BIOL 2023 Midterm 2 PDF - Enzymes
Document Details
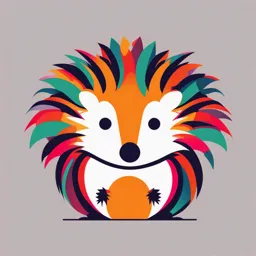
Uploaded by CozyGroup
2024
Tags
Summary
This document covers the topic of enzymes, including their role as biological catalysts, mechanisms and impact on reaction rates. It details how enzymes lower activation energy and stabilize transition states.
Full Transcript
Wed Oct 9 2024 Enzymes Bacterial Restriction Endonucleases What are enzymes? enzymes are biological catalysts usually proteins but RNA can also act as enzymes under specific conditions as well catalysts are compounds that increase the rate of reactions without being consu...
Wed Oct 9 2024 Enzymes Bacterial Restriction Endonucleases What are enzymes? enzymes are biological catalysts usually proteins but RNA can also act as enzymes under specific conditions as well catalysts are compounds that increase the rate of reactions without being consumed in the reaction the rate of chemical reactions depend upon the concentrations of participants (reactants and products) and the activation energy required for a reaction to occur in biological systems, the concentrations of reactants are usually very low and significant activation energy barriers exist. Therefore, most biochemical reactions would occur too slowly to permit life in the absence of catalysts. enzymes increase the rate of reactions by addressing low reactant concentrations and high activation energies Enzymes do not alter reaction equilibria “An enzyme cannot alter the laws of thermodynamics and consequently cannot alter the equilibrium of a chemical reaction” Stryer that birds to enzyme -reactant consider the reaction of Substrate (S) to Product (P): S P Note that with or without enzyme present the same amount of product will be formed in a chemical reaction. Enzymes increase reaction rates Note that in the enzyme catalyzed reaction, the rate of product formation levels off with time. This is because the reaction has reached equilibrium: substrate is still being converted to product but product is also being converted into substrate at a rate such that the product remains constant. Suppose in a chemical reaction that: - rxn rate the rate of product formation (forward reaction) (k1) = 1 x 10-4 mol/s and the rate of substrate formation (reverse reaction) (k-1) = 1 x 10-6 mol/s 10-4 s-1 this side - S would be P favored 10-6 s-1 · equilibrium The equilibrium constant for the reaction is given by the ratio of the rate constants [P] 10-4 Keq = = = 100 [S] 10-6 in other words, when the reaction is at equilibrium the ratio of product to substrate is 100:1 the presence of an enzyme catalyzing the reaction will not alter this ratio (equilibrium). but it may reduce the time to reach this equilibrium from hours or centuries to seconds or a fraction of second into dsDNA nucleotides cut ↓ - -> takes a Long time ↓ DNA S verable many reactions take hours, years, or thousands of years to proceed to completion (equilibrium) but enzymes can increase rates millions of times orotidine monophosphate conversion to uridine monophosphate by OMP decarboxylase half-life of OMP in absence of enzyme = 78 million years half-life of OMP in presence of enzyme = 18 milliseconds OMP bound in the active site of OMP decarboxylase enzyme OMP note amino acid sidechains forming intermolecular interactions with the OMP molecule functional groups I on Enzymes increase the Rate of Reactions by decreasing activation energy many of the reactions that occur in cells will occur spontaneously (-ΔG) but occur too slowly for life processes consider the oxidation of glucose C6H12O6 + 6 O2 → 6 CO2 + 6 H2O ↳ favoured ~ big -> Strongly Faloued to RIS the ΔG is about -2872 kJ/mol and a large amount of heat is liberated in this spontaneous reaction. The equilibrium for this reaction is far on the right side of the above equation because thermodynamically, the products are less energetic and therefore more stable and less ordered. The presence of glucose is not favored in this universe (at least in the presence of oxygen) yet a bottle of glucose can sit on a shelf for hundreds of years and not become substantially oxidized. An enzyme can quickly bring about the oxidation of glucose however by lowering the energy barrier to the forward (oxidation) reaction tends toward lower energy - transition state , ↓U does it change, ⱡ formof molecule from so equilibrium doesn't being commerced reactant toproduct change just gets , unstable-high energy( ⱡ thequicken bx very WellEa C6H12O6 C6H12O6 O2 O2 CO2 CO2 H2 O H2 O Note that a spontaneous reaction will proceed where the products have less energy than the reactants (substrate) and ΔG is the free energy change between the product and the reactant. Note that the enzyme does not alter the free energy change in a reaction which is why it does not alter the equilibrium associated with a reaction - only the rate. Enzymes lower Activation Energy by stabilizing a Transition State intermediate the free energy difference between reactants and products determines the equilibrium for a reaction but enzymes determine how quickly the equilibrium is reached by helping to form a transition state chemical form that is no longer reactant (substrate) but not yet product in structural terms S Xⱡ P where ⱡ denotes transition state The transition state is a high energy and unstable intermediate that must form during the conversion of substrate to product. It has a very short half-life (about 1 x 10-13 s). Its high energy is the source of activation energy (ΔGⱡ). Enzymes lower activation energy by providing an alternative reaction pathway toward formation of a more stable transition state. Enzymes lower activation energy by providing an alternative reaction pathway toward formation of a more stable transition state. (see upcoming lecture) when two chemicals react, the ability to form a transition state and eventual product relies on three main conditions: the concentrations of the reactants ↑ Conc Prate of cohisions the kinetic energy of the colliding substrate molecules theythat -> so transition state needto to uchl forms the orientation of the colliding substrate molecules * functional groups need to come into contact adding an external source of heat energy is one way to provide the required kinetic energy to substrate molecules such that they are able to form the transition state an enzyme reduces activation energy by binding two reactants, bringing them physically together in a orientation that facilitates reaction and by providing an alternative mechanistic pathway toward transition state formation. This occurs in the ACTIVE SITE of the enzyme. Substrates bind in the active site of an enzyme a relatively small portion of an enzyme is involved in catalysis the active site accommodates only specific substrates on the basis of size, shape, and chemical characteristics of the substrate the induced fit model describes that both the structure of the enzyme and the substrate is changed upon binding Amino acid side-chains interact with