’ in fish ‘Satellites’ M attempts to shed sperm near an egg-laying F in the company of a competitively-dominant M fish e.g. Tripterygion delaisi (Black-faced blenny) - Small common rocky shore fish - Feeds on benthic invertebrates - External fertilization - Females lay eggs on nests and male eject sperm directly on them. - Two alternative mating strategies: Territorial male Sneaker male changes colour No change in colour guards nest `sneaks` in when female Territory defense lay eggs Female courtship L11 Is insect colouration a defensive adaptation? Life-dinner principle: A prey may lose its life, while the predator may lose a meal → pressure on prey >>> predator Predator-prey coexistence Top-down control: Predators exerting to their prey Bottom-up control: also referred to as nutrient or resource limitation → Both population-level mechanisms → result from defensive adaptations arising from strong selective pressure on preys to improve their defences 1. Protective adaptations: against hostile physical & chemical factors in the environment e.g. dark colouration of this arboreal ant being an adaptation against high UVB radiations → leads to convergence in response to similar physical stresses 2. Defensive adaptations: against attacks by other organisms (usually predators) - Can be very diverse - Agents shaping adaptations: biological nature (living organisms) - Biological factors stimulate mutual evolutionary responses in the traits of interacting populations. In contrast, protective adaptations do not trigger reciprocal effect on the environment → foster diversity of adaptations Coevolution: Sequence of evolutionary responses resulting directly from the interaction between the two populations (e.g. prey and its predator). = Adaptations in one population (e.g. prey species) promote the evolution of adaptations in the other (e.g. predator species) *note coevolution is not specific to predator/prey e.g. Colouration The “defensive arsenal” of preys - Most species are both consumers (primary/secondary) and preys 1. Primary defences act regardless of whether predator is present or the prey is aware of it Either the predator does not distinguish the prey from its background (crypsis in cryptic insects) or the prey is detected but not recognized as edible food (mimicry in mimetic insects) → Crypsis & mimicry = primary defences - can be visual, audio, thermic or chemical - there are cryptic predators too 2. Secondary defences: when prey is detected and identified as potential food by predator (when primary defences failed) and can take different forms: - Escape behaviour (e.g. run, flight) - Chemical release or injection (e.g. sting) - Mechanical defences (e.g. bites, kick) Aposematic insects Species with good secondary defences (e.g. chemical defences) and which advertise them with bright colours or patterns → colour is the primary defence: warning colouration = aposematism Aposematic: appearance that warns off enemies because it denotes something unpleasant or dangerous - Contrast of a prey's colours against a background → Opposite of crypsis - often associated with other sensory modality: sound, smell, tastes or texture → Reinforce the signal → Predators learn to avoid them or in some cases have evolved innate aversion *BUT production of chemicals or their absorption from plants is energetically costly e.g. Monarch butterfly Toxic as larva (caterpillar) and adult due to secondary compounds (cardenolides) ingested and stored from milkweed (Asclepias genus) during larval stage *BUT Higher concentrations of cardenolides decrease performance in adults (e.g. decrease migration success) Defensive benefits of aposematism - Predators must learn characteristics of aposemete (i.e. association of colours with 'unpleasantness' & learn to avoid aposemete) - Important for aposemetes to not be killed or injured during learning process: e.g. hard cuticule, stinger, reflex bleeding, early predator detection - Complete avoidance is not necessary for aposematism to work; aposematism merely has to provide lower mortality than crypsis for the warning signal to be beneficial Warning signals need to be easy to detect, discriminate and memorize by the predators → survival value might depend on predators’ learning ability Innate avoidance of bright colours by some vertebrates Aposematic colours increase - the speed and durability of avoidance learning + elicit avoidance in naive predators - the probability of recognition in already experienced predators Experiment: Altering the colour pattern of the forewing of Heliconius erato → Frequency of major damages observed on altered individuals much higher Limitations of aposematism Risk of death (or injury) during the predators’ learning period defence can never be 100% effective More likely to evolve in species that are abundant → ↓ per capita risk of an individual of being sacrificed to inexperienced predators Evidence for survival value of crypsis? - Controversial due to lack of standardization and lack of data on relative abundance of cryptic vs. non-cryptic insects - Need for experiments Example: 1. Industrial melanism — Peppered moth Biston betularia typica morph(white moth): only form reported by 1850 