BIOL 111 Fundamentals of the Nervous System PDF
Document Details
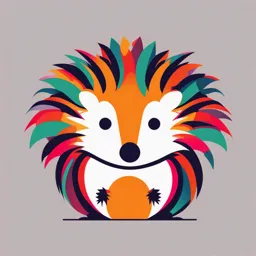
Uploaded by EffortlessPhiladelphia8629
Pamela Paynter-Armour
Tags
Summary
This document is a presentation on the fundamentals of the nervous system. It covers the organization, function, and structure of the nervous system, including neurons and neuroglia.
Full Transcript
BIOL 111: Fundamentals of the Nervous System Fundamentals of the Nervous System and Nervous Tissue 1 Instructor- Pamela Paynter-Armour Objectives Describe the divisions of the nervous system and their characteristi...
BIOL 111: Fundamentals of the Nervous System Fundamentals of the Nervous System and Nervous Tissue 1 Instructor- Pamela Paynter-Armour Objectives Describe the divisions of the nervous system and their characteristics. Identify the structures/functions of a typical neuron. Describe the location and function of neuroglia. Describe resting membrane potentials Discuss the events in the generation and propagation of an action potential. Define the structure/function of a synapse. Nervous System The master controlling and communicating system of the body Functions Sensory input –the stimuli goes to CNS Integration – interpretation of sensory input Motor output – response to stimuli coming from CNS (Collin College, n.d.). 3 Terminology Input: sensory = sensory input Receptors monitor changes Changes called “stimuli” (sing., stimulus) Information sent by “afferent” nerves Integration Info processed Decision made about what should be done Output: motor = motor output Effector organs (muscles or glands) activated Effected by “efferent” nerves (Los Angeles Valley College, n.d.). Nervous System (Los Angeles Valley College, n.d.). Organization of the Nervous System Central nervous system (CNS) Brain and spinal cord Integration and command center Peripheral nervous system (PNS) Paired spinal and cranial nerves Carries messages to and from the spinal cord and brain (Collin College, n.d.). 6 Peripheral Nervous System (PNS): Two Functional Divisions 1. Sensory (afferent) division Somatic sensory fibers – carry impulses from skin, skeletal muscles, and joints to the brain Visceral sensory fibers – transmit impulses from visceral organs to the brain (Collin College, n.d.). 7 Peripheral Nervous System (PNS): Two Functional Divisions 2. Motor (efferent) division Carries impulses from the CNS to effector organs- muscles and glands (Collin College, n.d.). 8 Motor Division: Two Main Parts 1. Somatic Nervous System (voluntary) - carry impulses from the CNS to skeletal muscles 2. Autonomic nervous system (ANS) (involuntary) - carry impulses from the CNS to smooth muscle, cardiac muscle, and glands (Collin College, n.d.). 9 ANS Division: Two Main Parts Sympathetic and Parasympathetic (Collin College, n.d.). 10 Central nervous system (CNS) Peripheral nervous system (PNS) Brain and spinal cord Cranial nerves and spinal nerves Integrative and control centers Communication lines between the CNS and the rest of the body Sensory (afferent) division Motor (efferent) division Somatic and visceral sensory Motor nerve fibers nerve fibers Conducts impulses from the CNS Conducts impulses from to effectors (muscles and glands) receptors to the CNS Somatic sensory Somatic nervous Autonomic nervous fiber system system (ANS) Skin Somatic motor Visceral motor (voluntary) (involuntary) Conducts impulses Conducts impulses from the CNS to from the CNS to skeletal muscles cardiac muscles, Visceral sensory fiber smooth muscles, Stomach and glands Skeletal muscle Motor fiber of somatic nervous system Sympathetic division Parasympathetic Mobilizes body division systems during activity Conserves energy Promotes house- keeping functions during rest Sympathetic motor fiber of ANS Heart Structure Function Sensory (afferent) division of PNS Parasympathetic motor fiber of ANS Bladder Motor (efferent) division of PNS (Meeking, 2010). Histology of Nerve Tissue The two principal cell types of the nervous system are: Neurons – excitable cells that transmit electrical signals Neuroglias (glial) – cells that surround and wrap neurons (supporting cells) (Collin College, n.d.). 12 Supporting Cells: Neuroglia Neuroglia Provide a supportive scaffolding for neurons Segregate and insulate neurons Guide young neurons to the proper connections Promote health and growth Six types- 4 CNS, 2 PNS (Collin College, n.d.). 