substrate molecules through weak forces such as ionic, hydrogen-bonding, and van der Waals interactions. it is these inter-molecular interactions that determine a) binding specificity, b) the orientation of substrate molecules in the active site, c) stabilization of the transition state structure, d) chemical reactivity or catalysis. During the catalytic process, covalent bonds may also form between the enzyme and transition state. **the enzyme binds the transition state form most strongly Active site amino acid side chains specifically interact with substrate molecules as shown in the active site of Ribonuclease (an enzyme that hydrolyzes RNA molecules to free ribonucleotides). We will talk about the mechanism by which enzymes catalyze chemical reaction later substrate-enzyme and transition state-enzyme interactions usually involve functional groups on amino acid side chains in the active site but can also involve main chain groups as well Catalytic amino acids reside in the active site the active site binding pocket or cleft is where several amino acid side chains that play instrumental roles in chemical catalysis coincide these are called active site residues or catalytic residues Naturally, the tertiary state of a protein is not a random structure. - C-terminus Protein Evolution as species diverge from one another, mutations will inevitably and independently occur in the genes that encode proteins. some mutations may be lethal and the host organism dies other mutations are permitted, leading to divergence amongst the amino acid sequences of like-proteins from different species. common -shar ancestor - Here are 4 homologous proteins from 4 different bacterial species. These four proteins carry out the same function in each bacterium. 1 N - MYFGHATFRVVARFPLKMNKYTWYHCNPCKACIYTGDEGGAKIADENS - C 2 N - MDKWAKWFMVAARGLCADDKLAQRHVQLCQACKMAADELNWEIALPAV - C 3 N - MSVKLGCWQVLARDEDSTGKAFERHVEICQACKLMADEAGSEIAYCVV - C 4 N - MDEWAVNFMVVARHLAADQRLANRHVADCQACKAWADDKQMEIVFQAV - C Note that not all amino acids have similar importance in the protein. Some are important for structure (pink) and some are active site catalytic residues (blue) and these are largely conserved. Some amino acids at specific positions are not conserved at all, indicating their precise identity is not overtly important to protein structure or enzyme activity 1 N - MYFGHATFRVVARFPLKMNKYTWYHCNPCKACIYTGDEGGAKIADENS - C 2 N - MDKWAKWFMVAARGLCADDKLAQRHVQLCQACKMAADELNWEIALPAV - C 3 N - MSVKLGCWQVLARDEDSTGKAFERHVEICQACKLMADEAGSEIAYCVV - C 4 N - MDEWAVNFMVVARHLAADQRLANRHVADCQACKAWADDKQMEIVFQAV - C Non-conservative substitutions Conservative Strictly substitutions Conserved ↳ mutation can happen but ↳ these ones do something important side chains must be similar no mutation can happen here enough to do the same chemistry Factors impacting conservative substitution: -size of side chain (spatial volume) -chemical attributes of side chain It is not uncommon for D and E to be interchangeable, but depending on context, sometimes even these cannot substitute for one another Some Enzymes possess co-factors required for either structure or catalysis some enzymes function on their own while some have co-factors intimately associated with their structure -> not proteins co-factors may be metal ions or larger molecules called co-enzymes or prosthetic groups both of these often participate in the catalysis process co-enzymes are often vitamin derivatives A Holoenzyme forms when the Apoenzyme form of the protein binds its co-factor Some selected co-factors (coenzymes and metal ions) Vitamins Co-Factors pre-cursor to are for enzymes that require co-factors, the protein lacking the co-factor is inactive this is the central reason why vitamins and certain metal ions are essential for life Thiamine, Vit B1 Riboflavin, Vit B2 Niacin Vitamin B6 Pantothenic acid Vit B12 Folic acid the 5 essential ions Ca2+, K+, Na+, Mg2+, Cl- The catalytically important amino acids and the co-factors define the active site of the enzyme and interact with substrate and the transition state (especially) When products are formed, interactions are lost and the products diffuse from active site cofactors are required When an enzyme can't do things by Active site amino acid side chains of the enzyme Cytochrome P450 themselves and its co-factor Heme interacting with its substrate camphor There are 6 Classes of Enzymes Transferase (32.5 %) Oxidoreductase (18.6 %) 1. Oxidoreductase 2. Transferase 3. Hydrolase 4. Lyase 5. Isomerase Ligase (10.9 %) 6. Ligase Hydrolase (22.4 %) Isomerase (5.6 %) Lyase (9.9 %) Oxidoreductase (e.g. alcohol dehydrogenase) oxidation-reduction reactions. 1 or 2 electron transfer reactions - corresponding change in redox rXn H or O atoms on molecule ethanol is oxidized to acetaldehyde Transferase (e.g. glucose kinase) transfer molecular groups from donor to acceptor molecules. Groups may be carboxyl, methyl, amino, phosphoryl, carbonyl, acyl groups. first step of glycolysis ADP Hydrolase (e.g. protease) bonds blu cleavage of bonds such as C-O, C-N, O-P break peptide accomplished with the addition of water. amino acids Lyase (e.g. pyruvate decarboxylase) reactions in which groups (e.g. CO2, H2O, NH3) are removed to form a double bond or are added to a double bond Isomerase (e.g. alanine racemase) one reactants one product catalyze intramolecular rearrangements ~ change bonding arrangement Ligase (e.g. pyruvate carboxylase) catalyze bond formation between substrate molecules at expense of ATP or similar molecules Summary Points -enzymes increase reaction rates but do not affect reaction equilibria -enzymes increase reaction rates by lowering activation energies -enzymes lower activation energies by promoting formation of the transition states -increase local concentration of reactants in a favorable orientation (proximity effect) -provide an alternative reaction pathway toward formation of the transition state -stabilizes the transition state Next few lectures we will cover: Enzyme kinetics (the study of the rate of enzyme catalysis) Catalytic mechanisms of enzymes Reaction mechanisms of selected enzymes How enzyme activity can be inhibited friOct 11 Enzyme Kinetics I Enzyme Kinetics What is it? Enzymes are molecular machines – as with any machine or mechanistic process in nature, it is worthwhile to understand: the individual steps taken by the machine in its process the rate at which the machine can operate the environment in which the machine best operates forward -> rates Y k1 k2 + + k-1 k-2 - 4 revere Enzyme-Substrate Enzyme Substrate Complex Enzyme Product (E) (S) (ES) (E) (P) Enzyme kinetics is a quantitative approach to understanding the functions of and mechanisms employed by enzymes. It is based upon determining the rates at which enzymes catalyze reactions and the environmental factors that influences these rates. Why analyze enzyme kinetics? 1. The kinetic analysis of enzyme behavior lends insight into the molecular mechanisms used by enzymes to convert substrate to products. 