carbonaria morph(brown moth): reported after 1850 in areas near industries (heavy pollution) 2. Stick insect - Timema cristinae. Polyrmorphic herbivorous insect On plants, predation (by lizard) of grey morph is higher; On the ground, predation of green morph is higher → Green morph more cryptic on foliage; Grey morph more cryptic on ground Limitation of crypsis Need to select the proper background / habitat → Conspicuous on wrong background Constraints the insect to a sedentary lifestyle at least during daylight / limit habitats exploitable Need for secondary defences when primary defences fail or when not efficient against particular predators (e.g. using non-visual cues) ∴ More advantageous to be cryptic if rare/ predators have poor memory (/can’t learn) Forms of Mimicry Mimicry: resemblance of one species of insect/animal (the mimic) to another (the model) so that a third species (the predator) is deceived by the similarity & confuses the two (mimic & model) 1. Müllerian Mimicry Two or more unpalatable or harmful species evolve to resemble each other. 2. Batesian Mimicry A harmless species (the mimic) evolves to imitate the appearance of a harmful or poisonous species (the model). E.g. Cockroach mimicking the beetle (the model) 3. Peckhamian mimicry An example of Peckhamian mimicry is a spider that looks nearly identical to its prey. By mimicking its prey, the predatory spider is able to invade the territory of the prey (ant) without the prey noticing the danger and running away. This then allows the predator to capture its prey. Müllerian mimicry Among aposemetes with similar warning colouration (i.e. have same ‘signal’) → all species are unpalatable - Can engage hundreds of species across vast regions, e.g. Velvet ants (which are wasps of the Mutillidae family) → each species individually does not have to be abundant to wear aposematic coloration Batesian mimicry A palatable species (the mimic) gains a degree of protection from predators by resembling an unpalatable species (the model) E.g. between tiger beetles (model) and a grasshopper (mimic) Ants (model) and jumping spiders (mimics shape & behavior – Salticidae) - Limitations mimics generally require the presence of the model to gain significant protection *The relative abundances of models and mimics* influence the mean rates of predation on these types. → large ratio, decreased predation BUT The mimic species must not become too common relative to the model, then the predators will learn of their presence and tend to increase their attacks Solution… Mimic species will use multiple aposematic models for mimicry – thus generating polymorphism within the same species So is colouration all about predator defence? Not only. Colouration is also selected by other factors - Intraspecific sexual communication (e.g. spiders) - Social interactions facilitation (e.g. wasps) - Thermoregulation - UV protection (e.g. melanism) (protective adaptation) - Parasite defence → These different selection pressures can show convergent or divergent directions → For some species (nocturnal/ subterranean/ troglodytic) the role of colouration can be minimal (no light to reflect colours) L12 Optimal foraging: What to eat, where, when and how? Foraging: The suite of activities involved in the exploitation of a resource. The term is mostly used for food resources but can be used for other resources such as nesting material, finding mates… Food resources vary in: Spatially – most food resources are available in an unpredictable fashion, few are spatially constant (e.g. fruits on particular trees, honeydew from sap-sucking insects) Temporally – food supplies are generally available in an unpredictable fashion In terms of quality – while foraging, organism encounter prey of unequal value (e.g. small vs. large worms) In terms of accessibility – close or far from the nest, areas with low or high competition/predation How to forage optimally & maximize the net rate of energy gain? What? Where? How long? Optimal Foraging Theory: organisms should maximize profitability i.e. energy gained per unit of time spent foraging in a given patch (rate of energy gain) - hypothesis: Nature selection should favor “efficient” foragers → individuals that maximize their energy or nutrient intake per unit of effort - Benefit (B): in terms of fitness gain → E available in food (Joules) - Cost (C): search & acquisition (handling) → E or Time (*may有其他C) - Maximized: B/C >1 - B/C = Energy Gained / (Search cost + Handling cost) Eg / (Es + Eh) or Eg / (Ts + Th) → Determines what to eat from a variety of resources Example: Pied wagtail → prefer medium size preys ∵ Small: not enough E; Large: too much C relative to E gained As the number of prey types increases, the average searching time decreases As the number of prey types increases, the average handling time increases An optimum is found when both searching and handling times intersects representing the best strategy in terms of prey types optimization ∵ Ts + Th or Es + Eh are kept to a minimum, so B/C is maximized Predator searching for P1, but finding P2. Should P2 be eaten or should the predator keeps looking for P1? (*P2 no need Ts, only Th) ∴ Strong weight of Ts1 Why do