13 Neuroglia of the CNS Astrocytes Most abundant, versatile, and highly branched glial cells Maintain blood-brain barrier They cling to neurons and their synaptic endings, They wrap around capillaries Regulate their permeability (Collin College, n.d.). 14 Neuroglia of the CNS Astrocytes Provide structural framework for the neuron Guide migration of young neurons Control the chemical environment Repair damaged neural tissue (Collin College, n.d.). 15 Astrocytes (Collin College, n.d.). 16 Neuroglia of the CNS Microglia – small, ovoid cells with spiny processes Phagocytes that monitor the health of neurons Ependymal cells – range in shape from squamous to columnar They line the central cavities of the brain and spinal column (Collin College, n.d.). 17 Microglia and Ependymal Cells (Collin College, n.d.). 18 Neuroglia of the CNS Oligodendrocytes – branched cells Myelin Wraps of oligodendrocytes processes around nerve fibers Insulates the nerve fibers (Collin College, n.d.). 19 Neuroglia of the PNS Schwann cells (neurolemmocytes) – Myelin Wraps itself around nerve fibers Insulates the nerve fibers Satellite cells surround neuron cell bodies located within the ganglia Regulate the environment around the neurons (Collin College, n.d.). 20 Oligodendrocytes, Schwann Cells, and Satellite Cells (Collin College, n.d.). 21 Myelin in the Peripheral and Central Nervous Systems (Los Angeles Valley College, n.d.). Application In Multiple Scleroses the myelin sheath is destroyed. The myelin sheath hardens to a tissue called the scleroses. This is considered an autoimmune disease. Why does MS appear to affect the muscles? (The Washington Township Public School, n.d.) An Introduction to Neuroglia (Ireland, 2004). Neurons (Collin College, n.d.). 25 Neurons are the nerve cells, the structural and functional units of the nervous system. They conduct impulses that enable the body to interact with its internal and external environments. There are various types of neurons. (Nebraska Department of Education, 2017) Neurons (Nerve Cells) All have a cell body: with nucleus and cytoplasm Cell bodies are in clusters CNS: clusters called nuclei PNS: clusters are called ganglia (Los Angeles Valley College, n.d.). The Neuron Dendrites Cell body Nuclei of neuroglia 600x Axon Neurons (Nerve Cells) Structural units of the nervous system Composed of cell body, axon, and dendrites Long-lived (>100 years), amitotic, and have a high metabolic rate Their plasma membrane function in: Electrical signaling Cell-to-cell signaling during development (Collin College, n.d.). 29 Dendrites Cell body (receptive regions) (biosynthetic center and receptive region) Nucleolus Axon (impulse generating and conducting region) Nucleus Impulse direction Node of Ranvier Nissl bodies Axon Axon hillock Schwann cell terminals (b) Neurilemma (one inter- (secretory node) Terminal region) branches Nerve Cell Body (Perikaryon or Soma) Contains the nucleus and a nucleolus Is the major biosynthetic center Is the focal point for the outgrowth of neuronal processes Has no centrioles (hence its amitotic nature) Has well-developed Nissl bodies (rough ER) Contains an axon hillock – cone-shaped area from which axons arise (Collin College, n.d.). 31 Processes Arm like extensions from the cell body There are two types of processes: axons and dendrites Myelinated axons are called tracts in the CNS and nerves in the PNS (Collin College, n.d.). 32 Dendrites: Structure Short, tapering processes They are the receptive, or input, regions of the neuron Convey messages towards the cell body Electrical signals are conveyed as graded potentials (not action potentials) (Collin College, n.d.). 33 Dendrites Cell body (receptive regions) (biosynthetic center and receptive region) Nucleolus Axon (impulse generating and conducting region) Nucleus Impulse direction Node of Ranvier Nissl bodies Axon Axon hillock Schwann cell terminals (b) Neurilemma (one inter- (secretory node) Terminal region) branches Figure 11.4b Axons: Structure Slender processes of uniform diameter arising from the hillock Vary from very short (almost absent) to perhaps 4 feet in length Usually there is only one unbranched axon per neuron Axon collaterals Axonal terminal or synaptic knobs (Collin College, n.d.). 