2. Kinetic analyses provide both the experimental means and strategy towards understanding readily measurable quantities that can be used to describe enzyme behaviour. Quantities derived through enzyme kinetics are the universal language by which enzymes are compared by biochemists. specificity constant Why analyze enzyme kinetics? 3. Enzymes are used in many industrial processes or constitute a component of useful products. Useful enzymes are identified by their kinetic properties e.g. their affinity for substrate and the efficiency with which they carry out reactions. Laundry Detergents remove stain Food stains 2) Oils/fats/lipids Lipases -> Proteins - Proteases Food Science Invertase …and thousands of other enzymes Why analyze enzyme kinetics? 4. In medicine: many chemicals living organisms may ingest either as therapeutic/recreational drugs or as toxins mediate their effects by binding to enzymes and altering the rate at which they catalyze reactions. antibiotics, pain killers, and thousands of others ethanol Amoxicillin nicotine tetrahydrocannabinol (THC) psilocybin Lysergic acid diethylamide (LSD) 3,4-Methylenedioxymethamphetamine (MDMA) venoms, toxins, poisons THC 5. The affinity of an enzyme for substrate and the rate at which it converts substrate to product is a consequence of its evolved role in the metabolic pathways that define life. The kinetic analysis of enzymes preceded knowledge of their molecular nature pre 1900 Pasteur posited that the special activities of living cells that could mediate chemical changes were a special property of life, inseparable from life. The concept of Vitalism. around 1900 Eduard Buchner discovered that these chemical activities were the properties of molecules that could function separate from living organisms. The term “Enzymes” was coined and over the next few decades it was established that these molecules were proteins. post – 1900 the emergence of theories that described the action of enzymes and their behavior The rate of an enzyme catalyzed reaction (v) is dependent on substrate concentration [S] reaction rate, v ↑ substrate ↑ Speed of rX substrate concentration, [S] Led Victor Henri in 1903 to propose that reaction proceed via an interaction between enzyme (E) and substrate (S). ES complex in 1913, Leonor Michaelis and Maud Menten proposed a general theory of enzyme activity: fast slow k1 k2 E+S ES E+P k-1 k-2 IVmax = km Vmax reaction rate, v ax Vmax[S] y= v= b+x Km + [S] Vmax is the maximum velocity of the rate of reaction that occurs when enzyme is saturated. KM is a measure of the apparent KM affinity of the enzyme for substrate substrate concentration, [S] Vmax and KM are the quantities that can be experimentally determined -derivation of the M-M equation from fast slow k1 k2 Vmax[S] to v= E+S ES E+P Km + [S] k-1 k-2 can lend insight into the meanings of these parameters Derivation of the M-M equation fast slow k1 k2 E+S ES E+P k-1 k-2 ↑ bl it out take product reaction rates are always measured no approximation yet as initial velocities, before there is appreciable depletion of substrate see end or formation of product of lecture fast slow I rate limiting Step k1 k2 bIc it's slow E+S ES E+P k-1 -the overall rate of rx (v) is limited by the conversion of ES → E + P -2 factors influence this conversion: rate constant (k2) and concentration of ES, [ES] rate of ren ~overal therefore, v = k2[ES] Equation 1 fast slow k1 k2 E+S ES E+P k-1 Two important assumptions are made during M-M analysis: 1) substrate is in vast excess i.e. [S] >>[E] 2) steady-state assumption, that ES is forming and breaking down at same rate. recenzyme ~Greenzymes substrate- ~ product k1[E][S] = k-1[ES] + k2[ES] formation breakdown collect terms on right side equation k1[E][S] = (k-1 + k2)[ES] solve for [ES] k1[E][S] [ES] = (k-1 + k2) next page fast slow k1 k2 E+S ES E+P k-1 k1[E][S] [ES] = since all 3 rate constants are on same side of equation, we can combine these into one term (k-1 + k2) anfinitentat enzyme has >WD for strate k1 1 (k-1 + k2) = I KM or KM = KM is called the Michaelis constant (k-1 + k2) k1 substitute 1/KM into above equation [E][S] [ES] = KM Equation 2 next page fast slow k2 [E][S] k1 Equation 2 [ES] = E+S ES E+P KM k-1 the total amount of enzyme, ET, is constant throughout experiment (i.e. the catalyst is not consumed). But it may be either free (not bound to substrate), E or bound to substrate, ES. therefore, [ET] = [E] + [ES] we can solve for [E] [E] = [ET] - [ES] and substitute this definition of [E] into Equation 2, so ([ET] – [ES])[S] [ES] = KM next page fast slow k1 k2 E+S ES E+P k-1 ([ET] – [ES])[S] [ES] = multiply through by [S] KM [ET][S] – [ES][S] [ES] = solve for [ET][S] KM [ET][S] = [ES]KM + [ES][S] collect terms [ET][S] = (KM + [S])[ES] solve for [ES] [ET][S] [ES] = KM + [S] next page fast slow k1 k2 E+S ES E+P k-1 [ET][S] remember Equation 1: v = k2[ES] [ES] = then KM + [S] v solve for [ES] substitute term for [ES] [ES] = k2 v [ET][S] = solve for v k2 KM + [S] k2[ET][S] v = KM + [S] Now, the maximum rate (Vmax) occurs when all enzyme bound to substrate. That is, when [ET] = [ES] Under this condition, Equation 1 becomes: Vmax = k2[ET] this ou substitute in Vmax Don't need to do Vmax[S] test v= the Michaelis-Menten equation KM + [S] The Significance of Vmax, KM and other kinetic quantities Vmax reaction rate, v ax y= b+x Vmax[S] v= Km + [S] KM substrate concentration, [S] Vmax fast slow k1 k2 E+S ES E+P k-1 k-2 reaction rates are always measured approximation as initial velocities, before there is appreciable depletion of substrate or formation of product fast slow k1 k2 E+S ES E+P k-1 In general the rate of a reaction is dependent on the rate constant k2 and the substrate concentration. Therefore v = k2[ES] but, when substrate concentration is so high that all of the enzyme is saturated then the enzyme is operating as fast as it can and Vmax = k2[ET] where ET is the total Enzyme in the reaction ([ES]=[ET] when the enzyme is saturated). Vmax is the maximal velocity of the enzyme KM - affinity enzume has for substrate KM is the substrate concentration at which the enzyme rate is half its maximal velocity (Vmax) or put another way: KM is the substrate concentration where the enzyme is half-saturated. Vmax reaction rate, v ½ Vmax Vmax[S] v= Km + [S] KM substrate concentration, [S] On a Michaelis-Menten plot, KM equals the [S] at which the reaction rate is half-maximal (1/2 Vmax) KM Vmax[S] reaction rates are always measured v= as initial velocities, before there is KM + [S] approximation appreciable KM is actually a combined rate constant that describesdepletion of substrate the formation and breakdown of the ES complex or formation of product fast slow k1 k 1 k2 (k-1 + k2) k1 1 1 = E+S (k ES = KE + Por KM = (k-1 + k2) KM k-1-1 + k2) M k1 in most reactions, k2 is usually much lower than k1 therefore KM approximately reduces to: ↓km affinite ↑ of enzyme k-1 substrate for KM = k1 Note that when k1 > k-1, the formation of ES is favored