animals need to maximize energy gain? To maximize time budget use and different functions to accomplish Higher net energy gain is beneficial for: - Growth (size & rate) - Reproduction (frequency & offspring size/number) - Defense - Repair (e.g. resting) - Storage (fat, etc.) A consumer (e.g. predator) optimising its effort should choose the most profitable food — which yields the highest energy gain per unit of time (not always largest item) Predictions about diet Assumption: consumers maximise their net rate of energy gain(optimal animal) 1) A consumer should eat only the most valuable prey type if the encounter rate is high (because inclusion of other types with lower food value would decrease the rate of energy gain) – be more specialist 2) If the most valuable prey is encountered at a low frequency, a consumer should expand the range of preys eaten to include the next most valuable type (& so on) – be more generalist 3) The optimal animal should eat any food item it finds unless, during the time spent handling or eating it, there is a good chance of finding a more valuable type of food item ∴ The diet should broaden as productivity of the environment declines (frequency of encounter with most valuable prey type falls) → Animals in unproductive environments will be generalists (otherwise too much time spent searching) → Animals in productive environments will be specialists (high encounter frequency allows focus on prey types with low handling time or high energy content) Where is the best place to eat? Landscapes are a mixture or mosaic of more-or less-valuable patches (= areas of distinct habitat type) separated in space → Scale dependent - If consumer gains more in exploiting highly profitable patches, the question is when should they leave it (as resources deplete)? Marginal value theorem — predicts the length of time an individual should stay in a resource patch before leaving and seeking another, based on 3 variables: - Richness of the food patch (prey density) - Time required to get there (travel time) - Time required to extract the resource (acquisition) 1) Initial time cost associated with traveling to the patch (no energy gain) 2) Once foraging is initiated, the rate of energy gain is high 3) As resources deplete, rate of energy gain decreases The rate of E return: the cumulative value of G divided by the combined travel time (t) and foraging time (T) The max. rate of energy gain: the line that is tangent to the curve of cumulative gain with time (here shown as Gopt: G optimal) Rate of return per unit of time: G1– increasing G2 – declining → Past Gopt, it is optimal for an organism to leave the patch both patches have the same quality but differ in their distance to consumer: travelling time t2>t1 The theorem predicts that consumer should then spend more time to exploit resources (foraging) in patch 2 than in patch 1 → In other words, increasing travelling time increases exploitation time required to optimally use a patch patches are at similar distance but have different resource values The theorem: spend more time to exploit the patch with higher resources value → Animals prefer to feed in the richest patches & so these patches will attract most animals (& they will stay longer). Animals ignore poor patches if richer patches are available Trichogramma brassicae - Female can discriminate between healthy and already attacked hosts (eggs) - Results: Females spent more time on patches of higher quality - All patches were reduced to the same level of profitability before being left by females L13 What prevents populations from growing indefinitely? Population: group of individuals of the same species that inhabit a given area. They rely on similar resources and are influenced by similar environmental factors Two important features: 1) Potential for interbreeding among members of the population (in sexually reproducing organisms). → Defines the gene pool 2) Geographically defined - spatial concept Structure: - Proportion of individuals in various age classes & sexes - Density (the number of individuals per unit area) - Spacing of individuals relative to each other (trees or nests) - Dynamics: Changes through time that results from the birth, death, and movement of individuals Demographics: Study of the vital statistics of populations & their changes over time Age specific mortality How to calculate? - Iife table — follow a cohort of individuals X: age classes (in years); nx: no. of alive individuals at a specific age (X) lx: prob. at birth of surviving to any given age E.g. l1= n1/n0 = 159/530 = 0.3 l2= n2/n0 = 80/530 = 0.15 dx: age specific mortality E.g. d0= n0 – n1 = 530-159 = 371 qx: age specific mortality rate (death rate) E.g. q0= d0 / n0 = 371/530 = 0.7 → Graphic construction of survivorship (lX) and mortality rate (qX) derived 3 general idealized types of survivorship curves Type I: Strong survivorship during most of lifespan followed by heavy mortality at the end (e.g. “modern” humans) Type II: No variation in survivorship with age (e.g. rodents, some plants) Type III: Mortality rate extremely high in early life (e.g. oysters, fish, many invertebrates, most tree species) * Most populations have survivorship