35 Axons: Function Generate and transmit action potentials Secrete neurotransmitters from the axonal terminals Movement along axons occurs in two ways Anterograde — toward the axon terminal Retrograde — toward the cell body (Collin College, n.d.). 36 Myelin Sheath Whitish, fatty (protein-lipoid), segmented sheath around most long axons It functions to: Protect the axon Electrically insulate fibers from one another Increase the speed of nerve impulse transmission (Collin College, n.d.). 37 Myelin Sheath and Neurilemma: Formation Formed by Schwann cells in the PNS A Schwann cell: Envelopes an axon Encloses the axon with its plasma membrane Has concentric layers of membrane that make up the myelin sheath (Collin College, n.d.). 38 Myelin Sheath and Neurilemma Formation (Collin College, n.d.). 39 Nodes of Ranvier (Neurofibral Nodes) Gaps in the myelin sheath between adjacent Schwann cells They are the sites where axon collaterals can emerge (Collin College, n.d.). 40 Unmyelinated Axons A Schwann cell surrounds nerve fibers but coiling does not take place Schwann cells partially enclose 15 or more axons Conduct nerve impulse slowly (Collin College, n.d.). 41 Axons of the CNS Both myelinated and unmyelinated fibers are present Myelin sheaths are formed by oligodendrocytes Nodes of Ranvier are widely spaced (Collin College, n.d.). 42 Regions of the Brain and Spinal Cord White matter – dense collections of myelinated fibers Gray matter – mostly soma, dendrites, glial cells and unmyelinated fibers (Collin College, n.d.). 43 44 Structural Classification of Neurons Three types: 1. Multipolar—1 axon and several dendrites Most abundant Major neurons in CNS 2. Bipolar—1 axon and 1 dendrite Rare, e.g., retinal neurons (Meeking, 2010). Structural Classification of Neurons 3. Unipolar (pseudounipolar)—single, short process that then branches: Peripheral process—more distal branch, often associated with a sensory receptor (Meeking, 2010). Found chiefly in the PNS Comparison of Structural Classes of Neurons (Collin College, n.d.). 47 Comparison of Structural Classes of Neurons (Collin College, n.d.). 48 Neuron Classification Functional: Sensory (afferent) — transmit impulses toward the CNS Motor (efferent) — carry impulses toward the body surface Interneurons (association neurons) — any neurons between a sensory and a motor neuron (Collin College, n.d.). 49 Neurophysiology Neurons are highly irritable Action potentials, or nerve impulses, are: Electrical impulses carried along the length of axons Always the same regardless of stimulus (Collin College, n.d.). 50 Electrical Current and the Body Reflects the flow of ions rather than electrons There is a potential on either side of membranes when: The number of ions is different across the membrane The membrane provides a resistance to ion flow (Collin College, n.d.). 51 Role of Ion Channels Types of plasma membrane ion channels: Nongated, or leakage channels – always open Chemically gated channels – open with binding of a specific neurotransmitter (Collin College, n.d.). 52 Role of Ion Channels Voltage-gated channels – open and close in response to membrane potential. 2 gates Mechanically gated channels – open and close in response to physical deformation of receptors (Collin College, n.d.). 53 Gated Channels 54 (Collin College, n.d.). Electrochemical Gradient Ions flow along their chemical gradient when they move from an area of high concentration to an area of low concentration Ions flow along their electrical gradient when they move toward an area of opposite charge Electrochemical gradient – the electrical and chemical gradients taken together (Collin College, n.d.). 55 Resting membrane potential Equals the difference in charge b/t inside and outside of the membrane Polarized (unequal) across the membrane -40mV to -90 mV in different types of neurons More different if considering all types of cells (-) sign indicates the INSIDE of the neuron is negatively charged in relation to the outside (Hartford Community College, n.d.). Resting Membrane Potential (Vr) The potential difference (–70 mV) across the membrane of a resting neuron It is generated by different concentrations of Na+, K+, Cl, and protein anions (A) (Collin College, n.d.). 