and KM is small when k-1 > k1, the dissociation if ES is favored and KM is large that is, small KM indicates high affinity of enzyme for substrate high KM indicates low affinity of enzyme for substrate KM is a measure of the affinity of an enzyme for its substrate Kcat fast k1 slow k2 E+S ES E+P k-1 kcat is equivalent to the rate limiting step, e.g. k2 One can calculate kcat therefore, k2 = kcat by experimentally determining Vmax and and when all enzyme is bound by substrate, knowing how much total enzyme (ET) is Vmax = k2[ET] used in the enzyme Vmax assay therefore, k2 = = kcat [ET] ↑ Kat speed res me kcat is also referred to as the turnover number (units of s-1) that is, the maximum number of substrate molecules per active site converted to product per second – the higher the Kcat, the faster the enzyme kcat is the turnover number also known as the Specificity constant kcat the parameters of KM or kcat alone are insufficient for comparing KM the overall efficiency of an enzyme a better measure is to calculate the ratio of kcat to KM it is a measure of the overall efficiency of the enzyme where ultimately every reaction relies on an encounter of Enzyme with Substrate the upper limit of kcat/KM is defined by the rate at which E and S can diffuse together in an aqueous solution (about 108 to 109 M-1s-1) some enzymes have specificity constants approaching this value they are said to be “catalytically perfect” enzymes Enzyme Substrate Kcat KM kcat/KM (s-1) (M) (M-1s-1) Catalase H2O2 more 4 x 107 1 x 100 4 x 107 active more -lactamase penicillin 2 x 103 2 x 10-5 more 1 x108 affinity catalytically efficient Practical Determination of Kinetic Parameters in Laboratory Hypothetical case: You work for an industrial company that uses the enzyme Cellulase in the formulation of laundry detergents. It is your job to determine the kinetic attributes of 5 different Cellulase enzymes to assess their suitability for use as an additive in laundry detergent. Background Cellulase is an enzyme that breaks down Cellulose into glucose molecules Energy O2 CO2 + H2O glucose cellulose glycosidic linkages Cellulases are enzymes that bind cellulose and chemically cleaves it back to free glucose molecules cotton light laundry - detergent - lit e cuts the ⑧ bits new shirt wear and tear after cellulase treatment surface look dull Your job: researcher in a laundry detergent company laboratory Carry out kinetic analysis of 5 cellulase enzymes Cellulase 1 isolated from bacterium A Cellulase 2 isolated from bacterium B Cellulase 3 isolated from fungus A Cellulase 4 isolated from bacterium C Cellulase 5 isolated from fungus B -determine the most active enzyme of the five. Measure cellulase enzyme activity by increase in free glucose concentration The Progress curve of reaction at defined concentration of cellulose, [S]. Enzyme + cellulose → Enzyme + glucose Cellulase 1 y=mx+b m=d[glucose]/dt The rate (v) is the increase in glucose liberated [glucose] from cellulose over time slope of the line = initial rate or velocity (v) of the reaction Xinitiaiveway don’t confuse this with the M-M plot, which has axes time of rate vs [S] Determination of reaction rates at varying substrate concentrations Cellulase 1 multiple progress curves [S] v 1 0.001 etc. 2 0.002 [S]=4 3 0.004 [glucose] [S]=3 4 0.007 5 0.010 [S]=2 6 0.013 [S]=1 you eventually test high enough time [S] to saturate the enzyme Next, plot Rates vs [S] Plotting substrate concentration vs rate – The Michaelis-Menten curve Cellulase 1 Vmax[S] v= KM + [S] rate, v o o o o o o Vmax o o o o o o o ½ Vmax o o o o [substrate], [S] Now you know Vmax and KM KM for Cellulase 1 So, Having generated a Michaelis-Menten Plot specific for the Cellulase #1: You now know the Vmax for the enzyme. You can now determine KM for the enzyme since KM is the substrate concentration at 1/2Vmax Also, you can calculate Kcat since you know how much enzyme you used in the assays and Vmax Kcat = [ET] much Awe know how weused enzume ! Therefore you can also calculate the specificity constant since Specificity Constant = Kcat/KM Having completed this analysis for all 5 enzymes: Enzyme kcat KM kcat/KM (s-1) (M) (M-1s-1) Cellulase 1 4 x 104 1 x 10-2 4 x 106 Cellulase 2 1 x 102 4 x 10-4 3 x 105 Cellulase 3 2 x 105 4 x 10-2 5 x 106 Cellulase 4 4 x 101 4 x 10-3 1 x 104 Cellulase 5 2 x 103 2 x 10-5 1 x108 =most active enzyme Therefore, you would report that Cellulase #5 is the most active enzyme Note that Cellulase #1 has a higher turnover number (kcat) than Cellulase #5 But Cellulase #5 has a much higher affinity for substrate (KM). Overall, based on the specificity constant (kcat/KM) Cellulase #5 is the most active enzyme. Understand the meanings of Vmax the relationship between Vmax and KM the meaning of KM in terms of substrate affinity the turnover number, Kcat Specificity constant Kcat/KM the general process for determining Vmax, KM, Kcat and specificity constant in the lab fast slow k1 k2 E+S ES E+P k-1 To determine the kinetic characteristics of an enzyme you used 1 nmol/L of enzyme in a series of assays where you measured the rate of reactions as you varied the concentration of substrate in each assay (Table A). Estimate from a Michaelis-Menten plot approximate values for Vmax, KM, Kcat, and the specificity constant for this enzyme and substrate. Table 1. [S] (μM) v (μmol/L/min) 0 0 5 20 I ⑱ 10 50 - B 20 75 40 95 8 80 125 120 135. ⑤ 160 143 ⑧ 200 150 8 needto do unit conversions B Estimated: Vmax 150 __________ Ms-1 150 150MONIC/S 5x10"St " Kat= V inmov= - /x0-mol/L - 1. KM 75 51 Kcat _______s _________M_ 1.5x10 -1___ Spec 2x109 -1s-1__ at = O = 2x const ________M Recommended Reading 1. Recommended Text: Biochemistry: Concepts and Connections Chapter 8 (Especially section 8.3 Pgs 251-258.) 2. Stryer Biochemistry 8th Edition Ch 8 Enzymes: Basic Concepts and Kinetics Pgs 216-245. 3. Stryer Biochemistry 7th Edition Ch 8 Enzymes: Basic Concepts and Kinetics Pgs 219-248. Next Lecture: Enzyme Kinetics II (Enzyme Inhibition) Wed Oct 16 Enzyme Kinetics II Musca domestica The heavy metal Mercury (Hg) Last Lecture we examined how an enzyme can be characterized kinetically conducting reactions with fixed concentrations of enzyme and increasing concentrations of substrate determining rates of reaction (v) from these plotting rate vs [S] to yield Michaelis-Menten curve and inferring Vmax, KM, Kcat, and specificity constant from that This Lecture we will examine a linear transformation of the M-M plot use these curves to examine the effects of inhibitors on enzyme activity Enzyme + cellulose → Enzyme + glucose y=mx+b m=d[glucose]/dt The rate (v) is the increase in glucose liberated [glucose] from cellulose over time slope of the line = initial rate or velocity (v) of the reaction ~ initial velocity (rate) time Determination of reaction rates at varying substrate concentrations multiple progress curves [S] v 1 0.001 etc. 2 0.002 [S]=4 3 0.004 [glucose] [S]=3 4 0.007 5 0.010 [S]=2 6 0.013 [S]=1 time Next, plot Rates vs [S] Plotting