BIO3303 Nervous System 1 PDF
Document Details
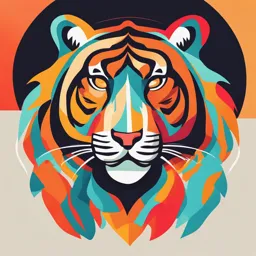
Uploaded by SafeMahoganyObsidian
Tags
Summary
This document provides an overview of the nervous system, including its structure, function, and associated processes like signal transmission, action potential generation, and neuronal networks. It covers key concepts and details about nervous system components, types of neurons, and related processes.
Full Transcript
BIO3303 – Nervous System 1 Control systems I – Nervous system (Chpts. 5 and 8) Control systems II - Endocrinology (Chpt. 4) Sensory physiology (Chpt. 7) Muscles, locomotion and animal energetics (Chpts. 6, 12 and 14) Control Systems I : Nervous System Objectives (1 of 3) By the end of this lecture,...
BIO3303 – Nervous System 1 Control systems I – Nervous system (Chpts. 5 and 8) Control systems II - Endocrinology (Chpt. 4) Sensory physiology (Chpt. 7) Muscles, locomotion and animal energetics (Chpts. 6, 12 and 14) Control Systems I : Nervous System Objectives (1 of 3) By the end of this lecture, you should be able to… Contrast and compare the nervous and endocrine systems Explain how neuroendocrine cells span the divide between the nervous and endocrine systems List the structural and functional units of the nervous system Distinguish among membrane, action and synaptic potentials, explaining the physiological basis of each Understand the difference between an electrical and chemical synapse (gap junction, ionotropic, metabotropic) Describe a reflex arc Understand the process of habituation and sensitization Overview of cell signaling Fig. 4.2 Comparisons between Nervous and Endocrine Systems Endocrine system Endocrine cells = all glands that secrete hormones Release chemical messengers (hormones) into circulatory system Carried to target tissues Nervous system Electrical signal travel in neuron Release chemicals (neurotransmitters) into space between neuron and target cell Fig. 11.3 Campbell & Reece 2002 Cells Distinguished by Communication Strategy Neurons: electrical signal releases neurotransmitters into synapse to communicate directly with target (postsynaptic) cell Neurosecretory cells: release neurohormones into circulation in response to electrical signals Interface between systems Endocrine cells: release hormones into circulation Fig. 9.8, Randall et al. 2002 Nervous and endocrine systems interact extensively Fig. 4.30 Differences between endocrine and nervous systems Table 4.1 Neuron Structure and Function Soma (cell body) Dendrites typically receive incoming signal result in change in membrane potential Axon hillock initiate action potentials Travels along axon to axon terminals and triggers neurotransmitter release Axon myelinated or unmyelinated Fig. 4.2/5.2 Neuronal Diversity * * * * Fig. 4.18/5.23 Ion concentration gradients and permeability establish membrane potentials Resting membrane potential ~-70mV (inside relative to outside) Made possible by imbalance of Na and K concentrations on either side of the membrane -> concentration gradient Recall phospholipid membranes permeable to hydrophobic molecules, but impermeable to hydrophilic molecules (ie, ions) Concentration gradient maintenance helped by Na+/K+ ATPase pumps to compensate for “leaks” – K+ in, Na+ out Ion concentration gradients and permeability establish membrane potentials Resting membrane potential ~-70mV (inside relative to outside) Example - consider starting concentrations: Inside cell: 100mM KCl, 10mM NaCl Outside cell: 10mM KCl, 100mM NaCl Fig. 2.50/5.3 Ion Channel Types Open under different conditions Ligand-gated channels open when ligands (neurotransmitter) bind Voltage-gated (VG) channels open when there is a specific change in membrane potential (open at specific stages of an action potential) Fig. 2.49 4.21 and 5.41 Signal transmission A signal travels down the axon to the terminals to communicate with other neurons, muscles or other target cells The transmitting neuron is presynaptic and the receiving neuron is postsynaptic Two types of membrane potentials: graded potentials, action potentials Presynaptic neuron Postsynaptic neuron signal Fig. 4.27/5.33 Graded Potentials Activated by ligand- gated Na+ channel, cause changes in membrane potential ‘Synaptic potential’ Spatially restricted response Conduction with decrement Fig. 4.6/5.8 Electrical Signals Graded changes in membrane potential occur in dendrites and cell body Are spatially and temporally summated If combined depolarization exceeds threshold, an action potential is generated Spatial summation can also Fig. 4.8/5.10 prevent generation of APs (excitatory + inhibitory potential) Electrical Signalling When net change in membrane potential at the axon hillock reaches or exceeds threshold (suprathreshold graded potential) an action potential is triggered and travels down the axon (non-graded, all-ornone signal transmission) Fig. 11.11, Hill et al. 2004 Fig 4.7/5.12 Action Potentials (“Spikes”) Stages of APs Membrane potential depolarized past threshold potential (- 55mV) Rapid depolarization until 30 mV Membrane potential repolarizes Membrane potential undershoots resting potential Resting potential is restored Fig. 4.10/5.12 Depolarization and Na+ Channels VG-channels shape action potential Two voltage regulated gates: 1. Activation gate Opens at threshold 