57 Resting Membrane Potential (Vr) Ionic differences are the consequence of: Differential permeability of the neurilemma to Na+ and K+ Operation of the sodium-potassium pump (Collin College, n.d.). 58 Remember… Inside the cell= less sodium & more potassium Outside the cell sodium is balanced by chloride ions Inside the cell (-) charged proteins help balance out the potassium Potassium diffuses out of cell easier than sodium diffuses in therefore inside is slightly more (-) which results in an electrical gradient that produces the resting membrane potential (Hartford Community College, n.d.). Remember… Sodium-potassium pump exports 3 sodium out & brings 2 potassium into the cell to maintain diffusion gradients for potassium & sodium Requires ATP…1 ATP for each 2K/3Na exchange (Collin College, n.d.). 60 Nervous Tissue Fundamentals P ANervous of the RT B System and Nervous Tissue 61 Membrane Potentials: Signals Used to integrate, send, and receive information Membrane potential changes are produced by: Changes in membrane permeability to ions Alterations of ion concentrations across the membrane Types of signals – graded potentials and action potentials (Collin College, n.d.). 62 Changes in Membrane Potential Changes are caused by three events Depolarization – the inside of the membrane becomes less negative Repolarization – the membrane returns to its resting membrane potential Hyperpolarization – the inside of the membrane becomes more negative than the resting potential (Collin College, n.d.). 63 Depolarization, Repolarization and Hyperpolarization 64 (Collin College, n.d.). Graded Potentials Short-lived, local changes in membrane potential Decrease in intensity with distance Magnitude varies directly with the strength of the stimulus Depolarization or hyperpolarization Sufficiently strong graded potentials can initiate action potentials (Collin College, n.d.). 65 Graded Potentials (Collin College, n.d.). 66 Figure 11.10 Graded Potentials Voltage changes are decremental Current is quickly dissipated due to the leaky plasma membrane Only travel over short distances (Collin College, n.d.). 67 Action Potentials (APs) A brief reversal of membrane potential Action potentials are only generated by muscle cells and neurons They do not decrease in strength over distance They are the principal means of neural communication An action potential in the axon of a neuron is a nerve impulse (Collin College, n.d.). 68 Action Potential: Resting State Na+ and K+ channels are closed Leakage accounts for small movements of Na+ and K+ Each Na+ channel has two voltage-regulated gates – Activation gates – closed in the resting state – Inactivation gates – open in the resting state (Hartford Community College, n.d.). Action Potential: Depolarization Phase Na+ permeability increases; membrane potential reverses Na+ gates are opened; K+ gates are closed Threshold – a critical level of depolarization (-55 to -50 mV) At threshold, depolarization becomes self-generating (Hartford Community College, n.d.). Action Potential: Repolarization Phase Sodium inactivation gates close Membrane permeability to Na+ declines to resting levels As sodium gates close, voltage-sensitive K+ gates open K+ exits the cell and internal negativity of the resting neuron is restored (Hartford Community College, n.d.). Action Potential: Hyperpolarization Potassium gates remain open, causing an excessive efflux of K+ This efflux causes hyperpolarization of the membrane (undershoot) The neuron is insensitive to stimulus and depolarization during this time (Hartford Community College, n.d.). Action Potential: Role of the Sodium-Potassium Pump Repolarization Restores the resting electrical conditions of the neuron Does not restore the resting ionic conditions Ionic redistribution back to resting conditions is restored by the sodium- potassium pump (Collin College, n.d.). 73 Phases of the Action Potential 1 – resting state 2 – depolarization phase 3 – repolarization phase 4 –hyperpolarization (Collin College, n.d.). 74 Action Potential 75 (Collin College, n.d.). Propagation of an Action Potential along an Unmyelinated Axon (Collin College, n.d.). 76 Propagation of an action potential Continuous propagation Unmyelinated axon Saltatory propagation Myelinated axon (Collin College, n.d.). 