substrate concentration vs rate – The Michaelis-Menten curve a rectangular hyperbola Vmax[S] v= KM + [S] rate, v o o o o o o Vmax o o o symptote-> estimation a o ↓ o linear transformation o o ½ Vmax o o o o [substrate], [S] KM Problem: Vmax can only be estimated from the curve Therefore: data can be linearly transformed which makes it easier to estimate parameters like Vmax and KM Transformation of the Michaelis-Menten Equation: Lineweaver-Burk Equation the Lineweaver-Burk transformation involves taking the reciprocal of the M-M equation Vmax[S] 1 KM + [S] v= = KM + [S] v Vmax[S] 1 KM [S] v = + Vmax[S] Vmax[S] 1 KM 1 the Lineweaver-Burk Equation v = + Vmax[S] Vmax 1 KM 1 1 v = + Vmax [S] Vmax Notice this is now in the form of the equation for a line: y = m x + b y=mx + b this transformation is sometimes called a double-reciprocal plot 1 v KM slope = Vmax 1 1 - ⑤ KM Vmax 8 when 1/[S] = 0, [S] is infinitely large and 1 therefore enzyme is saturated and operating [S] at Vmax. The y intercept is therefore 1/Vmax. negative 1/[S] values are an extrapolation. 1 KM 1 1 v = + Vmax [S] Vmax y = m x + b [S] v 1/[S] 1/v 1 0.001 1 1000 2 0.002 0.5 500 3 0.004 0.33 250 4 0.007 0.25 143 5 0.010 0.2 100 6 0.013 0.17 77 Michaelis-Menten Plot Lineweaver-Burk Plot The Lineweaver-Burk* transformation was originally devised because it is difficult to ascertain the actual value of Vmax from a Michaelis-Menten plot now however computer programs can be used to fit kinetic data to the equation for a rectangular hyperbola and thus solve precisely for Vmax and KM therefore, Lineweaver-Burk transformations are rarely used for their original purpose however, they are still qualitatively useful for examining the effect that inhibitors have on the Vmax and KM characteristics of enzymes Now we will consider enzyme inhibitors and use Lineweaver-Burk plots to examine their effect on enzyme activities * Eadie-Hofstee and Hanes-Woolf plots are two other common transformations Molecules that inhibit enzymes are very important they regulate enzyme activity in the body but are also found in many other situations Poisons e.g. cyanide Toxins e.g. from poisonous mushrooms Therapeutic Drugs e.g. Antibiotics e.g. Viagara e.g. Ibuprofen and other painkillers and many thousands of others There are 3 main types of enzyme inhibition mechanisms inhibition is caused by molecules that bind enzymes and alter either the Vmax or KM of the enzyme + inhib 1/v 1/v S - inhib X S - inhib 1/Vmax I Enzyme Competitive Inhibitor 0 1/[S] 0 1/[S] Enzyme -1/KM + inhib I + inhib 1/v 1/v - inhib S S - inhib or Enzyme Uncompetitive Enzyme I Enzyme I Noncompetitive Inhibitor Inhibitor 0 1/[S] 0 1/[S] occurs when a molecule structurally mimics the Competitive Inhibition substrate and binds the active site. minisa the transamemesit S blocks (or competes with) the substrate for X binding to the active site I Note the slope of L-B plot is changed since slope = KM/Vmax, one of these values must have Enzyme changed afte Note that the y-intercept (1/Vmax) has not changed therefore Vmax has not been altered + inhib 1/v Note that it is the apparent KM that has been altered - inhib (the x intercept = -1/KM) Competitive so the apparent KM has been increased (note that the Inhibitor real affinity of enzyme for substrate has not been altered 0 1/[S] -just that more substrate needs to be added to compete with inhibitor and saturate the enzyme adding more substrate will outcompete the inhibitor and the Vmax can be attained Comparing effects of competitive inhibitor using M-M plot and L-B plot adenosine Adenosine regulates sleep: upon prolonged wakefulness, adenosine levels rise and promote sleepiness by interacting with neuron receptors Caffeine, which is a structural mimic (analogue) of adenosine, acts as a competitive inhibitor by blocking binding of adenosine to the adenosine A₁ receptor, which enhances release of the neurotransmitter acetylcholine. The most common herbicide in the world RoundUp – (Glyphosate) analog of phosphoenolpyruvate, binds to EPSP synthase so is a competitive inhibitor tyrosine precursor for phenylalanine tryptophan synthesis Aspirin Ibuprofen acetylsalicylic acid competitive inhibitor of the COX (cyclooxygenase) enzymes involved in synthesis of prostaglandins - fatty acid derived hormones that regulate inflammation and sensation of pain. Structure of COX-1 sildenafil bound to Viagara (Sildenafil citrate) phosphodiesterase competitive inhibitor of an enzyme called cGMP-specific phosphodiesterase that regulates blood vessel dilation and blood flow in tissue. Originally tested as a treatment for reduced blood flow in heart muscle which can lead to angina. It did not work well. However, male volunteers reported unusual side effects during clinical trials. The pharmaceutical company (Pfizer) took note. Sometimes side-effects can be valuable. By A2-33 - Own work, CC BY-SA 3.0, https://commons.wikimedia.org/w/index.php?curid=18444742 Uncompetitive Inhibition I the inhibitor does not bind the active site, but instead binds the ES, the enzyme-substrate complex, and prevents S the conversion of substrate to product Note the slope of L-B plot remains the same, therefore either there is no effect on either KM or Vmax (which Enzyme would mean that there is no inhibition) OR both have been changed. Note that the y intercept is increased, therefore Vmax + inhib has been decreased AND the x-intercept has increased 1/v - inhib therefore Km has decreased ↓km ↑ affinity I in this cage this isan illusion , blc when so this inhibition primarily results from the fact that the s bonds to maximal velocity of the enzyme is severely decreased - enzymegets it Uncompetitive trapped Inhibitor unlike competitive inhibition, this cannot be rescued by adding more substrate 0 1/[S] the decrease in KM (which should mean the enzyme now has a greater affinity for substrate) results from fact that the equilibrium shifts towards formation of more ES complex as more inhibitor binds. Effects of Uncompetitive Inhibitors on KM and Vmax reaction rates are always measured KM is actually aapproximation as initial combined rate constant that velocities, describes before there the formation andis breakdown appreciable depletion of substrate of the ES complex or formation of product fast rxn tends slow k1 k1 to here k2 (k-1 + k2) k1 1 1 = E+S (k ES = KE + Por KM = (k-1 + k2) KM k-1-1 + k2) M k1 in most reactions, k2 is usually much lower than k1 therefore KM approximately reduces to: k-1 since all enzyme is progressively trapped in the ES form, this mimics KM = an increase in k1 rate constant thus decrease in KM k1 when enzyme is saturated with substrate then the enzyme is operating as fast as it can and Vmax = k2[ET] and … Vmax k2 = = kcat [ET] since the ES complex is trapped by the inhibitor, k2 (i.e. kcat) becomes very low and Vmax is depressed and cannot be rescued by more substrate. Noncompetitive Inhibition the inhibitor does not bind the active site, but instead binds the enzyme elsewhere and can