curves that combine features of different types Population Growth: represent increases or decreases in the number of individuals within a population over time - influenced by 4 factors: Births (b); Deaths (d); Immigration (i); Emigration (e) Closed population: no or negligible migration movement Open population: migration movement occur Change in population size (ΔN) over a time interval (Δt ): ΔN/Δt = B - D Propose R=B-D, R: Difference of B&D occurring in a time interval → So ΔN/Δt = R But ecologists are interested in calculating changes in population size on a per individual (per capita) basis: r – intrinsic(/per capita) rate of increase E.g. N=1000 individuals; R=16 individuals(in 1 yr) → r=16/1000 = 0.016 Assumption: a population with a stable age distribution The equation for exponential growth is expressed as: dN / dt = rN dN / dt represents the rate at which population is increasing in size at each moment in time If r > 0: exponential growth of the population, more or less delayed in time If r =0: population is at an equilibrium If r < 0: population size will decrease over time Examples of exponential growths: African elephant, St. Paul reindeer etc. → Even species with slow birthrate and late maturity age will show exponential growth Limits in population growth Thomas Malthus (1798) notes “a strong and constantly operating check on population from the difficulty of subsistence” → assumes that resources are abundant (or even unlimited) BUT resources are LIMITED as the size of population increase → each individuals has access to fewer resources Carrying capacity - Carrying capacity (symbolized as K) is the maximum population size that a particular environment can sustain - These limiting factors can be: energy (food), shelter, refuge from predators, nutrients, water, suitable nesting sites… → influence birth and/or death rates → ultimately modifying the intrinsic rate of population growth (r) Logistic Growth Model — the carrying capacity is being included dN / dt = rN(K-N)/K As the population size approaches the carrying capacity (K), the growth rate decreases Example of logistic growth: - Lab culture of bacteria However in some organisms, K is exceeded temporarily → In this case, females store food reserves allowing them to exceed the carrying capacity temporarily Long term fluctuations 1. may result from predator prey interactions → top-down control/ bottom-up control - A lag period is observed in the response of predator’s population to the population of preys 2. Severe winters – population decline e.g. grey heron Influences on population size 1. Extrinsic Abiotic factors (density independent way) - e.g. temperature, precipitation, and catastrophic events, alter birth and death rates largely without regard to the numbers of individuals in a population - Populations do not experience favourable conditions long enough to get near K (when the logistic equation applies) they simply fluctuate ‘randomly’ in response to the weather, etc. 2. Intrinsic biotic regulation Biotic factors — feedback mechanisms that increase in severity as N approaches K Density dependent factors (DDF) have an increasing effect or intensity (esp. on births or deaths) with increasing density: e.g. competition of all sorts (incl. territorial behaviour) & diseases Density independent factors (DIF) have an effect (mainly on deaths) the intensity of which does not vary with density. DIF do not regulate population size DDF – influence a population in proportion to its size They function by slowing the rate of population growth with increasing population density by: - Increasing the rate of mortality (d): density dependent mortality - Decreasing the rate of fecundity (b): density dependent fecundity - Or both increasing d and decreasing b - f DIF (Example: Grey heron) - Severe weather causes population declines but N soon returns to equilibrium density (~4,000 pairs) - This fish-eating bird (grey heron) is unable to find sufficient food when inland waters become frozen for long periods - Population crashes are simply the result of density independent mortality from which the herons rapidly recover → Density independent mortality can be rapidly recovered DDF (Example: Red Grouse) - Important fluctuation but long term stability - Feed on heather (Calluna vulgaris) shoots - Males are territorial - spend winter on territory with females & breed in spring - Only birds on territories breed - Females feed on Calluna in territory; offspring spend 3 mo. with parents eating Calluna in territory (Quality of the territory is very important) - Number of eggs laid depends on nutrient status of Calluna (tested by fertilizing areas of habitat) & grouse numbers (overgrazing → nutrients ‘locked up’ in bird bodies): so ↑N → ↓ natality - Chick mortality high if quality of Calluna is low - Birds that cannot obtain territories during winter suffer high mortality - Territory size is a main factor regulating populations via influence on natality & mortality - Territory size is determined by aggression of males and depends on their diet: low quality Calluna when chicks → become more aggressive adults Aggressive adults take large territories & so fewer grouse can breed → increases