77 Propagation of an Action Potential (Time = 0ms) Na+ influx causes a patch of the axonal membrane to depolarize AP’s are initiated and are conducted away from that point towards the axon’s terminals…kind of like a domino effect Positive ions in the axoplasm move toward the polarized (negative) portion of the membrane The repolarization wave chases the depolarization wave down the length of the axon (Hartford Community College, n.d.). Propagation of an Action Potential (Time = 2ms) A local current is created that depolarizes the adjacent membrane in a forward direction Local voltage-gated Na channels are opened The action potential moves away from the stimulus (Hartford Community College, n.d.). Propagation of an Action Potential (Time = 4ms) The action potential moves away from the stimulus Where sodium gates are closing, potassium gates are open and create a current flow (Hartford Community College, n.d.). Propagation of an Action Potential (Time = 4ms)- continuous propagation Repolarization Hyperpolarization Sluggish K channels are kept opened Resting membrane potential is restored in this region (Collin College, n.d.). 81 Saltatory Conduction Current passes through a myelinated axon only at the nodes of Ranvier Voltage-gated Na+ channels are concentrated at these nodes Action potentials are triggered only at the nodes and jump from one node to the next Much faster than conduction along unmyelinated axons (Collin College, n.d.). 82 Saltatory Conduction (Collin College, n.d.). 83 Types of stimuli Threshold stimulus Stimulus strong enough to bring the membrane potential to a threshold voltage causing an action potential Subthreshold stimulus weak stimuli that cause depolarization (graded potentials) but not action potentials (Collin College, n.d.). 84 Coding for Stimulus Intensity Action potential are All-or-none phenomenon Strong stimuli can generate an action potential more often than weaker stimuli The CNS determines stimulus intensity by the frequency of impulse transmission (Collin College, n.d.). 85 Stimulus Strength and AP Frequency (Collin College, n.d.). 86 Absolute Refractory Period Time from the opening of the Na+ activation gates until the closing of inactivation gates The absolute refractory period: Prevents the neuron from generating an action potential Ensures that each action potential is separate Enforces one-way transmission of nerve impulses (Collin College, n.d.). 87 Relative Refractory Period The interval following the absolute refractory period when: Sodium gates are closed Potassium gates are open Repolarization is occurring Stimulus stronger than the original one cause a new action potential (Collin College, n.d.). 88 Absolute and Relative Refractory Periods 89 (Collin College, n.d.). 90 Nerve impulses are transmitted via branches called synapses. The synapses are connectors… hooking dendrites and axons from one neuron to another. The number of synapses influences transmission. That number can decrease with disease, lack of stimulation, drug use, etc. (Nebraska Department of Education, 2017) The synapse The means by which adjacent neurons communicate Most occur b/t axon of one neuron & the dendrites of another (axodendritic) or b/t the axon of one neuron & the cell body of another (axosomatic) Presynaptic neuron is information sender while the postsynaptic neuron is the information receiver Neurons may have from 1000 to 10000 axonal terminals making synapses (Hartford Community College, n.d.). The brain and spinal cord receive impulses, process the information, and respond with the appropriate action. Gray matter of the brain and spinal cord consists of unsheathed nerve fibers (cannot be regenerated if damaged) in the cortex or surface layer. The white matter makes up the internal structure, and consists of myelinated nerve fibers. Electrical Synapses Are less common than chemical synapses Correspond to gap junctions found in other cell types Very fast propagation of action potentials Are important in the CNS in: Arousal from sleep Mental attention Emotions and memory Ion and water homeostasis (Hartford Community College, n.d.). Chemical Synapses Specialized for the release and reception of neurotransmitters Typically composed of: Presynaptic neuron Contains synaptic vesicles Postsynaptic neuron The receptors are located typically on dendrites and soma (Collin College, n.d.). 