bind free enzyme or the enzyme-substrate complex. In either case, since the inhibitor inactivates the enzyme allosterically, the affinity for substrate (KM) is unaffected, but the Vmax decreases. S or Enzyme I Enzyme I + inhib 1/v so this inhibition primarily results from the fact that the maximal velocity of the enzyme is severely decreased - - inhib unlike competitive inhibition, this cannot be rescued by adding more substrate Noncompetitive Inhibitor 0 1/[S] Examples of common non-competitive Enzyme Inhibitors Voltage-gated Na channels regulate Na+ ion passage across axon membranes. Ingredients in insecticides (e.g. pyrethroids like permethrin) are noncompetitive inhibitors that trap the channel in an open conformation, preventing repolarization of the membrane. permethrin Factors influencing non-target organism intoxication - dose/exposure - absorption of drug - absence of target enzyme in non-target organism - detoxification systems Permethrin-based topical anti-flea drugs have been used for dogs but are very toxic to cats because they have inactive glucuronosyltransferase systems. Glucuronic acid addition to a hydrophobic molecule increases hydrophilicity and elimination through kidneys This is why you should never give drugs prescribed for humans or dogs to cats. cyanide binds heme co-factors and inhibits the enzyme complex Cytochrome c oxidase in a noncompetitive fashion LD50 of 1.52 mg/kg structure of cyanide anion November 18, 1978: 909 people die from consuming cyanide in Jonestown Guyana at the behest of a charismatic religious leader, Jim Jones. https://en.wikipedia.org/wiki/Jonestown#Deaths_in_Jonestown Amanitin a cyclic 8 amino acid peptide produced by several genera of mushrooms binds and inhibits RNA polymerase as a noncompetitive inhibitor reduces mRNA synthesis from a rate of 3000 nt/min to about 30 nt/min. Antabuse (Disulfiram) - chemical treatment for chronic alcoholism - noncompetitive inhibitor of enzyme acetaldehyde dehydrogenase By NEUROtiker - Own work, Public Domain, https://commons.wikimedia.org/w/index.php?curid=3962576 acetaldehyde dehydrogenase alcohol acetaldehyde dehydrogenase dehydrogenase ethanol acetaldehyde acetate (from Wikipedia) Disulfiram plus alcohol, even small amounts, produces: flushing, throbbing in head and neck, throbbing headache, respiratory difficulty, nausea, copious vomiting, sweating, thirst, chest pain, palpitation, dyspnea, hyperventilation, tachycardia, hypotension, syncope, marked uneasiness, weakness, vertigo, blurred vision, and confusion. Heavy Metals e.g. Pb, Hg, Cd, Cr, As can act as noncompetitive inhibitors of enzymes elemental mercury H3C – Hg – CH3 dimethylmercury https://en.wikipedia.org/wiki/Karen_Wetterhahn Inhibitor Type Effect Competitive Increased KM Vmax unchanged Uncompetitive Decreased Vmax Decreased KM Ratio of KM/Vmax unchanged Noncompetitive KM unchanged Decreased Vmax From this lecture you should: -understand that most (but not all) chemicals that have the ability to alter your physiology do so by binding and altering the activity of an enzyme. -understand various types of enzyme inhibition, how they affect kinetic parameters of an enzyme, and why and how inhibition becomes qualitatively obvious using Lineweaver-Burk plots Eri Oct 18 one water molecule-up to four bouds mother #20 molecules butane butanol propanol Ethanol Solubility in #o longer fatty asid higher melting point PI lowest to : highest From tarts on C8 fat alid , double bond is cis-5" coo- but omega-c is counted from other end Mon Oct 21 Enzyme Catalysis I: Reducing the Activation Energy Barrier by Promoting the Formation of the Transition State Great White Shark (Carcharodon carcharias) Enzymes - Biological Catalysts Usually proteins, some RNA molecules Do not alter equilibrium of a reaction Decreases the time it takes for reaction to reach equilibrium i.e increases reaction rate How do enzymes accelerate reaction rates? Ea I h stays thesame but Lower activation energy barrier changes : -Wh favourable Oh : + nonfavourable Thermodynamically enzume cannot favourable fr alter favourable favourability rXn - Oh ↑ ↑ temp Kinetic energy ↑ colliding ↑ rate of ~xh Chapter 8, Figure 8.3, Effect of increasing temperature or lowering ∆G° on the rates of reactions. The curves show Maxwell–Boltzmann distributions of kinetic energies for systems of molecules at lower and higher temperatures. How do enzymes lower activation energy? 1. Substrate Binding/Proximity Effect Today 2. Stabilization of Transition State 3. Providing alternate reaction pathways Major was -acid/base catalysis chemistr happens of inactive site -covalent catalysis Next enzyme Class -metal ion catalysis -electrostatic catalysis Note that these categories are not necessarily distinct. For example, acid/base reactions can act to stabilize the transition state. A brief read that summarizes what we are talking about over the next couple lectures https://en.wikibooks.org/wiki/Structural_Biochemistry/Enzyme_Catalytic_Mechanism/Catalysis fast slow k1 k2 Expanding the reaction E+S ES E+P coordinate scheme k-1 k-2 O e - transit = enzymes this drive rxn forward Enzyme-Substrate Binding enzyme catalysis begins with substrate binding to enzyme Enzyme-Substrate Binding binding energy is equivalent to the free energy released in the formation of a large number of non-covalent interactions between substrate and enzyme pressure of it's volume internal energy product -> system fenthalpy -entropy ΔG = ΔH -TΔS where a negative ΔG indicates a favorable reaction and increasing ΔS and decreasing ΔH favors - ΔG everything mattery - towards (disorder) Enzyme-substrate binding: unfavorable S favorable H binding energy serves two purposes: establishes substrate specificity and proximity binding interactions lower enthalpy thus compensate for the entropic cost “the loss of entropy associated with the restriction of rotation and translation of substrate must be compensated by the intrinsic energy of binding (formation of favourable non-covalent interactions) between substrate and enzyme” Strain in the substrate(s) can be a manifestation of induced fit increasing number of enzyme- substrate interactions distort both the substrate and the enzyme The Proximity Effect (catalysis by approximation) Rates of reaction are in part related to substrate concentrations k1 A+B C+D where rate = k1 [A][B] k-1 In multi-substrate reactions, binding of substrate molecules to enzymes increase their local or effective concentration In single substrate reactions, the substrate molecule may be contorted to bring reacting functional groups in the molecule in closer proximity to each other In a non-catalyzed reaction, substrate molecules may possess sufficient energy to react upon collision, but their spatial orientation is unfavorable. Enzymes orient the reacting molecules to promote reaction (transition state formation). - side chains The Proximity Effect (catalysis by approximation) then enzymes bring intra Specific consider these non-enzyme catalyzed reactions together orientation tomake th transition state easier to form 1x 1. bromophenol acetate/acetate 2. 