mortality & decreases natality Density dependent population regulation because high grouse density results in low Calluna quality Other factors: Parasitic nematode worms (Trichostrongylus tenius) in grouse guts at high N (~ DDF) may be important in some populations → higher density → higher chance of spreading of this pathogen What stops populations growing infinitely? - Some populations are at equilibrium (stable, regulated); others are at non equilibrium (unstable & fluctuating but experience some limitations) - DDFs (biotic) & DIFs (mainly abiotic) affect population size; only DDFs can regulate populations - A combination of factors can apply at different ages; importance in each population depends on environmental circumstances - No general rule but, unless environment is very harsh (= intense DIF), DDFs must operate eventually in ALL populations Importance of population ecology Understanding population variations over space and time is key for many fields in ecology and economy: - Conservation (e.g. design of a protected area for a species) - Natural resources managements (e.g. fish stocks) - Agriculture (e.g. pests outbreaks and control) - Human societies (e.g. retirement pensions & public health) L14 Why do some animals live in groups? Living in groups VS social life - Living in groups is not necessarily a synonym of social life - Many live in groups under the influence of abiotic or biotic factors originating from their environment E.g. limited sustainable habitat area (mussels) Particular environmental conditions (moths) Temporary abundant food resources (vultures) But if gathering process emerge from individual interactions → sociality starts Joining a group or not? Benefits: - Protection against predators (e.g. increased vigilance, dilution effect, fighting or mobbing ability) - Finding mates (many potential partners) - Improved food finding ability (increased foraging efficiency, resource defense, cooperative hunting) Example: Predation in group in the Neotropical wrasse (Thalassoma lucasanum) on fishes embryos of Pacific sergent major (Embryos are defended by males) → Group hunting increases success and time spent hunting Main costs of the group: - Competition – resources have to be shared and thus the per capita benefit has to be larger in group - Increased predation and pathogens load: Group of individuals represent a valuable resource for predators – presence of vulnerable offspring Increased spread of pathogens (e.g. parasites & diseases) Natural selection Individuals should maximize their benefits and minimize their costs, with natural selection selecting for such behaviours → So they can maximize their fitness (reproductive success) BUT the presence of sterile castle in some social insects ??? Social behaviours Natural selection should favour behaviour that benefit the donor Spitefulness — behavior that reduces the fitness of both donor and recipient (not encountered in nature) Altruism: behaviour that increase the reproductive success of the beneficiary, while diminishing the reproductive success of the donor → evolutionary conundrum (riddle) Origin of eusociality * A groundbreaking idea is that natural selection does NOT operate on individuals but on their genes Let’s imagine that altruism behaviour is carried on a single gene The recipient of this behavior received a benefit “b” in the increase of gene copies in the next generation The donor of this behavior received a cost “c” in the decrease of gene copies in the next generation Hamilton’s rule (1964) If donor and recipient are related, then altruist gene can be selected and increase in frequency in population if: c cost of increased competition Ex: Red-cockaded woodpecker groups are male-biased Males help feed young Available nesting cavities are rare Example 2.0: Seychelles warbler sex ratios → As territories fill up, females on good territories produce fewer males (which disperse) but more females (which help) What is the extent of this variation in Homo sapiens? For males, maximum recorded reproductive success is ??? children (fathered by Moulay Ismail, the 'bloodthirsty emperor of Morocco') For females it is ?? Equivalent figures for Elephant seals are 100 versus 8; for Red deer are 24 versus 14 How to determine sex? “What is the evidence of deviations from 1:1 sex ratio in other taxa/groups? Fig wasps are in the order Hymenoptera where females store sperm & control the sex of the offspring (= haplodiploidy: unfertilized eggs → males). - Exclusive (or assured) sib mating is an extreme case of local mate competition: brothers & sisters always interbreed (so fittest parents maximize female number & minimize males). - The mite Acarophenax tribolii (order Acarina) feeds on insect eggs - average brood size is 15 - only 1 is male Chromosomal (genetic) sex determination supposed to be the rule for mammals, birds, etc. - but it is that simple?? (e.g. Komdeur et al. 1997) Seychelles warblers adjust % males in a clutch from 10% to 90%, depending on environmental conditions: e.g. need for helpers (daughters are better), or intensity of competition/food shortages (non- helping sons are better as they leave the area) Environmental Sex Determination Incubation