2. Inactivation gate Closes at +30mV Also VG K+ channels triggered at threshold (-55 mV) but open more slowly than Na+ channels Fig. 4.11/5.14 Conduction of APs Na+ comes in Induces local depolarization opens Na+ channels close by Na+ comes in, etc. APs travel down the axon only in one direction (absolute refractory period) http://www.youtube.com/watch?v=pbg5E9GCNVE&feature=related Fig. 5.17 Action Potentials Absolute and relative refractory period: limit # of APs along axon, prevents backward transmission of APs. Absolute refractory period – the inactivation gate of the Na ion channel closes at at 30mV, and remains closed until resting potential is reestablished – impossible to generate AP at an area where an AP has just occurred Relative refractory period – during hyperpolarization – possible, but harder to generate AP Fig. 4.10/5.12 Propagation of action potential video: http://www.youtube.com/watch?v=pbg5E9GCNVE&feature=related Evolution is cool… Takifugu rubripes: Japanese puffer fish. Contain tetrodotoxin TTX TTX blocks voltage-gated ion channels Prevents action potential generation 1 mg is deadly Puffer fish are safe due to a single mutation in their Na+ voltage gated ion channels. Signal Transmission Myelin sheath: a (mostly) vertebrate feature; a highly compacted, fatty substance PNS - Schwann cells wrap axons CNS – oligodendrocytes (OLs) wrap axons Schwann cells/OLs produce myelin - acts as insulation, preserving electrical potential – increases resistance, reduces capacitance – like insulation on an electrical wire Fig. 5.18 Signal Transmission Increases speed and efficiency of electrical transmission Nodes of Ranvier = lots of Voltage-gated Na+ channels Saltatory conduction: APs jump from node to node along the axon Unmyelinated vs myelinated propagation of action potential video: http://www.youtube.com/watch?v=DJe3_3XsBOg Electrical Signals Table 5.1 Synaptic Transmission Cell to cell communication 1. Electrical 2. Chemical a. Ionotrophic b. metabotrophic Fig. 4.26/5.32 Electrical synapses gap junctions composed of connexin proteins connect presynaptic and postsynaptic cell membranes Pore allows fast chemical and ionic transmission Gap junction proteins Fig. 3.2/4.3 Synaptic Transmission Chemical synapses Neurotransmitter binds postsynaptic receptor: A. Ionotropic receptors Are ligand-gated channels Rapid changes in postsynaptic membrane potential B. Metabotropic receptors signaling cascade opens ion channels Activates intracellular transduction pathway Slower changes in postsynaptic membrane potential Can also modify proteins and gene expression (eg. learning, memory) Fig. 4.28/5.34 Simple Neural Networks Sensory information detected by receptor → Signal transmitted from sensory neuron → efferent neuron → effector organ (response) Fig. 7.23/8.24 Reflex Arc Simple neural circuits that do not involve the conscious centers of the brain involuntary Simple (2 neurons) or complex (many neurons) Autonomic (e.g. Reflex control of blood pressure) Somatic (e.g. Knee-jerk reflex) Neural Networks Ancestral form: receptor cell directly innervates an effector cell (little processing, no CNS) Monosynaptic: sensory neuron synapses with efferent neuron e.g. knee-jerk reflex (http://www.youtube.com/watch?v=HfuhVWK8C0U) Polysynaptic: at least one interneuron between sensory and efferent neuron (increases processing capacity) Ancestral form Monosynaptic Polysynaptic Sensory neuron Fig. 8.2, Randall et al. 2002 Reflex Arc Example: Cockroach’s jump (startle response) Sensory neurons (afferent) in filiform hairs detect wind and sound waves (stimuli) Relays signal to giant interneuron in the terminal ganglion Signals leg motor neuron (efferent) to cause contraction of leg muscles Polysynaptic Fig. 11.3, Hill et al. 2nd ed. Reflex Arc Example: Cockroach’s jump (startle response) https://www.youtube.com/watch?v=t8DeAh-2nik Neural Networks Many combinations possible Convergence: many afferent neurons synapse with one efferent neuron Divergence: one afferent neuron synapses with many efferent neurons. Allows a single signal to control many independent processes, and it is a way to amplify the signal. Fig. 7.24/8.25 Aplysia Gill-Withdrawal Reflex Habituation simple kind of learning a decline in the tendency to respond to a stimulus due to repeated exposure partial inactivation of voltagegated channels in axon terminal of sensory neuron Less transmitters are released Sea slug http://www.uoregon.edu/~joet/aplysia.jpg Fig. 7.29/8.31 Sensitization of Gill-Withdrawal Reflex Sensitization: an increase in the response to a gentle stimulus after exposure to a strong stimulus. Electric shock to tail sensitizes response to siphon stimulation Occurs via facilitating interneurons Fig. 7.29 Fig. 7.30/8.32 Summary of Key Points Compare the nervous and endocrine systems Describe the structure of neuron and how it relates to function (Dendrites: input, Axon hillock: AP generation, Terminals: Nt release) Describe in detail how graded and action potential are generated, and how they relate to each other Understand the difference between an electrical and chemical synapse (gap junction, ionotropic, metabotropic) Distinguish among membrane, action and synaptic potentials Describe simple neural networks (e.g. Reflex arcs) Understand the process of habituation and sensitization