1000x 3. 200,000x fre rotateen ↑ Tether ran rate 50 4. million x Enzymes stabilize transition states all catalysts stabilize the transition state in a reaction transition states are unstable arrangements of atoms in which chemical bonds are in the process of being made or broken enzymes stabilize the transition state by making the maximum number of weak interactions with atoms in the transition state species Releases energy -> more stable that is, the enzyme interacts better with the transition state form than it does with either the original substrate(s) or the eventual product(s) Stabilization of the transition state lowers the activation energy required for product formation consider the reaction: bromomethane + hydroxide anions → methanol and bromine anions but neit are ve complete -> - u yet bond being bond being formed broken progress to product formation requires that the transition state be formed. many reactions proceed slowly in the absence of catalyst because formation of the transition state is infrequent Some reactions occur in multiple steps some reactions occur in multiple steps involving multiple transitions states and intermediates transition states are high energy, extremely unstable and short-lived (10-13 s). Intermediates are more stable than transitions states, can often be detected chemically, but still have a higher energy and are more unstable than either the reactants or the products Here, two lower energy barriers (transition states) replace one higher energy barrier Phosphoglycerate kinase (PGK) catalyses the seventh step in glycolysis and is the first enzyme in the pathway to produce energy, rather than consume it, by the synthesis of adenosine triphosphate (ATP). 1,3-biphosphoglycerate + ADP 3-phosphoglycerate + ATP 1,3-biphosphoglycerate ADP open conformation closed conformation http://www.youtube.com/watch?v=dM9MMmHlZJs bring together " ↑ I reactants free enzyme ES, open conformation ES, closed conformation two products transition state formation EP, open conformation EP, closed conformation http://www.youtube.com/watch?v=dM9MMmHlZJs Transition state (TS) analogues structurally mimic true transition states TS analogues are stable molecules that bind very strongly to enzymes because they mimic the transition state (bind more strongly than the reactant/substrate) though they are not perfect matches to the actual transition state, they give insight into what the transition state must look like TS analogs are useful for determining likely structural features of an enzyme when bound to the true transition state. TS analogs cannot be catalytically converted to product, therefore the enzyme is trapped in the TS analogue-bound state and is inhibited. many inhibitors of enzymes are TS analogues and fall into the category of competitive inhibitors (although not all competitive inhibitors are TS analogues) Competitive Inhibition subset S X very stor gly -binas substrate analogues or I transition state analogues can act as competitive inhibitors of Enzyme enzymes enzymes for 1/v + inhib change km -> afinity substrate - inhib affinity enzyme isn't really losing Competitive to add Inhibitor for substrate , you just have 0 1/[S] a bunch of substrate Transition state analogs are often potent enzyme inhibitors and have various therapeutic uses Cholesterol lowering drugs Statins (like Lipitor) are cholesterol lowering drugs because they are transition- state analog inhibitors of an enzyme called HMG-CoA Reductase, a key enzyme in the biosynthetic pathway for cholesterol. HIV protease inhibitors Three main HIV enzymes: Reverse Transcriptase -copies viral genome into double-stranded DNA Integrase -inserts viral genome into host (human) chromosomes Protease ->gene on were -processes viral proteins so they can assemble into new viruses destroys in mure system ↓ infection of some sort ends up Killing you Chapter 8 Pg 232 HIV protease structure Many drugs (such as Saquinavir) available for AIDS patients are protease inhibitors (protease transition-state analogs) http://www.youtube.com/watch?v=RO8MP3wMvqg How do enzymes lower activation energy? 1. Substrate Binding/Proximity Effect ↳bring reactants together into transition e Today 2. Stabilization of Transition State 3. Providing alternate reaction pathways -acid/base catalysis -covalent catalysis Next Class -metal ion catalysis -electrostatic catalysis Note that these categories are not necessarily distinct. For example, acid/base reactions can act to stabilize the transition state. The making and breaking of chemical bonds Electron Flow Proteins are composed of amino acids (and often co-factors such as co-enzymes and metal ions). Amino acid side chains are the main determinants of protein structure and function Hydrophobic side-chains play major roles in overall structure of protein hydrophobic effect van der Waals interactions Polar and ionizable side chains located in the active site of enzyme play major roles in catalysis Ionization involves proton loss or gain and about 75% of all reaction steps catalyzed by enzymes involve the transfer of a proton ↳ Why it's important to know i amino acid is protonated Seven amino acids have ionizable side chains serine - pla is so side chain high so in ionize a will never biological system ↳ but an enzy me can make that happen -many amino acids have important roles in the active site and may have accessory roles (eg. substrate/TS binding, stabilization) and might be called active site amino acids -some play a direct role in catalysis and these are called CATALYTIC amino acids. Often it is the amino acids with ionizable side chains that play a main role in catalysis Roles of amino acid in active site the phrases “proton transfer” and “covalent binding” imply CATALYTIC function cation and anion binding or hydrogen bonding implies accessory roles in the active site such as substrate binding and TS stabilization Frequency of amino acids acting as catalytic residues oneof the most commonalte site of enzu set the imidazole ring of Histidine Note Histidine: High frequency as catalytic amino acid pKa around 6-7 physiological pH is about 6.8-7.4 Remember that when pH = pKa of an ionizable group, both the protonated form and the unprotonated form have the same abundance (What equation could you use to demonstrate this ??) -> Henderson Hasslebach Recall that most chemical reactions involve the transfer of protons. This is why Histidine is such a common catalytic residue…a pKa poised at the physiological pH means that it can readily accept or donate protons during chemical reactions occurring in the cell. pKa values for ionizable groups on isolated amino acids Notice that environment can alter pKa values of amino acids pKa values for ionizable groups on amino acids in proteins an enzemamaking en t aspartate, glutamate nizable. histidine cysteine tyrosine lysine arginine serine Next day we will take a closer look at chemical reactions catalyzed by enzymes and the roles played by amino acid side chains in the active site. Wed Oct 23 Enzyme Catalysis II Charles Bell (1809) - Soldier during Napoleonic War dying from Lockjaw whose proteins is strate -enzume sub the The bacterium Clostridium tetani produces a protease called tetanospasmin (LD50 2.5-3 ng/kg) that catalytically degrades the human protein synaptobrevin and results in spastic muscle contraction. the 150 kDa tetanospasmin protein By Jawahar Swaminathan and MSD staff at the European Bioinformatics Institute Tetanus (Lockjaw) - protection provided by the DPT (Diphtheria-Pertussis-Tetanus) vaccine How do enzymes lower activation energy? 