temperature Terrapins Turtles Alligators Social Sex Determination Many fish can undergo sex reversal Depends on mating system Example: dominant female in Anthias sea bass harem changes into male when territorial male disappears L17 Case study 2: How (or why) did the guppy get its spots What is guppy? - Poecilia reticulata (Poeciliidae) - Lives in gravel-bottomed forest streams: headwaters to lowlands - Great variation in coloration from population to population, even within the same stream - Lots of natural predators; generally more and the most ferocious are at lower elevations (downstream) → higher risk in downstream → a longitudinal gradient of selective pressure from predators - Most dangerous: Crenicichla alta (mainly downstream) 15-30 cm - Least dangerous: Rivulus hartii (mainly upstream) 8-10 cm, eats baby guppies - Brightest guppy populations tend to live upstream: populations downstream are duller in color - Females are dull gray (and blend in with the stream bed) in all populations - Mixture of black, blue, green, orange & red spots (may be iridescent) in different shapes & combinations; - Only males have spots - Guppy populations vary in spotting: smaller & fainter spots where there are more enemies; bigger & brighter (red, blue &/or iridescent) where few enemies - Males (1.5-3.5 cm) much smaller than females (3-6 cm) - Colour, size & brightness of spots is heritable - Subject to artificial selection in aquarium trade (focus on M); an (introduced*) invasive species globally (>40 countries) *For mosquito control Reproduction - Live-bearing(viviparous): fertilization is internal & eggs hatch in body of females → well-developed young are ‘born’ after the yolk sac is absorbed - Early sexual maturity + multiple broods (12 per year; brood size up to 100) → fast pop^n growth (exponential) - Sperm transferred via gonopodium; modification of pelvic fin of males into a flexible, tube-like structure Consequence 1: internal fertilization has implications for mate choice; small, colourful males & drab larger females Consequence 2: live-bearing habit makes them successful invasive species globally (5 spp. are important) In order to mate, males have to be attractive to females; so if predator pressure is low, worthwhile to have bright spots even if lifetime is reduced → selection towards colorfulness upstream If predator pressure is high, dull & small spots are better to ensure live long enough to mate successfully → selection towards drabness downstream (males gets very close to ensure potential mate sees spots) What are the necessary conditions for evolution by natural selection? 1. Variation 2. Heritability: offspring resemble parents 3. Survival and reproduction are not random: consistent relationship between phenotype and fitness What is an adaptation? The phenotypic variant that results in the highest fitness in a given environment Endler’s experiments: Controlled pool experiment Set up guppy populations in pools in a greenhouse Foundation population highly variable Sexual selection constant (always) Vary predation pressure Vary environment Track phenotypes through time: number, size, location, color variation of spots Results: - Ponds with no predators (K) showed a steady increase in the # of spots - Little change in spot # (compared to the control) after addition of the innocuous predator (R) - Pools that received a voracious predator treatment (C) showed a marked decrease in the # of spots Summary: Phenotypes evolved in response to changes in the balance of sexual and predation selection Endler’s experiments 2.0: Field transplant in Trinidad Each population has an adapted phenotype, reflecting the local balance of sexual and predation selection. If evolution was “replayed”, the same adapted phenotype would evolve Population 1 should evolve the same phenotypes as population 2 (site 1 = site 2) → In site 1, colour pattern converged on that of site 2 → Demonstration of natural selection in action → Natural Selection can act quickly! Some correlative evidence In some upstream sites, prawns are the only predators. There, guppies tend to have big red spots Fish can see red, prawns cannot, so males can show off to mates without attracting prawns → Experimental evidence John Endler collected guppies from streams with differing predator richness (1 - 7 spp.); bred them together in captivity to produce a unique population with mixture of spots different from nature Set up 10 replicates of mixed populations of 200 guppies in outdoor pools (greenhouse); allowed them to breed, then added predators (C. alta or R. hartii); 9 mo elapsed Popns in pools without predatory C. alta became brighter: more & larger spots, & bigger bodies (< 9 guppy generations) Popns in pools with C. alta* evolved towards greater drabness (fewer, smaller spots, less blue & red); body size declined Weak effects in R. hartii treatments* Males in predator-free pools were competing for attention of females; in +predator pools, natural selection reduced the intensity of M ‘advertising’ to F The conflicting pressures of evading predator & attracting F: In his greenhouse pool experiments, Endler found guppy spots changed according to the characteristics of the bottom