95 Chemical Synapses Synaptic Cleft Fluid-filled space separating the presynaptic and postsynaptic neurons Transmission across the synaptic cleft: Is a chemical event (as opposed to an electrical one) Ensures unidirectional communication between neurons (Collin College, n.d.). 96 Synaptic Cleft: Information Transfer Nerve impulses reach the axonal terminal of the presynaptic neuron and open Ca2+ channels Neurotransmitter is released into the synaptic cleft via exocytosis (Collin College, n.d.). 97 Synaptic Cleft: Information Transfer Neurotransmitter crosses the synaptic cleft and binds to receptors on the postsynaptic neuron Postsynaptic membrane permeability changes, causing an excitatory or inhibitory effect (Collin College, n.d.). 98 Synaptic Cleft: Information Ac tent Transfer Neurotransmitter Ca2+ Na+ po tio ial Axon terminal of Receptor n 1 presynaptic neuron Postsynaptic Mitochondrion membrane Postsynaptic Axon of membrane presynaptic neuron Ion channel open Synaptic vesicles 5 containing neurotransmitter molecules Degraded 2 neurotransmitter Synaptic cleft 3 4 Ion channel closed Ion channel Ion channel (open) (closed) (Collin College, n.d.). 99 Termination of Neurotransmitter Effects Neurotransmitter bound to a postsynaptic neuron: Produces a continuous postsynaptic effect Blocksreception of additional “messages” Must be removed from its receptor (Collin College, n.d.). 100 Postsynaptic Potentials Neurotransmitter receptors mediate changes in membrane potential according to: The amount of neurotransmitter released The amount of time the neurotransmitter is bound to receptors The two types of postsynaptic potentials are: EPSP – excitatory postsynaptic potentials IPSP – inhibitory postsynaptic potentials (Hartford Community College, n.d.). EPSP’s Excitatory synapses cause depolarization of the postsynaptic membrane(Hartford Community College, n.d.). IPSP’s Inhibitory synapses reduce a postsynaptic neuron’s ability to generate an AP Changes membrane permeability to ions making the inner face of the membrane more (-) making it less likely to ‘fire’ (Hartford Community College, n.d.). Terminology for quiz Neuron = nerve cell Neuroglia = supporting cell Nerve fiber = long axon Nerve = collection of nerve fibers (axons) in PNS Tract = collections of nerve fibers (axons) in CNS Nucleus = cluster of cell bodies in CNS Ganglia = cluster of cell bodies in PNS New: Unilateral: on one side Ipsilateral: on the same side Contralateral: on the opposite side Remember also: CNS vs PNS Input: sensory: afferent: to brain Output: motor : efferent: from brain (Los Angeles Valley College, n.d.). Examine Yourself Which one of the following is related to the tract? Neurons outside the CNS. Neurons inside the CNS. Nerve fibers within the CNS. Nerve fibers outside the CNS. Which structure is concerning with formation of CSF ? The arachnoid villi. The choroid plexus. The subdural space. The dural venous sinus. The peripheral nervous system involves : The spinal ganglia. The spinal cord. The brain. The tracts. The lateral ventricle lies in : The cerebrum. The diencephalon. The midbrain. The cerebellum. Reference Collin College. (n.d.). Nervous Tissue. Retrieved from http://iws2.collin.edu/efanini/BIOL %202401/Lecture%20Note%20PPT/Ne rvous%20Tissue.pptx 106 Reference Cont’d Harford Community College. (n.d.). Neural Tissue. Retrieved from http://ww2.harford.edu/faculty/SSchaeff er/BIO%20203/Ch%2012-Neural %20Tissue.ppt Ireland, K. (2004). Neural tissue. Retrieved from http://websupport1.citytech.cuny.edu/F aculty/ibarjis/Teaching/Medical- Pathology/Lecture%2014.ppt 107 Reference Cont’d Los Angeles Valley College. (n.d.). Fundamentals of the Nervous System. Retrieved from http://www.lavc.edu/instructor/watson_k/docs/Lecture %2012%20-%20Fundamentals%20of%20the%20Nervous %20System.ppt Meeking, J. (2010). Fundamentals of the Nervous System and Nervous Tissue: Part A. Retrieved from http://faculty.sgc.edu/sundram/2210/ch_11_lecture_outline_ a.ppt Nebraska Department of Education. 2017. Retrieved from:https://www.education.ne.gov/wp-content/uploads/20 17/07/179-Anatomy-Nervous-System.ppt 108 The Washington Township Public School. Retrieved from: https://www.wtps.org/cms/lib8/NJ01912980/C entricity/Domain/880/Essential%20Marieb.ppt (Collin College, n.d.). 109