1. Substrate Binding/Proximity Effect Last 2. Stabilization of Transition State Day 3. Providing alternate reaction pathways -acid/base catalysis Today -covalent catalysis -metal ion catalysis -electrostatic catalysis Note that these categories are not necessarily distinct. For example, acid/base reactions can act to stabilize the transition state. The making and breaking of chemical bonds Electron Flow Proteins are composed of amino acids (and often co-factors such as co-enzymes and metal ions). Amino acid side chains are the main determinants of protein structure and function Hydrophobic side-chains play major roles in overall structure of protein hydrophobic effect van der Waals interactions Polar and ionizable side chains located in the active site of enzyme play major roles in catalysis Ionization involves proton loss or gain and about 75% of all reaction steps catalyzed by enzymes involve the transfer of a proton Roles of amino acid in active site the phrases “proton transfer” and “covalent binding” imply CATALYTIC function cation and anion binding or hydrogen bonding implies accessory roles in the active site such as substrate binding and TS stabilization Frequency of amino acids acting as catalytic residues the imidazole ring of Histidine Note Histidine: High frequency as catalytic amino acid pKa around 6-7 physiological pH is about 6.8-7.4 Remember that when pH = pKa of an ionizable group, both the protonated form and the unprotonated form have the same abundance (What equation could you use to demonstrate this ??) Recall that most chemical reactions involve the transfer of protons. This is why Histidine is such a common catalytic residue…a pKa poised at the physiological pH means that it can readily accept or donate protons during chemical reactions occurring in the active site of an enzyme. ↳ can act as acid or base pKa values for ionizable groups on isolated amino acids Notice that environment can alter pKa values of amino acids pKa values for ionizable groups on amino acids in proteins aspartate, glutamate histidine cysteine tyrosine lysine arginine serine Roles of Amino acid Side-Chains in Enzymes Non-polar hydrophobic side-chains Structural roles in protein e.g. Ala, Val, Leu, IIe, Phe, etc. Polar side-chains Proton transfer e.g. Asp, Glu, His, Lysine Substrate + TS binding thru charge-charge interactions e.g. Asp, Glu (neg), Lys, Arg (pos) Transient covalent bonds to substrate e.g. Cys, Ser Other roles Forming H bonds to substrate or other amino acids thus altering their pKa and propensity to ionize at certain pH note that the above roles for side-chains not absolute, for example lysine participates in a covalent mechanism in ligase (see DNA replication lecture) The Making and Breaking of Bonds in Chemical Reactions Nucleophiles, Electrophiles and Electron pushing Chemical reactions (the making and breaking of bonds) result from the movement of electrons from one molecule to another Many chemical reactions involve ionized substrate, intermediates or products Two important definitions: Nucleophilic molecules (Nucleophiles) electron rich, negatively charged or carry a non-bonding pair of electrons Electrophilic molecules (Electrophiles) electron-poor In chemical reactions, the nucleophile transfers electrons to the electrophile through bonding and is said to attack the electrophile through a Nucleophilic Attack (often a Nucleophilic Substitution reaction). Electron Pushing Diagrams the pathway of electron flow from nucleophile to electrophile can be shown using electron pushing diagrams arrows represent the movement of pairs of electrons Nucleophilic substitution reaction - nucleophile (Y) attacks electrophilic Carbon atom. Y substitutes X and X is the leaving group. Note all pairs of electrons conserved and accounted for. Electron pair forms transient negative charged Oxygen Double bond to oxygen broken as (oxyanion). Double bond is re- single pair of electrons attracted established. Arrow to mid-bond to electronegative Oxygen. indicates bond formation. Arrow from mid-bond indicates bond being broken. Electron pair joins X and negatively charged X leaves (is the leaving Nucleophilic attack-arrow from group). Arrow from mid-bond nucleophile (Y) to Carbon atom indicates bond breaking. indicates electrons donated by nucleophile and bond formed to Carbon Another Nucleophilic substitution reaction bromomethane + hydroxide anions → methanol and bromine anions bond being bond being formed broken Modes of Catalysis Acid-Base Catalysis -catalytic donation or acceptance of proton (hydrogen ion). Covalent Catalysis -where the active site contains a nucleophile (amino acid side chain group) that becomes temporarily covalently attached to the substrate. Metal Ion Catalysis -metal ions (e.g. Zn2+, Mg2+, etc.) may facilitate the formation of strong nucleophiles, may help stabilize transition states and reaction intermediates through charge-charge interactions, may help bind substrates in active site. Catalysis by Approximation -where reactants are bound in proximity to one another and in proper orientation to promote formation of transition state (the Proximity Effect). also Electrostatic Catalysis -where charges on TS and intermediates are neutralized by H bonding or interactions with charge functional groups or metal ions Chemical Modes of Catalysis Acid-Base Catalysis acceleration of a reaction achieved by catalytic transfer of proton most common event in enzyme catalyzed reactions relies on amino acid side-chains that can donate and accept protons at ambient pH, which might be physiological pH provides alternate route toward TS formation, neutralizes developing charges, stabilizes leaving groups With a pKa poised around physiological pH, Histidine is a frequent catalytic amino acid because it can readily donate or accept protons pKa ~6 Covalent Catalysis -substrate becomes temporarily covalently attached to active site amino acid side chain Covalent Catalysis nucleophilic amino acid side-chains can form a transient covalent bond with electrophilic group on substrate For example, certain proteases use Serine to hydrolyze peptide bonds in proteins generates an additional intermediate in the reaction pathway lowering overall energy of TS activesite X / reaction intermediate many enzymes use both general acid-base and co