sediments - If the sediments were small particles, & predators absent, guppy spot size tended to increase over generations; if particles were a similar size to the guppy spots, then spot size tended to decrease (→ conspicuousness) - But, if C. atla was present, guppy spots became smaller where sediments were small particles, & larger where sediments were large particles (→ inconspicuousness, crypsis) Seeing spots: beauty in the eye of the beholder? In low-predation environments males have many red or orange spots. Why red? Sandkam et al. (2015) compared field popns in Trinidad low-predation guppies invest more of their colour vision to detect red/orange coloration than high-predation guppies. Eyes of low-predation females have high levels of expression of 2 of the 9 types of opsin proteins– i.e those that detect red/orange pigment Consistent inter-population differences in frequency of alleles coding for each opsin detected (i.e. genetic diffs) - predation pressure ↓ age at sexual maturity; high-predation females reproduce earlier & at a smaller body size → Invest heavily in reproduction: more offspring per brood compared to similar-sized indivs in low-predation environments Experimental evidence: Resnick et al. (1997) transplanted guppies from ‘dangerous’ stream to ‘safe’ & guppy-free site above waterfall: after 4 y, maturation was delayed & reproductive investment reduced L18 Human Evolution: where did we come from? Order Primates: Lemurs, monkeys, apes & humans (Homo sapiens) Very successful globally 16 families 72 genera >230 species Many ‘subspecies’ - Mainly in tropics Mouse lemurs: - 24 species - nocturnal New species of orangutan: - 1350 ml – greater than other Homo spp. (except Neanderthals) Less robust postcranial skeleton - most gracile Homo spp. Relatively long limbs & short trunk – reflect origins in (warm) Africa (cf. crural index + neanderthals) Ecological transitions of modern humans Invention of agriculture in Middle East (Iraq) ~9,500 y BP = change in ecological niche Large-scale agriculture (started ~7,500 BP) This led to a dependence on cultivation (by 5,300 BP) & relative food security Eventually led to the development of complex societies, civilization + (eventually) urban mode of life BUT, this ‘simple’ story of a linear progression has become more complex in light of reinterpretations & new discoveries... - 1.98 my BP Australopithecus sediba was found in S. Africa (2008), with hands & brain (420 ml) somewhat human-like but with A. africanus features, raises more questions about Homo direct ancestors New Homo species announced in Sept 2015* Homo naledi (15+ indiv) found ‘buried’ in Rising Star Cave in South Africa Small brain: 560 ml , 465 ml Long curved fingers & skeleton similar to A. afarensis Human-like foot Status is currently controversial (2017 dating suggests may be 230-330K y BP) Out of Africa - Homo ergaster: Afrca & Europ; 1.8mya - qmya - H. heidelbergensis: Africa & Europe; 800-300kya - H. erectus: East Asia L20 Anthropocene Out of Africa Small amount of genetic variation in modern humans indicates we are descended from an initial African population of ~10,000 people Evolution of modern humans 250,000 years Childhood is costly (takes time) → But results in more capable adults → Selection for a longer life span (**Correlated with brain size in mammals) ∵ Individuals gain more benefit from what was learned during (costly) juvenile period Larger brains in humans: may lead to qualitative differences E.g. Humans vs apes - More advanced linguistic communication - More developed or advanced culture - More control over our environment - More developed reciprocal altruism (we are expected to help others) What selective pressures favour large brains? Social Intelligence Hypothesis: Social or ecological pressures arising from group interaction (reciprocal altruism, etc.) Ecological challenges of finding food (fruit ripeness, location, timing) & use of extracted foods (nuts, tubers) that require processing Behavioural flexibility to invent or learn new solutions (e.g. tools) & learn from others Evolution of language would intensify selection for increased brain size (→ +ve feedback) … but we are unsure when language evolved Language (Difficult to say — no fossil record) Language seems to be a human attribute only → importance of cultural transmission of information (speaking or writing). Language defined: “the cognitive aspect of human communication involving symbolic thinking structured by grammar” Grammar = unconscious rules of language that allow humans to speak & understand (& react correctly) to a word sequence even when individual words do not differ* (Linguistics call these ordering rules syntax) “The dog bit your sister” (→ concern, anger, revenge) “Your sister bit the dog” (→ alarm) Do animals have language? Animals can convey emotional state & limited information (e.g. honey bees), but not complex information or ideas Chimps can learn phrases using American sign language (i.e. associate things with arbitrary symbols); can’t speak or learn grammar What can animals communicate? African vervet monkeys give distinct calls when they detect different predators, & react differently according to the call → Still respond to recorded calls in absence of predator Communication & culture Definition: “totality of behaviour of a social group that is passed down the generations by learning & symbolic means” - defining human characteristic - information learned by individuals through imitation, teaching & learning = inheritance of acquired traits (cf. Lamarck). Language greatly facilitates this - includes systems of behaviour, beliefs & technologies characteristic of a group & transmitted by its members Examples of cave/rock art from outside Europe (up to 40 kya) Culture in humans vs other animals * we are not the only species that has culture Animal culture Human culture The behavior must be practiced by more ‘advanced’ because it exhibits multiple members of the community cumulative change – increases as It must vary between societies each new generation’s innovations The potential for that same added to sum of knowledge behavior must exist in other societies E.g. Macaques washing sweet potatoes in seawater Orangutans use sticks to extract food → Cultural adaptation allows us to respond to environmental change much faster (& more effectively) than biological (genetic) adaptation e.g. Tools, clothes, hunting skills - wider geographic range than any other primate → Rapid acquisition of information about environment → Allows us to better control our environment e.g. Agriculture = control food supply - Use of non-food energy (fossil fuels) changes relationship with environment → GREATLY increase capacity to exploit it - Medical technology reduces deaths (+inc. births) Beginnings of Agriculture North America & Asia → These areas had the right climate & wild species that are the precursors to many of our staple modern crops, including wheat, rice and corn Ecological transitions of modern humans Invention of agriculture led to a transition from Palaeolithic (hunter- gatherer) to Neolithic life-styles = change in ecological niche - Large-scale agriculture (started ~7,500 BP) → dependence on cultivation (by 5,300 BP) & relative food security → complex societies, civilization + (eventually) urban mode of life Industrial Revolution Products were 1st mass-produced Fossil fuels were 1st widely used for power → By the beginning of the Industrial Revolution, the human population had increased to 1.7 billion (about 17 times greater than it was 10,000 years before) Has our biological evolution ended? Medical science has reduced death rates → reduce intensity of NS BUT NS acts on fitness differences Some evidence that NS on modern humans → decrease in age at first birth + increase age at menopause ∴ ↑ reproductive period* (+ other changes**) Cultural Evolution NS not ceased, but effect of inherited genotype on survival + breeding chances must be less than in the past memes = Culturally-transmitted beliefs & values NS applies to memes in a similar way as for genes: memes compete for our attention or memory, & some will be passed on, some not (some memes spread, others lost) Cultural adaptation vs maladaptation - Cultural & transmission of memes give huge evolutionary benefit for humans → we don’t need to work out everything for ourselves - Disadvantage: learning from others (& culture) require ppl to be credulous – i.e. belief that other ppl do things in a sensible way - Credulousness has disadvantage: it make us vulnerable to maladaptive/ incorrect beliefs & behaviors e.g. prejudice/ xenophobia - Memes can spread even if they reduce evolutionary fitness (e.g. popularity of cigarettes/ dangerous drugs) ∵ cultural transmission is rapid The Anthropocene Rapid biodiversity loss Climate change Changes to the nutrient cycle Changes to the distributions of plants and animals Invasive species Nocturnality A new geological epoch? There has been a lot of debate about if ‘Anthropocene’ is really an epoch Recent study of lake sediments show that in the 1950’s there is a clear signal of human activities Plutonium levels at the bottom of the lake can be used as a marker Range of dates suggested for when the Anthropocene started A growing human population - Current population of earth 8.2 billion, at 0.83 % growth rate - inc. by 83m/yr → overconsumption? → mass extinctions Impacts on megafauna (animals >45 kg), exterminated by human hunters (+ burning/fires & diseases + rats) Occurred ~50 kya in Australia (evidence of humans from >40 kya, maybe 55 kya), & New World ~12 kya (N→ S), Madagascar ~2000 ya, & New Zealand 800 ya Modern extinction rates - Current rates of extinction are 1000 – 10000 x higher than the geological average - Species (especially non-vertebrates) are lost before they are discovered - Difficult to estimate how many species are going extinct Climate change - Global temperatures have increased by 1˚C over the last 100 years - Predicted increase 2.5-5˚C over the next 100 years - Melting ice caps and glaciers, sea level rise - Increased extreme weather events (drought, typhoon, heat waves) - Shifting climates (changes to rainfall) - HONG KONG: highly urbanized → heat island Double no. of extreme hot days More extreme weather events Increased diseases e.g. malaria Biodiversity decline → We MUST limit emission rapidly to avoid extreme climate change